MECH 6491 Engineering Metrology Lecture 1 PDF
Document Details
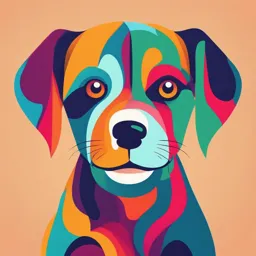
Uploaded by TrustyGenius
Concordia University
Dr. S. Narayanswamy
Tags
Summary
This document is a lecture for a course on Engineering Metrology and Measurement Systems. It covers an overview of metrology, including its importance, history, and economic benefits in areas like semiconductor manufacturing. The lecture topics include linear and geometric tolerances, methods in surface measurement, fundamentals of optical metrology, and more.
Full Transcript
MECH 6491 Engineering Metrology and Measurement Systems Credits: 4 Introduction Lecture 1 Lecturer contact details Instructor: Dr. S. Narayanswamy Office: Engineering and Visual Arts Building Room: EV 4-189 Office Hours: M _ W _ _ 12:00 –13:00 or by email e-mail: [email protected]...
MECH 6491 Engineering Metrology and Measurement Systems Credits: 4 Introduction Lecture 1 Lecturer contact details Instructor: Dr. S. Narayanswamy Office: Engineering and Visual Arts Building Room: EV 4-189 Office Hours: M _ W _ _ 12:00 –13:00 or by email e-mail: [email protected] Web site: Moodle Mission of the course • Enables the students to learn the techniques and standard practices in metrology • At the end of the lectures, one would be able to: – Have clear idea of challenges in metrology due increasing trend towards miniaturization – Understand many different metrological devices and principles and applicability of those devices – Understand the process and provide metrological solution for the betterment of part or process * The amount of acquired skills will be proportional to the capabilities, will and effort of the individuals Outline of the Course Week Date Lecture Topics 1 5th Sep Need for Metrology – an overview 2 12th Sep Linear and Geometric Tolerances 3 19th Sep Methods in Surface Measurement 4 26th Sep Fundamentals of Optical Metrology 5 3rd Oct Optical interferometry – theory and overview 6 17th Oct Moiré and phase shifting interferometry (Term Test) 7 24th Oct Speckle Interferometry and Holography 8 31st Oct Light sources, detectors and imaging systems 9 7th Nov Application to precision measurement and MEMS devices 10 14th Nov Special Topics - Nanometrology, Bio Metrology 11 21st Nov Special Topics - Spectroscopy 12 28th Nov Student Presentations About the course • Metrology is the science of measurement that deals with resolution, accuracy and repeatability – Lectures - 2.5 hours each – 11 Lectures in all - one is an introductory lecture – 1 Project – presentation due during the last week of classes – Final exam Class logistics • 3 Continuous teaching hours • Once a week _ T _ _ _ 10:15 – 12:45 • Lecture room FG B 040 • 11 (or 10) lectures + 1 Project Presentations • Final exam Text book and other reference TEXTBOOK • 1. 2. 3. 4. There is no prescribed textbook for this course. Lecture notes available at the course webpage will be enough provided effort is done to research information from the references REFERENCES (not exhaustive) Gary Cloud: Optical Methods of Engineering Analysis, Prentice Hall; 1998 Eugne Hecht: Optics, Addison-Wesley Pub Scarr: Metrology and Precision Engineering, Mc.Graw Hill, 1995. Hariharan: Basics of Interferometry, Prentice-Hall, Ninth Edition, 1981. The Assignment • There will be two assignments. The first one will have some problems to solve and some theory questions to answer and is due – date will be specified in the course webpage. • The second assignment is slightly different involving review of a journal paper (provided by the instructor). • Review should be critical, detailed, and thorough - extended abstract of strictly less than 500 words – Due date will be specified in the course webpage. • Proper Concordia guidelines of referencing to be followed. Plagiarism in any form is strictly prohibited - penalized with 0 marks. The Term Test • Term test will be held during lecture on the 17th of October • The exam will be conducted in person • Will contain multiple choice questions, short answer questions, and few problems to solve. • Will discuss the modalities during class • Exam will be in SCANTRON Sheet The Project • A project in the topics related to Metrology. • 6 minute presentation per student (depending on the number of students/teams after DNE) • Literature review and theoretical work will be needed to complete the work. • The project will be graded on the presentation and the powerpoint slides that you will submit in moodle • Presentation on the last lecture day. Details available in moodle Final Test • The final test will be conducted on the last but one lecture or it will be conducted by the exam office during the examination period. We can decide about this closer to the end date. • A number of multiple choice, short answer and comprehensive questions will require answer. • Duration of the test: 3 hours • Write the final exam with confidence that you will do very well Grading Scheme • Grade composition: – Assignment 20% – Term Test 10% – Project 20% – Final 50% • To pass the course you have to – Pass the final – Submit your project and assignment Promptly Content of the Lecture • History and Philosophy of measurement • Economic benefits • International trade • Calibration and Traceability • Current manufacturing trend • Need for precision measurement What is Metrology? Metrology is the science of measurement, embracing both experimental and theoretical determinations at any level of uncertainty in any field of science and technology Why is it important? • When you can measure what you are speaking about, and express it in numbers, you know something about it; • but when you cannot express it in numbers, your knowledge is of a meager and unsatisfactory kind. • It may be the beginning of knowledge, but you have scarcely, in your thoughts, advanced to the stage of science. Lord Kelvin Why is it important? • Wrong or inaccurate measurements can lead to wrong decisions, that have serious consequences, costing money and even lives. Eg.. Metric mishap caused loss of NASA orbiter • Failure convert English/US system made the probe travel 60 miles farther and it was lost. (125m USD in 1999 september) • It is important to have reliable and accurate measurements which are agreed and accepted by the relevant authorities worldwide. • Metrologists are therefore continuously involved in the development of new measurement techniques, instrumentation and procedures, to satisfy the ever-increasing demand for greater accuracy, and increased reliability. A bit of history • One of the earliest records of precise measurement is from Egypt. The Egyptians studied the science of geometry to assist them in the construction of the Pyramids. It is believed that about 3000 years BC, the Egyptian unit of length came into being. www.fairchild-controls.com/ methistory.html depts.washington.edu/ ctltstaf/cc/gallery.shtml A bit of history • The "Royal Egyptian Cubit" was decreed to be equal to the length of the forearm from the bent elbow to the tip of the extended middle finger of the hand of the Pharaoh or King ruling at that time www.fairchild-controls.com/ methistory.html History in Images History in Images History in Images History in Images History of Metrology • Although standardization has been a goal of