Lecture 2: Electrical Network Theory, Current, and Voltage PDF
Document Details
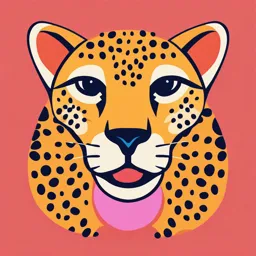
Uploaded by GratifyingTechnetium6978
كلية تكنولوجيا العلوم الصحية التطبيقية
Tags
Summary
This document is lecture notes on electrical network theory, detailing different types of circuits, the concept of voltage and current, and the use of different types of circuits.
Full Transcript
Introduction to electrical engineering: Lecture 2 Electrical Network Theory and Electricity Electrical Circuit An electric circuit is defined as a combination of interconnected networks that provide one or more closed paths for electric current. A circuit is an unbroke...
Introduction to electrical engineering: Lecture 2 Electrical Network Theory and Electricity Electrical Circuit An electric circuit is defined as a combination of interconnected networks that provide one or more closed paths for electric current. A circuit is an unbroken loop of conductive material that allows charge carriers to flow through continuously without beginning or end. If a circuit is “broken,” that means its conductive elements no longer form a complete path, and continuous charge flow cannot occur in it. The location of a break in a circuit is irrelevant to its inability to sustain continuous charge flow. Any break, anywhere in a circuit prevents the flow of charge carriers throughout the circuit. Figure 1: circuit A circuit with a complete path for electrical flow is called a closed circuit. A complete path must exist before electricity can flow through a circuit. A simple switch closes and opens an electrical circuit. If the circuit path is incomplete or broken, this is called an open circuit. a circuit element with a resistance approaching infinity. A short circuit has an unintended shorter pathway. a circuit element with resistance approaching zero. Figure 2: (a) closed circuit (b) open circuit (c) short circuit All electrical circuits require three elements. (1) A source voltage, that is, an electron pump usually a battery or power supply. [ Energy In] (2) A conductor to carry electrons from and to the voltage source pump). The conductor is often a wire. [Energy Transfer] (3) A load or resistance. A point where energy is extracted from the circuit in the form of heat, light, motion, etc. [Energy Out] 1 All functional circuits have a source and a load. The source generates power, while the load consumes it. The rest of the circuit is simply formatting the electricity to travel properly to the load. By definition, a source is a device delivering energy into a system, while a load is a device extracting energy from a system. Figure 3: an example of a complete circuit with source and a load and connecting wires. Electrical Network Analysis In electrical engineering and electronics, a network is a collection of interconnected components, connected together by wires. Network topology is a graphical representation of electric circuits. Network topology is the interconnection of its elements. That, plus the constraints on voltage and current imposed by the elements themselves, determines the performance of the network, described by the distribution of voltages and currents throughout the network. So, it is useful for analyzing complex electric circuits by converting them into network graphs. Electric network theory deals with two primitive quantities, which we will refer to as: 1. Potential (or voltage), and 2. Current. Current is the actual flow of charged carriers, while difference in potential is the force that causes that flow. Network analysis is the process of finding the voltages across, and the currents through, all network components. As we will see, potential is a single- valued function that may be uniquely defined over the nodes of a network. Current, on the other hand, flows through the branches of the network. An electric network is a combination of interconnected circuit elements and may not always provide a closed path for current. However, an electrical circuit includes one or more networks that create closed paths for electric current to flow. When these networks interconnect to complete one or more paths, an electric circuit is formed. An electric circuit is based on three concepts: nodes, branches, and loops. Nodes of Electric Circuit a node is a point at which two or more circuit elements are connected together. The point through which a circuit element is connected to the circuit is called node. Node is a junction point in the circuit. 2 + v1 − − v2 + i1 i2 v3 i3 Figure 4: This is a node NB:- If three is no element between two or more connected adjacent nodes, these nodes can be recombined as a single node. Figure 5: This is also a node Finally, the circuit can be redrawn as, Figure 6: This is redrawn of a node in figure 5 Branch of Electric Circuit Elements in an electric circuit generally have two terminals. When a circuit element connects to the circuit, it does so through both terminals, becoming part of a closed path. Figure 7: elements of circuit. Any circuit element connects between two nodes in the circuit. A branch is the part of the circuit between two nodes that can deliver or absorb energy, excluding short circuits. As per this definition, the short circuit between two nodes is not referred as branch of electric circuit. 