Lecture 1 Physiology: Introduction & Body Fluid Compartments PDF
Document Details
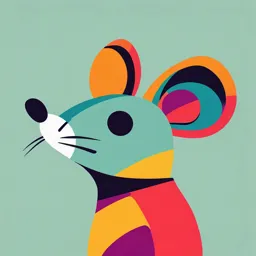
Uploaded by GreatJasper8017
Faculty of Science
Tags
Summary
This document introduces human physiology and discusses body fluid compartments. It details the composition of body fluids, including intracellular and extracellular components, and explains their roles in maintaining human life. It also explores examples of different body systems.
Full Transcript
Lecture 1 physiology INTRODUCTION TO HUMAN PHYSIOLGY BODY FLUID COMPARTMENTS Definition of Human Physiology: Physiology is a Latin word divided into 2 parts: Physio = means Function. Logy = means Science. So, Human Physiology is the science that deals with the fun...
Lecture 1 physiology INTRODUCTION TO HUMAN PHYSIOLGY BODY FLUID COMPARTMENTS Definition of Human Physiology: Physiology is a Latin word divided into 2 parts: Physio = means Function. Logy = means Science. So, Human Physiology is the science that deals with the functions of the human body under different environmental conditions (internal or external) in order to maintain life. Human Body Organization: The cell is the smallest basic structural unit of the human body. A tissue is formed of a group of cells arranged side-by side & having the same shape, structure and function. An organ consists of more than one tissue and performs a specific function; e.g. the teeth masticates and the heart beats to pump the blood. A system consists of more than one organ performing complementary functions, all of which determine the characteristic role of that system in life. Thus, our bodies consist of a group of systems; each of them plays a specific role in our body and the sum of these roles altogether are essential to keep our lives. Examples of Different Body Systems: * The Cardiovascular System * The Endocrine System. * The Respiratory System. * The Nervous System. * The Urinary System. * The Reproductive System. * The Digestive System * The Musculoskeletal system. CHEMICALLY, Human Body is composed of: Water (60%). Solids (40%); either; a. Organic (protein 18% - fat 15%). b. Inorganic (minerals 7%). BODY FLUIDS: The total body water is normally about 60% of the body weight in young adult males. Therefore, in a young adult male weighing 70 Kg, the total body water is normally about 42 litres, and it is distributed as follows: (1) Intracellular fluid (ICF): it represents about 2/3 of the total body water i.e. about 40% of the body weight (~28 L). (2) Extracellular fluid (ECF): it represents about 1/3 of the total body water i.e. about 20% of the body weight (~ 14 L). It includes the following subdivisions: a) Intravascular fluid (i.e. blood plasma): This is normally about 1/4 of the ECF volume. b) Extravascular fluid: This is normally about 3/4 of the ECF volume. It includes the interstitial fluid + transcellular fluids. N.B. Interstitial or intercellular or tissue fluid): the fluid in spaces in between tissue cells. Transcellular fluids: the fluid present in closed spaces surrounded by epithelium. e.g. cerebrospinal fluid, intraocular fluids, and the fluids in the pleura, joints, peritoneum, etc. Calculate the total body water of a 40 years adult male, 70 Kg: Total Body Water = 60/100 * 70 = 42 litres How many litres lie intracellularly? 42 X ⅔ = 28L OR 70 X 40/100 = 28L. How many litres lie extracellularly? 42 X ⅓ = 14L OR 70 X 20/100 = 14L. Composition of ECF vs. ICF: Item ECF ICF Mainly Na+ (142 mEq/L) Mainly K+ (140 mEq/L) & K (4 mEq/L) Mg2+ Main cation + small amounts of Ca2+ and small amounts of Na Mg2+ very little Ca2+ Cl- HPO4 & protein. -, Main anion small amounts of: HCO-3, Small amounts of Cl HCO3 proteins, and HPO4 and SO4 pH About 7.4 About 7 due to low HCO-3 Osmolarity The same The same Functions of Body Water: 1. It is the medium for chemical and enzymatic reactions. 2. It is the medium for the physical processes e.g. diffusion and filtration. 3. It is an ionizing medium (in regulating pH and body fluid osmolarity). 4. Regulation of body temperature. 5. It is a lubricant in the joints and potential spaces (e.g. the pleura). 6. It is a refractive medium in the eye. 7. The cerebrospinal fluid is a mechanical buffer that protects the brain. 8. It is the medium for gas exchange (O2 & CO2) in lungs and tissues. *************************************************** Human Cell; Cell Membrane Structure & Function The cell is the Building (Structural) Unit of the human body. THE CELL MEMBRANE: Functions: 1. It forms the outer boundary surrounding the cell to protect it from the external environment. 2. Selective permeability; as it permits the passage of certain substances and prevents others. 3. Very thin; 75 angstroms. Chemical Structure: It is formed of lipid (phospholipid(flexibility and cholesterol tufness), Proteins and carbohydrates a. Phospholipids: - Each phospholipid molecule in the cell membrane is formed of: 1. Water soluble (hydrophilic) phosphate part (head). 