social and economic advancement since very early times, only in 18th century that there was a unified measurement system • The earliest systems of weights and measures were based on human morphology. Consequently, these units of measurement were not fixed; they varied from one town to another, from one occupation to another, and on the type of object to be measured • This lack of a standardized system of measurements was a source of error and fraud in commercial and social transactions, putting a brake on international commerce and prevented the development of science as an international endeavor Economic benefits of Metrology • Metrology is Value Added • It is often erroneously stated that “metrology is not value added to the product” but, today it is becoming a key enabler in a number of areas • Metrology is a check and a balance in the manufacturing process • Metrology does provide an economic benefit • It is often difficult to assess this economic benefit as it is often hidden and often forgotten The $B Wafer Industry If it can’t be repeatedly measured, then it is just an opinion." DeVere Bobier • process-control is based on measurements • faulty measurements... • loss of control • form & function failure • loss of profits, wealth 30 cm U= 30 nm Economic Impact • Measurement and measurement related operations are estimated to account for between 3% and 6% of the GDP of industrialized countries • Other studies for developed countries find 15% of GDP is measurement-related • Government investments in national metrology of developed countries vary between 20 and 70 ppm of GDP Economic Impact • UK study (on behalf of NPL and DTI) find: • Measurement in the UK delivers a significant impact on the economy of 0.8% of GDP, which equates to 5 000 million GBP • Government budget of NPL is about 38 million GBP per annum Economic Impact • NIST studies find that NIST investments lead to industry savings including: • Lower transaction costs • Lower regulatory compliance costs • Energy conservation • Increased product quality • Enabling new markets • NIST leverage factors, like benefit-cost-ratio varies from 3 to 110 Economic Impact • EU study demonstrates: • IVD (in-vitro diagnostic) industry expects a larger market • Automotive industry is extremely globalized and cannot do without international metrology infra-structure • European Union relies largely on gas consumption, which is internationally traded (sold at volume compensated at 15°C) Economic benefits of Metrology Example: Semiconductor Manufacturing What’s a Nanometer worth? •Faster microprocessors fetch higher prices •Narrower gate =>less capacitance => more speed For 180nm gates, a 10nm improvement in CD control was estimated to an increase of $100 market value per microprocessor “Under these assumptions, the value of CD control for the 180 nm generation of microprocessors exceeds $10 per nanometer.” C.P. Ausschnitt and M. E. Lagus, IBM Advanced Semiconductor Technology Center, Proc. SPIE Vol. 3332, p. 212 (1998). 1999 Worldwide PC sales exceed 113 million units (Source: International Data Corp.) >108units ×$10/unit ≥$1 billion/nm It’s a big industry, and small improvements yield big economic benefits…. Credit: John Villarrubia, NIST Economic benefits of Metrology imagine a fighter jet traveling in excess of Mach 5 (nearly 4,000MPH) at less than 1-inch off the ground, and being able to stop on any given blade of grass. Flying height in Hard Disk Drive * 2002 20nm 2004 < 7nm Current 4nm * Centre for Magnetic Recording Research, University of California, San Diego International Trade • • • • Manufactured products Parts Services Have to fulfil – specifications – regulations • Are delivered worldwide • Therefore measurements have to be comparable (traceable) International Trade • Is an essential and often hidden part of the technical infrastructure • Underpins any large scale industrial revolution in history • Underpins the conformance structure of any modern economy • Confidence in results of measurements is a prerequisite to international trade* CROSS-BORDER RECOGNITION Product Certification Accreditation System Quality System Certification Inspection, Testing & Calibration National Measurement System Economy A C O N F I D E N C E (trade dispute) Product Certification Accreditation System Quality System Certification Inspection, Testing & Calibration National Measurement System Economy B * Intercomparison of scanning probe microscopes – Precision Engineering (26) International Trade The importance of metrology to trade Drivers • Reliable, traceable, comparable measurements • Taking away Technical Barriers to Trade • Trade agreements, accreditation agreements and metrology agreements • Once tested/measured, everywhere accepted Accuracy and Precision • When we talk about traceability of measurement and comparison with other measurement methods, one need to know the difference between two basic aspects in measurement – ACCURACY – PRECISION Accuracy and Precision Perhaps the easiest way to illustrate the difference between accuracy and precision is to use the analogy of a marksman, to whom the "truth" represents the bulls eye. Accuracy the degree of conformity with a standard (the "truth"). Accuracy relates to the quality of a result, and is distinguished from precision, which relates to the quality of the operation by which the result is obtained. In Figure, the marksman has approached the "truth", although without great precision. It may be that the marksman will need to change the equipment or methodology used to obtain the result if a greater degree of precision is required, as he has reached the limitations associated with his equipment and methodology. Accuracy Accuracy and Precision Precision : the degree of refinement in the performance of an operation, or the degree of perfection in the instruments and methods used to obtain a result. An indication of the uniformity or reproducibility of a result. Precision relates to the quality of an operation by which a result is obtained, and is distinguished from accuracy, which relates to the quality of the result. In Figure, the marksman has achieved a uniformity, although it is inaccurate. This uniformity may have been achieved by using a sighting scope, or some sort of stabilizing device. Precision With the knowledge gained by observation of the results, the marksman can apply a systematic adjustment (aim lower and to the left of his intended target, or have his equipment adjusted) to achieve more accurate results in addition to the precision that his methodology and equipment have already attained. Accuracy and Precision Accuracy and Precision - Example A metal rod about 4 inches long has been passed around to several groups of students. Each group is asked to measure the length of the rod. Each group has five students and each student independently measures the rod and records his or her result Group Student 1 Student 2 Student 3 Student 4 Student 5 Average Group A 10.1 10.4 9.6 9.9 10.8 10.16 Group B 10.135 10.227 10.201 10.011 10.155 10.146 Group C 12.14 12.17 12.15 12.14 12.18 12.16 Group D 10.05 10.82 8.01 11.5 10.77 10.23 Group E 10 11 10 10 10 10.2 Which group is most accurate? Unknown because true value is not known Which group has greatest error? Unknown because true