3 Figure 8: branch Circuit Element Loops in Electric Circuit Loop is any closed path in the circuit formed by branches. A loop in the network is any closed path through two or more elements of the network where one returns to the starting node without crossing any intermediate node twice. An electric circuit has multiple nodes. If you start at one node and travel through a set of nodes, returning to the starting node without crossing any intermediate node twice, you have completed a loop in the circuit. Figure 9: This is a loop Charge and Current All matter is made of fundamental building blocks known as atoms and that each atom consists of electrons, protons, and neutrons. The concept of electric charge is the underlying principle for explaining all electrical phenomena. Also, the most basic quantity in an electric circuit is the electric charge. Charge is an electrical property of the atomic particles of which matter consists, measured in coulombs (C). The SI unit (the International System of Units) of charge is coulomb, symbolized by C. The coulomb is a large unit for charges. 1 C = 6.24 x 1018 e 1 electron= -1/ 6.24 x 1018 = -1.602 x 10-19 C 1 Proton=1/ 6.24 x 1018 = 1.602 x 10-19 C In 1 C of charge, there are 6.24 x 1018 electrons. The charge e on an electron is negative and equal in magnitude to 1.602 x10-19 C, while a proton carries a positive charge of the same magnitude as the electron. The presence of equal leaves of protons and electrons leaves an atom neutrally charged. 4 Current Current can be defined as the motion of charge through a conducting material. Free electron motion is random unless outside force is applied. Electric current is what happens when the random exchange of electrons that occurs constantly in a conductor becomes organized and begins to move in the same direction. Current – the directed flow of charge through a conductor. The SI unit of current is Ampere. Figure 10: Random motion of free electrons in a material. Figure 11: Electrons flow from negative to positive when a voltage is applied across a conductive or semiconductive material. If a conducting or semiconducting path is provided between two poles having a potential difference (Voltage provides energy to electrons that allows them to move through a circuit), charge carriers will flow in an attempt to equalize the charge between the poles. This flow of electric current will continue as long as the path is provided, and as long as there is a charge difference between the poles. Electrical current is the rate of flow of charge. Current is measured in terms of the number of electrons or holes (amount of charge) passing a single point in one second. Current is often represented by the letter I in electrical equations. The I stand for intensity. The SI unit of current is Ampere whilst charge is measured in Coulombs. Mathematically, I = Q/t Where, I = the intensity of the current in Ampere (A) q = the amount of charge in Coulombs (C) t = the time in seconds (s) required for the charge (q) to pass Definition of an Ampere One ampere (1 A) is the amount of current that exists when a number of electrons having a total charge of one coulomb (1 C) move through a given cross-sectional area in one second (1 s). One ampere (A) is defined as one coulomb of electricity flowing past a given point in one second. 1 ampere = 1 coulomb/second 5 Figure 12: Illustration of 1 A of current (1 C/s) in a material. Current is always measured through a circuit element. Ammeters measure current in amperes and are always wired in series in the circuit. An ammeter is connected in series to measure current, I, through the element, R. Figure 13 demonstrates the use of an ampere-meter or ammeter in series with a circuit element, R, to measure the current through it. Figure 13: An ammeter is connected in series to measure current, I, through the element, R. An ammeter is an instrument for measuring the current flowing through a live circuit. The word ‘ammeter’ is actually an abbreviation for the word ‘ampere meter’… why? It is because the current flowing through a circuit is measured in Amperes (A) and this meter counts that. The internal resistance of an ideal ammeter should be zero so that there is no voltage drop across the ammeter. A direct current (DC) Direct Current (DC) is unidirectional (one way). It is a current that remains constant with time. A constant current (DC) is denoted by the symbol I. Figure 14: Direct Current (DC) Mathematically, I = Q/t Where, I = the intensity of the current in Ampere (A) q = the amount of charge in Coulombs (C) t = the time in seconds (s) required for the charge (q) to pass 6 An alternating current (AC) Alternating Current (AC) which is bidirectional (two way, or back and forth). In AC the flow of current changes its direction forward and backward periodically about 50–60 times every second. In an alternating current (AC) circuit, the electrons reverse direction many times each second. It is a current that varies sinusoidally with time. A time varying current (also known as alternating current or AC) is represented by the symbol i or i(t). Figure 15: Alternating Current (AC). Mathematically, Where, i = the intensity of the current in Ampere (A) dq = the amount of charge in Coulombs (C) dt = the time in seconds (s) required for the charge (dq) to pass The current direction In a given circuit, the current direction depends on the polarity of the source voltage. Current always flow from positive (high potential) side to the negative (low potential) side of the source as shown in the schematic diagram of Figure 16 (a) where Vs is the source voltage, VL is the voltage across the load, and I is the loop current flowing in the clockwise direction. Figure 16: Effect of reversing the voltage polarity on current direction. Please observe that the voltage polarity and current direction in a load is opposite to that of the source. In Source current leaves from the positive terminal. In Load current enters from the positive terminal. 