Lecture 2 Excitable Cells PDF
Document Details
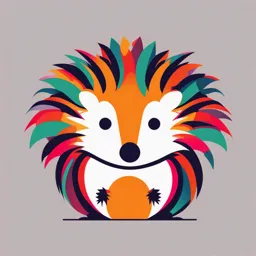
Uploaded by GloriousJasper3189
University of Malta
2016
Massimo Pierucci
Tags
Summary
These lecture notes cover excitable cells. They discuss the membrane potential, ion channels, and the action potential. 
Full Transcript
PSY1617 BILOGICAL PSYCHOLOGY 1 Excitable Cells Dr Massimo Pierucci University of Malta Room 125, Biomedical Building massimo.pieru...
PSY1617 BILOGICAL PSYCHOLOGY 1 Excitable Cells Dr Massimo Pierucci University of Malta Room 125, Biomedical Building [email protected] © Cengage Learning 2016 Summary of the Lecture Basic concepts: the cell membrane and the transport of substances through it; Protein Carriers and Active Transport. Resting Membrane Potential: an energy store Ion Channels Electrochemical Gradients across the membrane Na+-K+ ATPase Pump Action Potential: role of voltage-activated Ion Channels Graded Potentials: integration of neuronal imputs Excitable cells Cells that are capable of changing their membrane potential on stimulation in an explosive and reversible manner and generate ACTION POTENTIALS © Cengage Learning 2016 Excitable cells An action potential (or nerve impulse) is a transient alteration of the transmembrane voltage (or membrane potential) across an excitable membrane in an excitable cell (such as a neuron or myocyte) generated by the activity of voltage-gated ion channels embedded in the membrane. The best known action potentials are pulse-like waves of voltage that travel along the axons of neurons. © Cengage Learning 2016 Methods for Recording Activity of a Neuron © Cengage Learning 2016 MEMBRANE POTENTIAL © Cengage Learning 2016 The membrane potential depends on unequal distributions of ions across the membrane Body is electrically neutral, solutes include both anions (-) and cations (+). Na+,Ca++ extracellular fluids (ECF) K+ intracellular fluids (ICF) Cl- extracellular fluids (ECF) Protein anions- intracellular fluids (ICF) Phosphate anions- intracellular fluids (ICF) However, ions are not distributed evenly between the ECF and ICF Membrane Potential © Cengage Learning 2016 MEMBRANE POTENTIAL © Cengage Learning 2016 Resting membrane Potential Em=-70 mV Distribution of the major ions across a membrane at rest Element Ion Extracellular Intracellular Ratio Sodium Na+ 145 14 10:1 Potassium K+ 4 150 1:30 Chloride Cl- 120 5 24:1 Calcium Ca2+ 2 10-4 2 x 104:1 Although intracellular Ca2+ content is about 2 mM, most of this is bound or sequestered in intracellular organelles (mitochondria and sarcoplasmic reticulum). 9 © Cengage Learning 2016 The Membrane of a Neuron © Cengage Learning 2016 The Cell Membrane and its Permeability © Cengage Learning 2016 Transport Proteins move most ions and large/non-lipophilic molecules across the plasma membrane of a cell © Cengage Learning 2016 GATED MEMBRANE CHANNELS 13 © Cengage Learning 2016 Transport Proteins mediate both Passive and Active Transports across the cell membrane © Cengage Learning 2016 Pore channels are selectively permeable to different ions © Cengage Learning 2016 MEMBRANE POTENTIAL (DIFFERENCE) ECF is in equilibrium with the ICF ECF is NOT in equilibrium with the ICF Because of the uneven distribution of ions between ICF and ECF, the 2 compartments are not in electrical equilibrium, thus generating an electrical potential difference between the two, the so called membrane electrical potential. The membrane is an insulator. © Cengage Learning 2016 Impermeable membrane © Cengage Learning 2016 Selective permeable membrane An electrical gradient is created, this will avoid all K+ ions to leak out of the cell! Electro-chemical Gradient © Cengage Learning 2016 © Cengage Learning 2016 Nernst Equation For any given concentration gradient of a single ion, the equilibrium potential can be calculated using the Nernst Equation: © Cengage Learning 2016 Equilibrium Potential for the different IONS The main assumption, when calculating these values using the Nernst equation, is that the membrane is freely permeable to each single ion, i.e. the CONDUNCTANCE is infinite! © Cengage Learning 2016 NERNST EQUATIONS Em = -90 mV 22 © Cengage Learning 2016 RESTING MEMBRANE POTENTIAL DIFFERENCE © Cengage Learning 2016 IN REAL LIFE... All animal (and plant) cells show a resting membrane potential difference; Cells ARE NOT permeable to only one ion, but they show different permeabilities to any of them and they can change over time; The resting memebrane potential difference results from the different ions involved and the membrane permeability to each of them; In real life, to calculate the equilibrium