value is not known Which group is most precise? C because measurements are repeatable Which group has most uncertainty D because maximum variation in results Accuracy and Precision - Example We now receive a report from the machine shop where the rod was manufactured. This very reputable firm certifies the rod to be 4 inches long to the nearest thousandths of an inch. Answer the questions below given this new information Group Student 1 Student 2 Student 3 Student 4 Student 5 Average Group A 10.1 10.4 9.6 9.9 10.8 10.16 Group B 10.135 10.227 10.201 10.011 10.155 10.146 Group C 12.14 12.17 12.15 12.14 12.18 12.16 Group D 10.05 10.82 8.01 11.5 10.77 10.23 Group E 10 11 10 10 10 10.2 Which group is least accurate? C because most away from true value Which group has smallest error? A because average is close to true value Which group is least precise? D because maximum variation in results Which group has least uncertainty C because measurements are repeatable Calibration and Traceability • The International Vocabulary of Basic and General Terms in Metrology (VIM)1 defines traceability as: "property of the result of a measurement or the value of a standard whereby it can be related to stated references, usually national or international standards, through an unbroken chain of comparisons all having stated uncertainties.“ • The first thing to notice is that only the result of a measurement or the value of a standard can be traceable. • Measuring equipment cannot be traceable in and of itself. • What is traceable about the equipment is the determination of its imperfections during calibration. What we mean when we talk about traceable equipment is that it is potentially able to produce traceable measuring results. Calibration and Traceability • A standard cannot be traceable, but the value assigned to it can. ISO/QS 9000 requires calibration (of inspection, measuring and test equipment) against certified equipment having a known valid relationship to internationally or nationally recognized standards. • To understand this question, we need to look at the purpose of the traceability requirement. • The customer requiring traceability wants some assurance that the measurements are "right". • The only way to prove that measurements are right, is to prove that their uncertainty is low enough to allow the desired conclusions to be drawn from the results, such as whether or not a workpiece meets its specification. Calibration and Traceability Traceability Chain Schematic representation of the various types of standard that exist in a particular area of metrology, and how the level of precision will decrease along the chain of responsibility Calibration and Traceability Traceability Chain Primary standards Calibration and Traceability Traceability Chain Example Calibration and Traceability Uncertainties of physical realizations of the base SI units Calibration and Traceability • Traceability may therefore require calibration of several attributes of the measuring equipment and not all of them may be in the unit of what we are measuring. For example the uncertainty of a length measurement may be highly dependent on temperature and therefore the ability to measure temperature. Thus the traceability of the calibration of the temperature sensor becomes a significant part of the uncertainty for the length measurement. • Using this logic, the information needed to prove that a measurement is traceable in the technical sense is: • A list of the significant uncertainty contributors for the measurement. Calibration and Traceability • A list of the equipment (serial no. etc.) used in the measurement that adds significantly to the uncertainty. • For each piece of equipment a reference to its traceability (Calibration scope, calibration source, calibration date and calibration id, e.g. certificate number). • For each calibration source, evidence of its credibility, e.g. accreditation. • The requirement of credibility of the calibration source is what recursively ensures that this information is available at each link in all the chains back to the national laboratory level. • Accreditation is intended to provide this credibility. Accreditation is essentially a third party putting a seal of approval on the comparisons and the accompanying uncertainties that a calibration laboratory performs. Manufacturing Trend The Machine Revolution 1800-1920 – the milli-inch (1 mil = 25 µm) • Mass production of firearms, sewing machines, automobiles, etc. • Process: machining, stamping, casting, forging, etc. • Essential requirement: accurately dimensioned, interchangeable machine parts. • Enabled by widespread dissemination of accurate length scale (~1 mil) embodied as gauge blocks. • Accuracy transferred to work piece by vernier calipers. Manufacturing Trend The Machine Revolution 1800-1920 – the milli-inch (1 mil = 25 µm) Manufacturing Trend The Machine Revolution 1800-1920 – the milli-inch (1 mil = 25 µm) Manufacturing Trend The Machine Revolution 1800-1920 – the milli-inch (1 mil = 25 µm) Manufacturing Trend The Semiconductor Revolution 1950-2010 – the micro-meter (1 µm = 1000 nm) • Mass production of semiconductor circuits. • Process: planar multi-level lithographic processing. • Essential requirement: accurately dimensioned and placed patterns. • Enabled by widespread dissemination of accurate length scale (~0.1 µm) embodied as laser interferometers. • Accuracy transferred to work piece by optical or electron imaging. Manufacturing Trend The Semiconductor Revolution 1950-2010 – the micro-meter (1 µm = 1000 nm) Manufacturing Trend The Semiconductor Revolution 1950-2010 – the micro-meter (1 µm = 1000 nm) Current Manufacturing Trend The Nanotechnology Revolution 2000-2050 – the nano-meter (nm) • Mass production of nanosystems (electronic, mechanical, biological, etc.). • Process: planar multi-level lithography; self assembly of nanoobjects (e.g., nanowires, nanocrystals). • Essential requirement: accurately dimensioned and placed patterns and nano-objects. • Enabled by widespread dissemination of accurate length scale (~1 nm) embodied as optical encoders. • Accuracy transferred to work piece by optical, electron or atom imaging, nano-tip microscopes. Current Manufacturing Trend The Nanotechnology Revolution 2000-2050 – the nano-meter (nm) • (A) shows a hand holding a computer chip. This is shown magnified 100 times in (B). Another factor of 100 magnification (C) shows a living cell placed on the chip to show scale. • Yet another factor of 100 magnification (D) shows two nanocomputers beside the cell. The smaller (shown as block) has roughly the same power as the chip seen in the first view; the larger (with only the corner visible) is as powerful as mid-1980s mainframe computer. • Another factor of 100 magnification (E) shows an irregular protein from the cell on the lower right, and a cylindrical gear made by molecular manufacturing at top left. • Taking a smaller factor of 10 jump, (F) shows two atoms in the protein, with electron clouds represented by stippling. A final factor of 100 magnification (G) reveals the nucleus of the atom as a tiny speck. Current Manufacturing Trend The Nanotechnology Revolution 2000-2050 – the nm What started this revolution? This possibility was first advanced by Richard Feynman