7 A reversal in source voltage polarity changes the direction of the current flow and vice versa as depicted in Figures 16(a) and 16(b). Conventional Current flow Vs Electron Flow It is common to think of current as the flow of electrons. However, the standard convention is to take the flow of protons to determine the direction of the current. There is a lot of confusion around conventional current flow and electron flow. In this section, let us understand their differences. Figure 17: Conventional Current flow Vs Electron Flow. Conventional Current Flow The conventional current flow is from the positive to the negative terminal and indicates the direction in which positive charges would flow. Electron Flow The electron flow is from negative to positive terminal. Electrons are negatively charged and are therefore attracted to the positive terminal as unlike charges attract. The law of conservation of charge It states that the total amount of electric charge in a closed system must remain constant. Thus, the algebraic sum of the electric charges in a system does not change. We now consider the flow of electric charges. A unique feature of electric charge or electricity is the fact that it is mobile; that is, it can be transferred from one place to another, where it can be converted to another form of energy. The net charge of the system being distributed uniformly in the objects. So rather than concentrating negative charge in a few bodies, the charge on the body is evenly distributed throughout by the transfer of the electron, and this can be achieved by the transfer of electrons from higher to lower polarity. Only electrons can be involved in the transfer charges, not protons. 8 Electric Field A charged object creates an electric field. An electric field is an invisible force field caused by an electric charge. It is an alteration in the space (air or vacuum) around the charge. It results in an electric force that is felt by another electric charges when placed close to one another. Whether a charged object enters that space or not, the electric field exists. Electric Field Lines and Direction When a unit charge or point charge (example: positive test charge) is placed in the electric field of another charged particle (Positive or negative), it will experience a force. The strength and direction of the electric field are represented by the imaginary lines that called electric lines of forces or electric field lines. The lines are drawn with arrows to signify the direction. The electric field is a vector quantity having both magnitude and direction. As another charged object enters the space and moves deeper and deeper into the field, the effect of the field becomes more and more noticeable. The concept of electric lines of forces was introduced by Michael Faraday in 1837. Figure 18: electric field direction. If the positive test charge placed in electric field of a positive charged particle, the electric field of the positive charged particle pushed the positive test particle in direction away from it because like charges repels. So, for positive charge, the electric lines of force move away from the center of the charge. Thus, the electric field direction about a positive source charge is always directed away from the positive source. Figure 19: electric field lines of a positive charged particle. But in case of negative charge, If the positive test charge placed in electric field of a negative charged particle, the electric field of the negative charged particle attract the positive test particle in direction toward it because opposite charges attract. So, in case of negative charge, the electric lines of force move towards the center of the charge. Thus, the electric field direction about a negative source charge is always directed toward the negative source. 