potential we use an equation related to the Nernst equation, called the Goldman-Hodgkin-Katz (GHK) equation: © Cengage Learning 2016 Dissipation of Na/K ionic gradients is prevented by the Na/K pump Continuous flux is counterbalanced by the Na/K pump © Cengage Learning 2016 Electrogenic properties of the Na/K pump is important for the generation of Action Potentials Plays a key role in setting and maintain a resting membrane potential in neurons by keeping Sodium and Potassium concentration gradients. Ionic gradients across the cell membrane of a neuron are needed for the generation of Action Potentials. During period of intense neuronal activity, increased influx of Sodium leads to an increased activity of the pump. © Cengage Learning 2016 The Sodium-Potassium ATPase is an electrogenic pump © Cengage Learning 2016 The Sodium-Potassium ATPase is an electrogenic pump Na+ K+ Na+ K+ Na+ Na+ © Cengage Learning 2016 Sodium and Potassium Gradients for a Resting Membrane: role of different ions permeability © Cengage Learning 2016 ELECTRICAL AND CHEMICAL DRIVING FORCES K+ set the membrane potential Ca++ = 2 Ca++ = 10-4 Em=-70mV Membrane potential © Cengage Learning 2016 The membrane potential depends mostly on K+ Real Life Cell In normal conditions, most of the cells are 40 times more permeable to K+ As a result, the equilibrium potential of the cell is closer to the one of K+ (-70mV vs -90mV) The slightly more depolarized state depends on a smaller leak of Na+ inside the cell If the permeability to Na+ becomes much bigger than the K+, the equilibrium potential will move towards....? Action potential of a neuron © Cengage Learning 2016 ION PUMPS MANTAIN ION GRADIENT 32 © Cengage Learning 2016 33 © Cengage Learning 2016 34 © Cengage Learning 2016 35 © Cengage Learning 2016 36 © Cengage Learning 2016 Changes in resting membrane potential: definitions © Cengage Learning 2016 The Resting Potential of the Neuron, Part 1 Messages in a neuron develop from disturbances of the resting potential At rest, the membrane maintains an electrical gradient known as polarization – A difference in the electrical charge inside and outside of the cell © Cengage Learning 2016 The Resting Potential of the Neuron, Part 2 The inside of the membrane is slightly negative with respect to the outside (approximately -70 millivolts) The resting potential of a neuron refers to the state of the neuron prior to the sending of a nerve impulse © Cengage Learning 2016 Forces Acting on Sodium and Potassium Ions The membrane is selectively permeable, allowing some chemicals to pass more freely than others Sodium, potassium, calcium, and chloride pass through channels in the membrane When the membrane is at rest: – Sodium channels are closed – Potassium channels are partially closed allowing the slow passage of potassium © Cengage Learning 2016 Ion Channels The sodium-potassium pump is a protein complex – Continually pumps three sodium ions out of the cells while drawing two potassium ions into the cell – Helps to maintain the electrical gradient © Cengage Learning 2016 Electrical and Concentration Gradients The electrical gradient and the concentration gradient – the difference in distributions of ions – work to pull sodium ions into the cell The electrical gradient tends to pull potassium ions into the cells – However, they slowly leak out, carrying a positive charge with them © Cengage Learning 2016 Resting Membrane Potential (RMP) in summary An electrical Membrane Potential exists across the plasma membrane; it is negative inside and positive outside (0V) The RMP depends on an unequal distribution of Na+, K+, Cl- ions across the membrane The unequal distribution is the result of an equilibrium between an electrical gradient and a chemical gradient, ELECTROCHEMICAL GRADIENT This equilibrium depends on the permeability of the membrane to the different ions At equilibrium, there is a constant flow of ions in/out of the cells with no net changes in the electric charge across the membrane. This equilibrium is maintained over time through the activity of the Sodium/Potassium Pump. At Equilibrium, the ions with the highest permeability set the membrane potential The Action Potential © Cengage Learning 2016 1.2 The Nerve Impulse The electrical message that is transmitted down the axon of a neuron – Does not travel directly down the axon, but is regenerated at points along the axon so that it is not weakened The speed of nerve impulses ranges from less than 1 meter/second to 100 meters/second – A touch on the shoulder reaches the brain more quickly than a touch on the foot © Cengage Learning 2016 The Nerve Impulse