in 1959 when he said: "The principles of physics, as far as I can see, do not speak against the possibility of maneuvering things atom by atom." His talk entitled "There Is Plenty Room At the Bottom" has become a classic milestone. • proposed that tiny robots might be able to build chemical substances. He noted that they could be used to create nanomachines. • pointed out that a new class of miniaturized instruments would be needed to manipulate and measure the properties of the small "nano" structures. In the 1980's these instruments were invented. • The scanning tunneling microscope, atomic force microscope and the near-field microscopes provided the eyes and fingers required for nanostructure measurement and manipulation. Current Manufacturing Trend A visual imagery* A nanomachine swimming through a capillary attacks a fat deposit 100 nm-scale manipulator arm estimated to contain about 4 million atoms Bearing These miniature devices would roam between the red cells of the bloodstream, seeking out and destroying harmful viruses Medical nanodevices could augment the immune system Success in moving atomsXenon on Nickel (110)+ by finding and disabling unwanted bacteria and viruses. +IBM image gallery *www.zyvex.com/ nanotech/visuals.html To put things in perspective • Good metrology practice is more than just reading numbers from a data sheet. • In order to measure a quantity accurately, it is necessary to fully study and understand the entire measurement process itself • This may require multiple disciplines working closely together to achieve the final goal What are the Challenges? • History teaches that metrology infrastructure essential for manufacturing. • Metrology technology must be developed well before manufacturing can proceed. • Current lithography and metrology infrastructure inadequate for non-IC nanotechnology. • Funding for metrology technology (i.e., high resolution, low cost tools) • Improved microscopes (higher resolution, lower noise & drift). • Atoms beams? Electron holography? SW nanotubes? X-rays? • Resolution standards in the nano-range. • Improved substrate stages with accuracy in the sub-nanometer range MECH 6491 Engineering Metrology and Measurement Systems Lecture 2 Instructor: N R Sivakumar Current Manufacturing Trend The Nanotechnology Revolution 2000-2050 – the nano-meter (nm) • Mass production of nanosystems (electronic, mechanical, biological, etc.). • Process: planar multi-level lithography; self assembly of nanoobjects (e.g., nanowires, nanocrystals). • Essential requirement: accurately dimensioned and placed patterns and nano-objects. • Enabled by widespread dissemination of accurate length scale (~1 nm) embodied as optical encoders. • Accuracy transferred to work piece by optical, electron or atom imaging, nano-tip microscopes. Current Manufacturing Trend The Nanotechnology Revolution 2000-2050 – the nano-meter (nm) • (A) shows a hand holding a computer chip. This is shown magnified 100 times in (B). Another factor of 100 magnification (C) shows a living cell placed on the chip to show scale. • Yet another factor of 100 magnification (D) shows two nanocomputers beside the cell. The smaller (shown as block) has roughly the same power as the chip seen in the first view; the larger (with only the corner visible) is as powerful as mid-1980s mainframe computer. • Another factor of 100 magnification (E) shows an irregular protein from the cell on the lower right, and a cylindrical gear made by molecular manufacturing at top left. • Taking a smaller factor of 10 jump, (F) shows two atoms in the protein, with electron clouds represented by stippling. A final factor of 100 magnification (G) reveals the nucleus of the atom as a tiny speck. Current Manufacturing Trend The Nanotechnology Revolution 2000-2050 – the nm What started this revolution? This possibility was first advanced by Richard Feynman in 1959 when he said: "The principles of physics, as far as I can see, do not speak against the possibility of maneuvering things atom by atom." His talk entitled "There Is Plenty Room At the Bottom" has become a classic milestone. • proposed that tiny robots might be able to build chemical substances. He noted that they could be used to create nanomachines. • pointed out that a new class of miniaturized instruments would be needed to manipulate and measure the properties of the small "nano" structures. In the 1980's these instruments were invented. • The scanning tunneling microscope, atomic force microscope and the nearfield microscopes provided the eyes and fingers required for nanostructure measurement and manipulation. Current Manufacturing Trend A visual imagery* A nanomachine swimming through a capillary attacks a fat deposit 100 nm-scale manipulator arm estimated to contain about 4 million atoms Bearing These miniature devices would roam between the red cells of the bloodstream, seeking out and destroying harmful viruses Medical nanodevices could augment the immune system by finding and disabling unwanted bacteria and viruses. *www.zyvex.com/ nanotech/visuals.html Success in moving atomsXenon on Nickel (110)+ +IBM image gallery To put things in perspective Good metrology practice is more than just reading numbers from a data sheet. In order to measure a quantity accurately, it is necessary to fully study and understand the entire measurement process itself This may require multiple disciplines working closely together to achieve the final goal What are the Challenges? History teaches that metrology infrastructure essential for manufacturing. Metrology technology must be developed well before manufacturing can proceed. Current lithography and metrology infrastructure inadequate for non-IC nanotechnology. Funding for metrology technology (i.e., high resolution, low cost tools) Improved microscopes (higher resolution, lower noise & drift). Atoms beams? Electron holography? SW nanotubes? Xrays? Resolution standards in the nano-range. Improved substrate stages with accuracy in the subnanometer range Outline ◼ Conventional surface measurement methods ◼ Stylus based methods ◼ Non-optical methods ◼ ◼ scanning electron microscopy ◼ atomic force microscopy ◼ scanning capacitance microscopy Optical methods ◼ Microscopy ◼ Focus sensing systems ◼ Interferometry What is surface measurement? ◼ Often product quality is associated with surface roughness ◼ A smooth surface is usually more expensive to make ◼ The machining process will determine the roughness ◼ Very few surfaces are completely smooth ◼ On a microscopic level most surfaces exhibit waviness and roughness ◼ Measurements of surface profiles are carried out to better visualize the surface, and to quantify roughness. ◼ Statistical formulae are applied to the data collected and standard measures are obtained. Examples of surfaces ◼ Telescope Mirror ◼ Primary property: Has to be reflective ! ◼ “Rest of material” - support and shape. ◼ Brake pad ◼ Primary properties: high friction and low wear over wide temperature range ◼ Secondary properties: right shape. ◼ Machine tools ◼ Primary Property: high hardness and abrasion and oxidation resistance ◼ Secondary properties: right shape ◼ Semiconductor substrates ◼ Absolute smoothness and freedom from defects ◼ Strength, etc. Substrate may need different electrical