9 Figure 19: electric field lines of a negative charged particle. Electric Field Equation The strength of the electric field in the space surrounding a source charge is known as the electric field intensity. Mathematically, an electric field is defined as the electric force experienced by a unit charge. The following equation gives the electric field vector. Where, E: Electric field F: Electric force q: Electric charge SI Unit: Newtons/Coulomb (N/C) This explains the Conventional Current flow Vs Electron Flow Figure 20: the Conventional Current flow Vs Electron Flow. 10 Electricity Electricity is a type of energy that can build up in one place or flow from one place to another. When electricity gathers in one place it is known as static electricity (the word static means something that does not move); electricity that moves from one place to another is called current electricity. Static electricity Static electricity is the result of an imbalance between negative and positive charges in an object due to its loss of negative charge by any physical means like rubbing. These charges can build up on the surface of an object until they find a way to be released or discharged. Charge carriers, particularly electrons, can build up, or become deficient, on things without flowing anywhere. You’ve probably experienced this when walking on a carpeted floor during the winter, or in a place where the humidity was very low. An excess or shortage of electrons is created on and in your body. You acquire a charge of static electricity. It’s called “static” because it doesn’t go anywhere. You don’t feel this until you touch some metallic object that is connected to earth ground; but then there is a discharge, accompanied by a spark that might well startle you. It is the current, during this discharge, that causes the sensation that might make you jump. In radiology, Static electricity can be caused by removing the film from the cassette too quickly or due to low humidity. The Static electricity may discharge when the film is handled, leaving an artifact. This discharge appears as black lines or dots on the film often having a tree- branch appearance. with the following precautions the radiologist can prevent the formation of static electricity in the film: 1. Avoid friction during handling the film. 2. Moderate humidity (Maintain humidity of 30-50%). Humidifiers can increase humidity within the storage room falls below 30% or rises above 50%, there is a chance that static electrical charges will build up between sheets of film. Figure 21: examples of Radiographic artifact caused by static electricity. 11 The electromotive force The electromotive force is work done on a unit electric charge. Current is always measured through a circuit element. The amount of current that will flow in an electrical circuit depends on the voltage, and also on the resistance. Electricity comes in two forms: AC and DC power. The terms describe the direction of the electrical current's flow in a circuit. In DC voltage, the current flows in a single direction. For AC, electrons switch directions, going both forward and backward. AC voltage is what the majority of homes, appliances, and electronic devices consume because it is better at transmitting electricity over a longer distance. If you plug into an outlet, AC power will flow from it. Most AC electricity is used for televisions, computers, routers, cell phones, refrigerators, stoves, dishwashers, and water heaters. A generator or battery is used to convert one energy into another. The output of these devices, one terminal is positively charged, and the other is negatively charged. Electric battery (Cell) An electric battery is a device that converts chemical energy into electrical energy and maintains a constant flow of charge in a circuit. A battery is used to provide a continuous steady current (DC) source by way of providing constant EMF or Electromotive force to an electrical circuit or a machine. Battery consists of two rods of different metals which are called electrodes or plates. They are immersed in a liquid called electrolyte. This liquid has this property that when the plates are immersed in it, one plate becomes positively charged and the other becomes negatively charged. The electrolytes in batteries have a finite value of resistance. The resistance generated inside a battery to the flow of current is referred to as the internal resistance of a battery (r). Figure 22: electric battery. When the cell is connected in a circuit chemical reaction start to take place at the anode and the cathode. The anode in the negative terminal of a cell. The cathode is the positive terminal. At the anode, an oxidation reaction removes electrons from the metal, and they begin to flow into the circuit. This leaves the anode positively charged, which attracts more electrons from the electrolyte. The anode returning electrons are collected at the cathode and with time it is reduced. After a while the anode will have lost almost all of its electrons and the electrolyte is unable to supply anymore, so the reaction slows down, and the emf of the cell drops. 12 Figure 23: A simplified example of how a cell works. When the chemical reactions take place, as well as producing electricity, the cell produces a small amount of heat which radiates away from the system and is lost. As the energy lost from a cell is heat, it can be modelled as if it was a resistor generating heat and is called the internal resistance (r) of the cell. The rate of energy lost from a cell is of course measured in watts and the equations of power for a resistor can be used to describe it. Figure 24: When a cell generates a current it also produces heat. Sometimes in circuit diagrams a resistor is drawn in series with a cell to represent the internal resistance; of course there is not actually a resistor inside of a cell, it is just a useful way of describing the energy lost from the circuit. Internal resistance is an analogy for the efficiency of the cell. The greater the internal resistance, the more heat it creates