The brain is not set up to register small differences in the time of arrival of touch messages However, in vision, movements must be detected as accurately as possible The properties of impulse control are well adapted to the exact needs for information transfer in the nervous system © Cengage Learning 2016 Action potentials 47 © Cengage Learning 2016 A stimulus is needed to start an AP The resting potential remains stable until the neuron is stimulated – Hyperpolarization: increasing the polarization or the difference between the electrical charge of two places – Depolarization: decreasing the polarization towards zero – The threshold of excitation: a level above which any stimulation produces a massive depolarization © Cengage Learning 2016 The AP Threshold A rapid depolarization of the neuron The action potential threshold varies from one neuron to another, but is consistent for each neuron Stimulation of the neuron past the threshold of excitation triggers a nerve impulse or action potential © Cengage Learning 2016 Resting and Action Potentials © Cengage Learning 2016 Action Potential Phases © Cengage Learning 2016 Voltage-Activated Channels Membrane channels whose permeability depends upon the voltage difference across the membrane – Sodium and potassium channels When sodium channels are opened, positively charged sodium ions rush in and a subsequent nerve impulse occurs © Cengage Learning 2016 The Movement of Sodium and Potassium Ions During an Action Potential Refractory Periods After an action potential, a neuron has a refractory period during which time the neuron resists the production of another action potential – The absolute refractory period: the first part of the period in which the membrane cannot produce an action potential – The relative refractory period: the second part, in which it takes a stronger than usual stimulus to trigger an action potential © Cengage Learning 2016 Refractory Periods © Cengage Learning 2016 Refractory Periods © Cengage Learning 2016 The Movement of Sodium and Potassium After an action potential occurs, sodium channels are quickly closed The neuron is returned to its resting state by the opening of potassium channels – Potassium ions flow out due to the concentration gradient and take with them their positive charge The sodium-potassium pump later restores the original distribution of ions © Cengage Learning 2016 Restoring the Sodium-Potassium Pump The process of restoring the sodium- potassium pump to its original distribution of ions takes time An unusually rapid series of action potentials can lead to a buildup of sodium within the axon – Can be toxic to a cell, but only in rare instances such as stroke and after the use of certain drugs © Cengage Learning 2016 Blocking Sodium Channels Local anesthetic drugs block sodium channels and therefore prevent action potentials from occurring – Example: Lidocaine, Novocaine and Xylocaine Tetrodotoxin (TTX): Na channels blocker Can lead to death due to respiratory failure: it causes loss of sensation, paralysis of voluntary and respiratory muscles. Tetraodontidae © Cengage Learning 2016 Puffer Fish The AP originates at and propagates from the Axon Hillock/Initial Segment Back-Propagation Forward-Propagation The axon hillock and initial segment have a much higher density of voltage-gated ion channels than is found in the rest of the cell body. © Cengage Learning 2016 The All-or-None Law, Part 1 Action potentials back-propagate into the cell body and dendrites – Involvement in synaptic plasticity, help in forming LTP (Long-Term potentiation) The all-or-none law – States that the amplitude and velocity of an action potential are independent of the intensity of the stimulus that initiated it – Action potentials are equal in intensity and speed within a given neuron © Cengage Learning 2016 The All-or-None Law, Part 2 Action potentials vary from one neuron to another in terms of amplitude, velocity, and shape Studies of mammalian axons show that there is much variation in the types of protein channels and therefore in the characteristics of the action potentials © Cengage Learning 2016 Propagation of an Action Potential, Part 1 In a motor neuron/interneurons, the action potential begins at the axon hillock (a swelling where the axon exits the soma) – sensory neurons: the trigger zone is immediately adjacent to the receptor, where the dendrites join the axon. Propagation of the action potential: the transmission of the action potential down the axon © Cengage Learning 2016 Refractory period: a 1 way direction Action potentials cannot sum Action potential can only travel in one