properties. Surface Vs. Cost Surface topography Measurement of Surface topography ◼ Commonest industrial (& lab) method is to use stylus profilometry ◼ Sharp point is dragged across surface, and up and down movement recorded ◼ Beware! Vertical and horizontal scales often very different - may be misleading Real profile - 1:1 Vert/Horz - 15:1 Vert/Horz - 300:1 These are same surface! Profile parameters ◼ Average roughness Ra ◼ Also called as CLA or AA ◼ Arithmetic mean deviation of surface height from mean value. (Equal areas of profile above and below mean line) L 1 Ra = y ( x )dx L0 ◼ When evaluated from digital data, the integral is normally approximated by a trapezoidal rule 1 N Ra = y x N x =1 Initial Surface ◼ Take an arbitrary surface profile ◼ We must first find the datum from which measurements are taken. Establish the Datum Line ◼ The horizontal line is placed so that there is an equal area of color above and below it. This line is the datum from which measurements are taken. Add the Samples Together ◼ Divide the datum line into a number of sample points over the sample length. ◼ At each sample point draw a vertical line from the datum to the profile line. ◼ Add all these sample lengths together and divide by the number of samples. You Just Calculated the Ra ◼ The number you calculated is the Ra. It is the average distance the profile differs from the datum. ◼ Other names are Arithmetic Average (AA), Center Line Average (CLA) or Arithmetical Mean Deviation ◼ Even though the surfaces look different to the eye, these set of surfaces have same Ra Ra is Misleading Profile parameters ◼ RMS roughness Rq ◼ If surfaces differ in shape or spacing, we need to measure peaks and valleys and profile shape and spacing ◼ Square mean deviation of surface height from mean value ◼ Rq has now superseded by Ra In metal machining specs ◼ It is more directly related to the optical quality of a surface ◼ The digital equivalent is L 1 2 y ( x ) dx Rq = L0 1 N 2 Rq = (y x ) N x =0 Profile parameters ◼ Rp Rv and Rt ◼ Rp is the height of the highest peak in the evaluation length ◼ Rv is the height of the deepest valley in the evaluation length ◼ Rt is the sum of both heights Rp + Rp =Rt ◼ Used to find extreme cases: ◼ a sharp spike or burr on the surface that would be detrimental to a seal ◼ a crack or scratch that might be indicative of poor material or processing Profile parameters - Statistical ◼ Amplitude Distribution Function (ADF) ◼ A surface could be characterized by a number ◼ If few samples of measurements are taken on a surface, different numbers for Ra and Rq could be recorded ◼ Ra and Rq are widely used due to the great convenience, but they do not provide: ◼ ◼ Any information about distribution of heights; ◼ Any information about length scale in surface; ◼ Any information about possible anisotropy ADF is a probability function that gives the probability that a profile of the surface has a certain height, z, at any position x Profile parameters - Statistical Amplitude distribution function ◼ The ADF has a bell shape like many probability distributions ◼ The ADF tells "how much" of the profile lies at a particular height ◼ It is the probability that a point on the profile at a randomly selected x value lies at a height within a small neighborhood of a particular value z: Profile parameters - Statistical Bearing Ratio ◼ ◼ ◼ ◼ ◼ The Bearing Ratio Curve mathematically is the integral of ADF It is the corresponding cumulative probability distribution It has much greater use in surface finish It shows what linear fraction of a profile lies above a certain height (compared to the ADF which tells how much of a surface lies at a given height). Tells about shape, plateau, peaks, valleys ◼ Mathematically the bearing ratio curve can be calculated from ADF ◼ Or calculated directly from a profile Calculated from highest peak downward Horizontal ref line intersects the surface over 15% of its length Profile parameters - Statistical Surface Roughness Trace Amplitude Distribution Function Bearing Ratio Profile parameters - Statistical Skewness (third moment of ADF) ◼ ◼ ◼ ◼ ◼ Skewness is another parameter that describes shape of ADF is a measure of asymmetry of ADF (it is non dimensional) positive skewness, (turned surfaces) have high spikes negative skewness, (porous surfaces) have deep valleys More random (e.g. ground) surfaces have a skew near zero: l Rsk 1 3 = z ( x )dx 3 LRq 0 Digital equivalent Rsk 1 = NRq3 N 3 Z x x =1 Profile parameters - Statistical Kurtosis (fourth moment of ADF) ◼ ◼ ◼ ◼ ◼ Kurtosis is the last ADF shape parameter considered It relates to the uniformity of ADF or, the spikiness of the profile It is non dimensional In statistics, a probability distribution can be constructed from all its moments The more moments are known, the more precisely the shape of the distribution is known. Rku 1 = NRq4 N 4 Z x x =1 Surface topography – measurement techniques Magnification range Method Optical Microscopy Scanning Electron Microscopy (SEM) Transmission Electron Microscopy (TEM) At lowest At highest X 20 X 1000 Resolution at maximum magnification (microns) Horizontal 0.5 vertical 0.01* Depth of field (microns) Lowest 5 Highest Comments 0.5 * Using interference contrast 0.03+ X 20 X 2000 Surface Profilometry X 50 X 100000 X 200000 X 100000 0.015 0.0005 5* 1* 0.0005 0.05 + Using 1000 5 1 Taper section 0.2 Operates in vacuum; limits on specimen size 0.1 Operates in vacuum; requires replication of surface 0.5 Operates along linear track. * Limited by stylus radius Surface Measurement Methods ◼ The earliest ways of measuring surfaces ◼ Thumbnail ◼ Eye ◼ Both of these are highly effective but subjective ◼ Demand for quantitative results led to the development of two branches of instrumentation: ◼ one following the tactile example of the nail, (contact type) ◼ the other mimicking the eye (non-contact type) Surface Measurement Methods Contact Type ◼ This mimics the thumbnail (Eg. CMM or Talysurf) ◼ A stylus tip is used for measuring the surface ◼ Stylus with tip size of approximately 0.1 to 10m traverse the surface. ◼ As the stylus tracks the surface peaks and valleys, its vertical motion is converted to a time varying electrical signal ◼ The resulting data set is stored in a computer for statistical analysis Surface Measurement Methods Contact Type CMM f1engineering.com Talysurf www.ijs.si Surface Measurement Methods Contact Type ◼ Stylus-based instruments have 3 reference guideways ◼ In this coordinate system, the stylus moves inside the measurement volume to acquire measurement data ◼ The probe head moves towards the workpiece and the movement in the X, Y and Z direction are tracked by the computer ◼ On contacting the workpiece, the actual coordinates are transferred to the computer Surface Measurement Methods Contact Type - disadvantages ◼ Scanning is time consuming ◼ Soft materials or bio-medical samples, which might be damaged or scratched by touch ◼ In process measurement is not possible ◼ Limitations in resolution and accuracy due to mechanical movement and detection Surface Measurement Methods Non-Contact surface Measurement* Non optical methods Scanning Tunneling Microscopy Optical methods Focus sensing techniques s Atomic force Microscopy Scanning Electron Microscopy Interferometric