as it produces electricity and the less efficient it is. The internal resistance will also have a potential difference across it, which means that the voltage measured across the terminals when a current is flowing is lower than the actual true value of emf of the cell. The true emf of the cell is these ‘lost volts’ plus the terminal voltage. emf = terminal p.d. + lost volts Figure 25: emf is equal to the terminal p.d. plus the 'lost volts'. We can state this mathematically according to ohms law: E=IR ε=IR+Ir 13 Where: ε is the emf in V I is the current in A R is the total resistance of the circuit in Ω r is the internal resistance of the cell in Ω The term IR represents the terminal p.d. (potential difference) and the term Ir is the lost volts. The above equation can be simplified to: ε=I(R+r) Often in examples of circuits, the internal resistance is neglected, and an ideal cell with no internal resistance is assumed. Typical internal resistance for modern cells is in the region of 100mΩ to 900mΩ. Solar cell (solar panels) A photovoltaic cell (solar cell) generates its own DC electricity when it is exposed to sunlight falling on a special semiconductor chip, and this is stored in the batteries. When the stored energy is used to power a home, an inverter converts it from DC to AC, the standard for household appliances. Some off-grid solar system owners may use DC appliances to avoid the need for an inverter, but their options are limited to a small range of appliances. Figure 26: solar panels. Electrical generators A generator is a mechanical device that converts mechanical energy into electrical energy for use in an external circuit. Sources of mechanical energy include steam turbines, gas turbines, water turbines, internal combustion engines, wind turbines and even hand cranks. Mechanically, a generator consists of a rotating part and a stationary part which together form a magnetic circuit, One of these parts generates a magnetic field, the other has a wire winding in which the changing field induces an electric current. In the simplest form of linear electric generator, a sliding magnet moves back and forth through a solenoid, a copper wire or a coil. An alternating current is induced in the wire, or loops of wire, by Faraday's law of induction each time the magnet slides through. Also, when a coil spins in a magnetic field or moves relative to a magnet, it generates an electromotive force (emf) or potential difference. The emf is caused by a phenomenon known as electromagnetic induction. 14 Electromotive force (EMF) Electromotive force denotes the voltage produced inside the electric source. Electromotive force is the characteristic of any energy source capable of driving electric charge around a circuit. Energy is converted from one form to another in the generator or battery as the device does work on the electric charge being transferred within itself. One terminal of the device becomes positively charged, the other becomes negatively charged. The work done on a unit of electric charge, or the energy thereby gained per unit electric charge, is the electromotive force, abbreviated EMF, or sometimes simply E. But you know it more commonly as voltage. Electromotive force, energy per unit electric charge that is imparted by an energy source, such as an electric generator or a battery. Despite its name, electromotive force is not actually a force. EMF creates and maintains a voltage in the active cell, by supplying energy in Joules to each unit of Coulomb charge. It is commonly measured in units of volts, equivalent to one joule per coulomb of electric charge. EMF is referred to as the maximum potential difference (voltage) between two points of the battery when no current flows from the source in the case of an open circuit. Figure 27: When the switch is in the off position (circuit is open) the voltameter measures the electromotive force (EMF) in the circuit. Voltage A voltage is nothing more than a difference in charge between two places. Electric Potential Difference is the true scientific term but is commonly called Voltage. Informally, voltage or electric potential difference is sometimes called "Potential Difference". Technically, the voltage is the difference in electric potential between two points and is always measured between two points. e.g. between the positive and negative ends of a battery, between a wire and the ground, or between a wire or a point of a circuit and a point in another part of the circuit. In everyday use with household electricity in the U.S. the voltage is most often 120V. This voltage is measured from the electric wire to the ground. In case of closed circuit, when a power source delivers current, the measured voltage output is lower than the voltage when no current is flowing through the circuit. EMF is affected 15 by the voltage drop caused by the internal resistance of the battery to the flow of current through it. So, EMF always greater than the voltage (the potential difference across the battery). It also affected by the voltage drop across circuit elements. Figure 27: When the switch is in the on position (circuit is closed) the voltameter measures the potential difference (voltage) in the circuit. Resistance in Electricity can be linked to Friction in Mechanics. The electrical