direction Absolute Refractory period: no generation of AP Relative Refractory Period: strong graded potentials can generate an AP of smaller amplitude. © Cengage Learning 2016 AP propagation © Cengage Learning 2016 © Cengage Learning 2016 Propagation of an Action Potential, Part 2 © Cengage Learning 2016 The Myelin Sheath The myelin sheath of axons are interrupted by short unmyelinated sections called nodes of Ranvier – Myelin is an insulating material composed of fats and proteins – At each node of Ranvier, the action potential is regenerated by a chain of positively charged ions pushed along by the previous segment © Cengage Learning 2016 An Axon Surrounded by a Myelin Sheath © Cengage Learning 2016 Saltatory Conduction The “jumping” of the action potential from node to node – Provides rapid conduction of impulses – Conserves energy for the cell Multiple sclerosis: disease in which the myelin sheath is destroyed – Associated with poor muscle coordination and sometimes visual impairments © Cengage Learning 2016 AP propagation in myelinated axons © Cengage Learning 2016 Saltatory Conduction in a Myelinated Axon © Cengage Learning 2016 Local Neurons, Part 1 Have short axons, exchange information with only close neighbors, and do not produce action potentials When stimulated, produce graded potentials – membrane potentials that vary in magnitude and do not follow the all-or- none law Depolarize or hyperpolarize in proportion to the stimulation © Cengage Learning 2016 Local Neurons, Part 2 Difficult to study due to their small size Most of our knowledge has come from the study of large neurons Myth – Only 10 percent of neurons are active at any given moment Truth – You use all of your brain, even at times when you might not be using it very well © Cengage Learning 2016 Graded Potentials An action potential requires a stimulus above the threshold: role of Graded Potentials. © Cengage Learning 2016 The stimulus is provided by Graded Potentials Graded Potentials are depolarizations (+) or hyperpolarization (- ) occurring in dendrites or cell body. Graded because their amplitude (size) is proportional to the strength of the triggering event and the distance from their origin. Triggering stimulus could be the interaction between the neurotransmitter released by pre-synaptic neuron and its receptors on the post-synaptic neurons (Ligand-gated or GPCR). Opening of ion channels (Na+, K+, Cl-) These electric stimuli propagate across the cytoplasm like a wave, reducing their amplitude with distance. If strong enough, they can reach the trigger zone for AP, called Initial Segment and localized at the Axon Hillock; this area is reach in voltage-gated Na+ channels. If© Cengage the potential Learning 2016 reaches the threshold, an AP will be fired. Graded potentials reduces their amplitude with distance © Cengage Learning 2016 Graded Potentials amplitude depends on the strength of the stimulus © Cengage Learning 2016 Temporal summations occurs when 2 graded potentials from one pre-synaptic neuron occur close together in time. © Cengage Learning 2016 Spatial Summations © Cengage Learning 2016 © Cengage Learning 2016 SUMMARY Resting membrane potential Action Potential: role of Na+ and K+ in its generation Axon Hillock/Initial Segment is the triggering area for AP AP propagate in one direction and cannot summate: All-or-None Law Role of refractory periods Graded potentials results from receptors activation in the synapse Graded potentials represent inputs to the neuron that are integrated to eventually trigger and AP © Cengage Learning 2016 Optogenetics: changing organism’s behavior with light Using transgenic technique, light- activated channelrhodopsin-2 (ChR2, Chlamydomonas reinhardtii) are expressed in target neurons. Using blue light, these are activated and, in doing so, they open briefly to allow the passage of Na/K ions, resulting in the generations of depolarizing stimuli and generation of action potentials https://www.youtube.com/watch?v=RVJls79LaTo&feature=youtu.be https://www.youtube.com/watch?v=ChogEq7fBgE&feature=youtu.be Traditionally, dopamine is known to transmit reward signals (food, sex, etc.) in the brain and promote behaviors that lead to that reward again. What you may not know, however, is that the area of the brain that releases dopamine, the ventral midbrain, also receives signals of aversion (things we find unpleasant or even dangerous) from a far-off brain region called the lateral habenula. These avoidance signals promote behaviors that lead us to avoid unpleasant or dangerous things in the world. © Cengage Learning 2016 THANK YOU! © Cengage Learning 2016