techniques s Scanning Capacitance Microscopy *Not Exhaustive STM - Introduction ◼ The scanning tunneling microscope (STM) is the ancestor of all scanning probe microscopes ◼ It was invented in 1981 by Gerd Binnig and Heinrich Rohrer at IBM Zurich ◼ Five years later they were awarded the Nobel prize in physics for its invention ◼ The STM was the first instrument to generate real-space images of surfaces with atomic resolution Scanning Tunneling microscopy Table Z Scanning table Probe Path Sample Force detection system Granite table Table X Table Y STM Working Principle ◼ A sharp tip is moved over the surface and a voltage is applied between probe and the surface ◼ Depending on the voltage electrons will "tunnel" resulting in a weak current proportional to the distance between probe and the surface. ◼ A feedback loop keeps the tunneling current constant by adjusting the distance between the tip and the surface (constant current mode) ◼ By scanning the tip over the surface and measuring the height, surface structure of the material under study can be constructed ◼ The material should be a conductor for this principle STM Working Principle STM Working Principle Constant height mode I e(-2Kd) K (2m)1/2 0.51()1/2 Constant current mode I : Tunneling current; d: distance between probe and sample; m : the electron mass; : effective local work function, eV K: decay length Å Current decreases by an order of magnitude if d increases by 1 Å AFM - Introduction ◼ The second SPM, developed soon after the STM in 1986, is the Atomic Force Microscope (AFM) ◼ AFM tip 2 long and < 100Å in dia located at the free end of a cantilever that is 100 to 200µm long gently touches the surface ◼ AFM measures contours of constant attractive or repulsive force ◼ The force is an interatomic force (van der Waals force) ◼ Can also be used on non-conductive materials AFM Working Principle ◼ Due to the topography of the probed sample and/or due to attractive or repulsive forces between the sample surface and the tip, the cantilever is bent up and down during scanning ◼ The bending motion of the cantilever is detected by a laser beam reflected from the cantilever to a position-sensitive light detector ◼ The detection is made so sensitive that the forces that can be detected can be as small as a few picoN ◼ Forces < 1 nanoN are usually sufficiently low to avoid damage to surface or tip AFM Working Principle ◼ In contact mode, tip lightly touches the sample. AFM measures repulsion forces between tip and sample ◼ As a raster-scan drags the tip over the sample, detection apparatus measures the vertical deflection of the cantilever, which indicates the local sample height. ◼ In noncontact mode, derives topographic images from measuring attractive forces and does not touch the sample ◼ AFMs can achieve a resolution of 10 pm AFM Working Principle laser photodiode cantilever z piezo x cantilever tip y AFM Working Principle Biological Samples AFM tip DNA AFM Tips Three common types of AFM tip. (a) normal tip (3 µm tall); (b) supertip; (C) Ultralever (also 3 µm tall) Seeing the Nano World (SEM) Seeing the Nano World (SEM) ◼ Because visible light has wavelengths that are hundreds of nanometers long we can not use optical microscopes to see into the nano world. Atoms are like boats on a sea compared to light waves. Seeing the Nano World (SEM) • (A) shows a hand holding a computer chip. This is shown magnified 100 times in (B). Another factor of 100 magnification (C) shows a living cell placed on the chip to show scale. • Yet another factor of 100 magnification (D) shows two nanocomputers beside the cell. The smaller (shown as block) has roughly the same power as the chip seen in the first view; the larger (with only the corner visible) is as powerful as mid-1980s mainframe computer. • Another factor of 100 magnification (E) shows an irregular protein from the cell on the lower right, and a cylindrical gear made by molecular manufacturing at top left. • Taking a smaller factor of 10 jump, (F) shows two atoms in the protein, with electron clouds represented by stippling. A final factor of 100 magnification (G) reveals the nucleus of the atom as a tiny speck. SEM - Introduction ◼ The first electron microscope was produced by Max Knoll and Ernst Ruska in the 1930’s. ◼ There are many types of electron microscopes. These include ◼ TEM transmission electron microscope ◼ SEM scanning electron microscope ◼ Enhanced depth of field ◼ Much higher resolution (up to 10-6 m) Scanning Electron Microscope ◼ In SEM, electrons are accelerated in a vacuum until their wavelength is extremely short. The higher the voltage the shorter the wavelengths ◼ Beams of fast-moving electrons are focused on an object and are absorbed or scattered by the object so as to form an image on an electron-sensitive photographic plate ◼ SEM samples have to be electrically conductive and not produce vapors in a vacuum SEM - Overview ◼ Topography ◼ The surface features of an object or "how it looks", its texture. ◼ Morphology ◼ The shape, size and arrangement of the particles making up the object that are lying on the sample surface ◼ Composition ◼ ◼ The elements and compounds the sample is composed of. Crystallographic Information ◼ The arrangement of atoms in the specimen SEM Working Principle http://mse.iastate.edu/microscopy SEM Working Principle http://mse.iastate.edu/microscopy/beaminteractions.html TEM Working Principle ◼ The first TEM was invented in Germany in 1931. It was the first microscope that could resolve the interior structure of a cell with over 10,000 X magnification. ◼ TEMs can be used to look at the morphology of the sample on the atomic scale, the crystalline structure of the sample, and in some cases the elemental composition of the sample. TEM Working Principle ◼ TEM works like a slide projector. Focused electron beam is send on a very thin specimen; the specimen deflects and scatters the beam which is projected on a screen ◼ Requires stringent preparation sample to be thin for electrons to pass through and the sample must withstand high energy electrons & a strong vacuum SEM Applications ◼ Imaging of microscopic scale objects in high resolution ◼ Medical: compare healthy and unhealthy cells. ◼ Forensics: compare hair, fibers,, paint, inks, etc. ◼ Metals: structural strength. ◼ Scientific Research: cells, nanotubes, metals. Etc. SEM Applications ◼ Imaging individual atoms. ◼ Imaging of surface materials. ◼ Imaging of Nanotubes. Surface Measurement Methods Non-Contact surface Measurement* Non optical methods Scanning Tunneling Microscopy Optical methods Focus sensing techniques s Atomic force Microscopy Scanning Electron Microscopy Interferometric techniques s Scanning Capacitance Microscopy *Not Exhaustive Surface Measurement Optical Methods ◼ light is refracted (bent) when passing from one medium to another ◼ refractive index ◼ a measure of how greatly a substance slows the velocity of light ◼ direction and magnitude of bending is determined by the refractive indexes of the two media forming the interface Surface Measurement Optical Methods ◼ Lenses focus light rays at focal point ◼ distance between center of lens and focal point is Focal Length FL ◼ shorter focal length more magnification ◼ In microscopes, a magnified image is formed by