resistance of a body is the measure of the difficulty in passing an electric current through it. When electricity encounters resistance, potential energy is lost because it is converted into another form of energy to do the work. The higher the resistance of a component, the greater the voltage drop between connections. Voltage drop is a drop in potential along the current path through a circuit. For example, electric potential energy is converted into thermal energy by a resistor. The mathematical representation of voltage (ohms law) is as follows: V=IxR Where, V = Voltage in volts, I = Current in amperes, R = Resistance in ohms Voltage is always measured across a circuit element as demonstrated in Figure 28. A voltmeter is connected in parallel with the circuit element, R to measure the voltage across it. The voltmeter is a measurement device used to measure voltage in electrical circuits or electronic devices. It is commonly employed to measure the voltage across a circuit or component, typically expressed in volts. Voltage represents the electrical potential difference or the rate of transfer of electrical energy from one point to another. Voltmeters have high internal resistance, allowing only a minimal current to pass through when the measurement probe or tip is connected to the circuit. This ensures that the meter does not significantly affect the circuit during measurement. An important application of the voltameter that it is used for Electrical Troubleshooting, Electrical engineers and technicians use voltmeters for troubleshooting and diagnosing electrical issues. By monitoring voltage levels, they can identify faulty components.. Figure 28: A voltmeter is connected in parallel with the circuit element, R to measure the voltage across it. 16 Like electric current, a constant voltage is called a dc voltage and is represented by V, whereas a sinusoidally time-varying voltage is called an ac voltage and is represented by v. A dc voltage is commonly produced by a battery; ac voltage is produced by an electric generator. In an alternating circuit, voltage periodically reverses itself. When the voltage reverses, so does the direction of the current flow. Current and voltage are the two basic variables in electric circuits. Current is the organized flow of electric charges through a conductor, and voltage is the driving force that pushes electric charges to create current. Volt can also be defined as electric potential along a wire when an electric current of one ampere dissipates one watt of power. V = Dissipated Power/Current = P/I Unit: Watt/Ampere Power The third piece of the puzzle is called power (abbreviated P in equations). Power is the rate at which energy is expended. Electric current, in and of itself, isn’t all that useful. It becomes useful only when the energy carried by an electric current is converted into some other form of energy, such as heat, light, sound, or radio waves. In all cases, the energy is “used up” somehow, converted from one form into another form at a certain rate. For example, in an incandescent light bulb, voltage pushes current through a filament, which converts the energy carried by the current into heat and light. A resistor converts electrical energy into heat energy, at a rate that depends on the value of the resistance and the current through it. A light bulb converts electricity into light and heat. A radio antenna converts high-frequency AC into radio waves. A speaker converts low- frequency AC into sound waves. The power in these forms is a measure of the intensity of the heat, light, radio waves, or sound waves. The term dissipate is often used in association with power. As the energy carried by an electric current is converted into another form such as heat or light, the circuit is said to dissipate power. Calculating the power dissipated by a circuit is often a very important part of circuit design. That’s because electrical components such as resistors, transistors, capacitors, and integrated circuits all have maximum power ratings. For example, the most common type of resistor can dissipate at most ¼ watt. If you use a ¼ -watt resistor in a circuit that dissipates more than ¼ watt of power, you run the risk of burning up the resistor. In DC circuits, and also in AC circuits having no reactance, power can be defined this way: Power is the product of the voltage across a circuit or component, times the current through it. The standard unit of power is the watt, abbreviated W; it is equivalent to one joule 17 per second. The definition of one watt is simple: One watt is the amount of work done by a circuit in which one ampere of current is driven by one volt. Mathematically this is written P=E x I In other words, Power (P) equals voltage in volts (E) times current in amperes (I), then P is in volt-amperes (VA). This translates into watts when there is no reactance in the circuit. Sometimes power is given as kilowatts (kW or thousands of watts), megawatts (MW or millions of watts) or gigawatts (GW or billions of watts). It might be given as milliwatts (mW or thousandths of watts), microwatts (µW or millionths of watts), or nanowatts (nW or billionths of watts). figure 29: When there is no reactance in a circuit, the power, P, is the product of the voltage E and the current I. 18