action of 2 lenses The Microscope ◼ Many types ◼ bright-field microscope; dark-field microscope ◼ phase-contrast microscope; fluorescence microscopes ◼ Ability of a lens to separate or distinguish small objects that are close together ◼ Wavelength of light used is major factor in resolution ◼ shorter wavelength greater resolution ◼ Distance between the front surface of lens and surface of cover glass or specimen is the working distance The Light Microscope Confocal Microscopy ◼ Ordinary light microscope produces 2-D superposition of in-focus and out-of-focus regions ◼ Tracing only focused parts of photographs at different focus settings difficult for specimens lacking clear borderlines ◼ Slicing specimen into large number of thin sections is time-consuming and may deform the specimen; cannot study living specimens this way and alignment of images of different slices requires considerable computer processing ◼ In Confocal microscopy, a Laser beam illuminates spots on specimen ◼ Computer compiles images created from each point to generate a 3dimensional image Optical Principles ◼ Specimen illuminated one point at a time ◼ Detector only registers light from illuminated point ◼ Resolution limit is improved ◼ Very pronounced depth discrimination is obtained ◼ Can study different depth layers much more clearly (no out-of-focus information in image) and can study surface structures Why Confocal Microscopy? ◼ 3-D MICROSCOPY! ◼ Scan a number of confocal images, refocusing between successive images ◼ Stack resulting images to produce 3-D structure ◼ No need for alignment processing ◼ Can record sections of live specimens ◼ Can make projections of image to view from different angles Feasibility Studies To successfully perform confocal microscopy, specimen must be reasonably transparent to allow light to penetrate to regions below the surface of the specimen Carlsson and Åslund selected three different applications for their paper: two physiological and one botanical Feasibility Studies Studied various neurons in spinal cord of lamprey Used fluorescent confocal microscopy by staining cells with a dye Obtained good understanding of 3-D structure of neurons by displaying projections through recorded volume in rapid succession One difficulty in studying neurons is the specimen thickness (100s of μm): makes it impossible to scan deepest part of specimen using objective with large N.A. Feasibility Studies Studied ovule of Neuwiedia (an orchid) Fragility of ovules limits physical slicing to 8μm; confocal microscopy allows specimen to remain intact and take ~1μm slices Staining unnecessary due to autofluorescence from ovule wall and nuclei Viewing sections more useful in this case Digitization of data facilitates measurements of 3-D positions of nuclei, size of ovule, etc. Feasibility Studies Studied lung tissue from rat Were able to avoid physical sectioning of specimen through confocal microscopy Used data from individual sections directly for evaluation Staining tissue before embedding in epoxy makes registering sections with high contrast possible Stack of such sections allows measurement of interesting parameters concerning 3-D structure of lung tissue Conclusions Feasibility studies show usefulness of confocal microscope scanning as tool in 3-D microscopy Digitization of recorded volume data allows direct access for computer processing, evaluation, and display Fluorescent specimens are often well-suited for 3-D scanning Objectives with large N.A. should be used, but small working distance of such objectives can create problems MECH 6491 Engineering Metrology and Measurement Systems Lecture 3 Instructor: N R Sivakumar Outline ◼ Tolerancing and interchangeability ◼ Limits and tolerances ◼ Tolerance representation ◼ Tolerance stackup ◼ Theory and applications of tolerancing techniques in precision machining ◼ Tolerance analysis, and synthesis Why Use GD&T? ◼ First and foremost: IT SAVES MONEY! ◼ Provides maximum production tolerances ◼ Helps achieve design intent ◼ Ensures interchangeability of mating parts ◼ Provides uniformity of specification and interpretation – reducing guesswork & controversy ◼ Maximizes quality 99% Quality Means… ◼ 20,000 lost pieces of mail per hour ◼ Unsafe drinking water 15 minutes per day ◼ 5,000 incorrect surgical procedures per week ◼ Two short or long landings at most major airports per day ◼ 200,000 wrong drug prescriptions per year ◼ No electricity for 7 hours per month Tolerance Definition “The total amount by which a given dimension may vary, or the difference between the limits” - ANSI Y14.5M-1982(R1988) Standard [R1.4] Tolerance Types ◼ Linear Tolerance ◼ ◼ Size Geometric Tolerance ◼ Form ◼ Profile ◼ Location ◼ Orientation ◼ Runout Tolerance vs. Mfg Process ◼ Nominal tolerances for steel ◼ Tighter tolerances => increase cost $ Limits and tolerances Key Concepts Actual Size - is the measured size Basic Size - of a dimension is the theoretical size from which the limits for that dimension are derived by the application of the allowance and tolerance. Design Size - refers to the size from which the limits of size are derived by the application of tolerances. Limits of Size - are the maximum and minimum sizes permissible for a specific dimension. Nominal Size - is the designation used for the purpose of general identification. Limits and tolerances Key Concepts Tolerance - The tolerance on a dimension is the total permissible variation in the size of a dimension. The tolerance is the difference between the limits of size. Bilateral Tolerance - With bilateral tolerance, variation is permitted in both directions from the specified dimension. Unilateral Tolerance - With unilateral tolerance, variation is permitted in only one direction from the specified dimension. Maximum Material Size - The maximum material size is the limit of size of a feature that results in the part containing the maximum amount of material. Thus it is the maximum limit of size for a shaft or an external feature, or the minimum limit of size for a hole or internal feature. Limits and tolerances Terminology Representing Linear Tolerance ◼ General tolerance ◼ Note: all decimals ±.02 ◼ Limit dimensions ◼ Plus and minus dimensions ◼ unilateral ◼ bilateral Fits and Allowances Fits The fit between two mating parts is the relationship between them with respect to the amount of clearance or interference present when they are assembled. There are three basic types of fits: clearance, interference, and transition. Clearance Fit - A fit between mating parts having limits of size so prescribed that a clearance always results in assembly. Interference Fit - A fit between mating parts having limits of size so prescribed that an interference always results in assembly. Determining Fits Fits and Allowances Allowance -intentional difference between the maximum material limits of mating parts. Basic Size The size to which limits or deviations are assigned. - same for both members of a fit. Deviation The difference between a size and corresponding basic size. Upper Deviation maximum limit of size - basic size. Lower Deviation minimum limit of size - basic size. Tolerance maximum size minimum size on a part. And the zone representing this is the Tolerance Zone Fundamental Deviation The deviation closest to the basic size. Fits and Allowances Description of Fits (Values given in Handout) Running and Sliding Fits - A special type of clearance fit. These are intended to provide a similar running performance, with suitable lubrication allowance, throughout the range of sizes. Locational Fits - They may provide rigid or accurate location, as with interference fits, or some freedom of location, as with clearance fits. Accordingly, they are divided into three groups: clearance fits, transition fits, and interference fits. Locational clearance fits - are intended for parts that are normally stationary but that can be freely assembled or disassembled. Locational transition fits - are a compromise between clearance and interference fits when accuracy of location is important but a small amount of either clearance or interference is permissible. Locational interference fits - are used when accuracy of location is of prime importance and for parts requiring rigidity and alignment with no special requirements for bore pressure. Fits and Allowances Description of Fits Drive and force fits It constitute a special type of interference fit, normally characterized by maintenance of constant bore pressures throughout the range of sizes. Standard inch Fits RC LC LT LN FN Running and sliding fit Locational clearance fit Locational transition fit Locational interference fit Force or shrink fit Hole Basis System ◼ Smallest possible hole (MMC) is the basic size ◼ Hole tolerance added to basic size for largest hole ◼ Allowance subtracted from basic size for largest shaft ◼ Tolerance of shaft subtracted from largest shaft for smallest shaft Example - Hole Basis System 1.994 1.991 2.000 2.004 SHAFT HOLE Nominal size - 2” Basic size - Hole tolerance - Shaft tolerance - .003” Allowance - 2.000” .004” .006” Fits and Allowances Basic Hole System In the basic hole system, which is recommended for general use, the basic size will be the design size for the hole, and the tolerance will be plus. The design size for the shaft will be the basic size minus the minimum clearance, or plus the maximum interference (Refer Handout) +.0020 +.0000 Hole Ø 1.0000 -.0000 Shaft Ø .9975 -.0012 Basic Shaft System Fits are sometimes required on a basic shaft system, especially when two or more fits are required on the same shaft. +.0020 Hole Ø 1.0025 -.0000 +.0000 Shaft Ø 1.0000 -.0012 Fits and Allowances Preferred Metric Limits and Fits Hole Basis System Metric Fits Fits and Allowances Metric Tolerance symbol Fits and Allowances Preferred Metric fits Fits and Allowances Example Surface Texture Surface texture characterisitcs Roughness - Roughness consists of the finer irregularities in the surface texture, usually including those that result from the inherent action of the production process. These include traverse feed marks and other irregularities within the limits of the roughness-width cutoff. Roughness-Height Value - Roughness-height value is rated as the arithmetic average (AA) deviation expressed in micro inches or micrometers measured normal to the center line. The ISO and many European countries use the term CLA (center line average) in lieu of AA. Both have the same meaning. Roughness Spacing - Roughness spacing is the distance parallel to the nominal surface between successive peaks or ridges that constitute the predominant pattern of the roughness. Roughness spacing is rated in inches or millimeters. Surface Texture Surface texture characterisitcs Roughness Spacing - Roughness spacing is the distance parallel to the nominal surface between successive peaks or ridges that constitute the predominant pattern of the roughness. Roughness spacing is rated in inches or millimeters. Roughness-Width Cutoff - The greatest spacing of repetitive surface irregularities is included in the measurement of average roughness height. Roughness-width cutoff is rated in inches or millimeters and must always be greater than the roughness width in order to obtain the total roughness-height rating. Waviness - Waviness is usually the most widely spaced of the surface texture components and normally is wider than the roughness-width cutoff. Lay - The direction of the predominant surface pattern, ordinarily determined by the production method used, is the lay. Flaws - Flaws are irregularities that occur at one place or at relatively infrequent or widely varying intervals in a surface. 0 Surface Texture Surface texture symbols Roughness range for common production methods Typical surface Roughness height applications Tolerance Types ◼ Linear Tolerance ◼ ◼ Size Geometric Tolerance ◼ Form ◼ Profile ◼ Location ◼ Orientation ◼ Runout Reason for GD & T Geometric tolerancing Rationale • The tolerances and limits of size that are seen so far do not specifically control other variations – form, orientation, and position to some extent • To meet functional requirement, geometric tolerances need to be added to control limits of size, but also to ensure that limits of form orientation and position are maintained • A geometric tolerance is the maximum permissible variation of form, profile, orientation, location, and runout from that indicated or specified on a drawing. • The tolerance value represents the width or diameter of the tolerance one within which the point, line or surface or the feature must lie. Geometric tolerancing Points line and surfaces • Engineering surfaces may be flat, cylindrical conical etc or some irregular shape • But line or surface is evaluated dimensionally by making measurements at various points along the length • Geometric tolerances are concerned with points and lines, and surfaces are considered as series of lines in 2 or more directions • Points have only position and no size, so position is the only thing requiring control. • But lines must be controlled for from, orientation and location Geometric Tolerances Notes Vs. Symbols Material Condition ◼ ◼ ◼ Maximum Material Condition (MMC) M ◼ Largest possible external feature (e.g. shaft) ◼ Smallest possible internal feature (e.g. hole) Least Material Condition (LMC) ◼ Smallest possible external feature ◼ Largest possible internal feature L Regardless of Feature Size (RFS) ◼ Default condition if not specified Material Condition Applicability of RFS, MMC and LMC • If freedom of assembly in the mating parts is the key, MMC is leas preferred • If in fig MMC is applied, both the pin and hole will be at .250in • Theoretically, this will assemble provided there are no variations in form or orientation – and a perfect centre distance of 2in • If the same is in LMC they are .247 and .253 this gives more play while assembly. Like the centre distance can be varied slightly or there can be form tolerances and orientation tolerance 5 Categories of Control Design Specs and Tolerance ◼ Develop from quest for production quality and efficiency ◼ Early tolerances support design’s basic function ◼ Mass production brought interchangeability ◼ Integrate design and manufacturing tolerances Affected Areas Engineering Tolerance Product Design Quality Control Manufacturing • Product Design - to design with consideration for the maximum possible working tolerances compatible with the functional design requirements. • Manufacturing - to bringing the design into a physical entity with consideration for