2. Fat soluble (hydrophobic) lipid part (tail) containing cholesterol. - The plasma membrane is formed of two layers (bilayer) of phospholipid molecules with their hydrophilic phosphate heads directed outwards and inwards and their hydrophobic lipid tails directed to the interior of the membrane. b. Proteins: Proteins determine the cell membrane’s specific functions more active membrane= more ptn - Cell membrane proteins are formed of peptide chains of amino acids. - Chemically, they are either; 1. Pure proteins. 2. Conjugated proteins with carbohydrates (glycoproteins) or with lipids (lipoproteins). - According to their site; Proteins are either; 1. Peripheral proteins on the outer or inner surfaces. ex: cell surface identity marker (antigens especialy glycoptn carbohydrate), receptors (out side) or enzyme from (inside) 2. Through & through proteins (i.e. transmembrane or integral proteins). Channel , carrier and pump Chemical Structure of Cell Membrane. Functions of Cell Membrane Proteins: 1. Surface proteins act as receptors or surface recognition sites (self-antigens ) Carbohydrate part→ very important for immune system to differentiate between what is self and non-self (foreign) → thus preventing the body from attacking itself (i.e. autoimmune diseases). 2. Act as active Pumps e.g. Na+-K+ pump. 3. Act as Receptors for; Hormones. Chemical transmitters. 4. Act as Enzymes e.g. adenylate cyclase enzyme → which catalyses the formation of cyclic AMP (cyclic AMP) from ATP. 5. Act as Carriers; helping transport of substances through the cell membrane. Types of Carriers: a) Uniport: transport one substance in one direction. b) Symport: cotransport more than one substance at a time in only one direction. c) Antiport: transport one substance in one direction in exchange for another in opposite direction e.g. Na+-K+ pump carrier → it transports 3 Na+ out of the cell in exchange with 2 K+ into the cell. Types of cell membrane carrier proteins. 6. Act as Channels: through which water soluble substances can pass through the cell membrane. Types of Cell Membrane Channels: 1. Non gated channels: they are channels that are open all the time allowing passage of ions all the time. Sometimes they are called “leak channels”. 2. Gated channels: these channels are classified into: a. Voltage gated channels: they open or close in response to changes in membrane potential. b. Ligand gated channels: they open or close in response to binding to a chemical substance called (ligand), which may be either: External ligands; binds to the outer surface of the cell membrane as neurotransmitters and hormones. Internal ligands; binds to the inner surface of the cell membrane as Ca+2 and cyclic AMP. c. Mechanical gated channels: they open or close in response to mechanical stretch or pressure. Types of cell membrane channels PHYSIOLOGY; LECTURE 2 Transport Mechanisms through Cell Membrane Either I. Passive II. Active i.e. No need for energy i.e. need energy Passive transport I_-Diffusion Definition: It is the free movement of substance molecules through the cell membrane caused by their kinetic energy. Principles: All molecules of solution or of gases possess kinetic energy and can diffuse in either direction. The rate of diffusion is directly proportional to: 1. Concentration difference of the substance across the cell membrane. 2. Temperature. 3. The surface area of the membrane. The rate of diffusion is inversely proportional to: 1. The thickness of the membrane. 2. The square root of molecular weight. √ N.B. Fat soluble substances as O2 and CO2 …….. etc diffuse through the phospholipid layer of the cell membrane. The greater the lipid solubility of the substances, the greater the rate of diffusion. 1 Water is the ONLY hydrophilic lipophobic substance that can diffuse through phospholipid layer of the cell membrane due to its smaller molecular size. Figure: Simple diffusion Simple diffusion through integral membrane proteins Channels: - These are pathways (i.e. pores) for substances that are NOT lipid soluble. - Water and water-soluble substances e.g. ions diffuse through transmembrane protein channels on condition that: a. The molecular size of the diffusing molecule is less than that of the channel. b. The electric charge allows diffusion as follows: The charge lining the channels. The rule is “opposite charges attract and enhance while similar charges repel and oppose diffusion. c. The greater the hydration energy of the molecule, the thicker is the water jacket around and the slower is the diffusion rate. II-Facilitated diffusion: It occurs when the diffusing substance cannot pass by simple diffusion through the phospholipid layer due to: a) It is not lipid soluble. b) It is water soluble, but cannot diffuse through the channel, Because: It has a molecular size larger than the pore size; e.g. all sugars as Glucose 2 Membrane proteins that facilitate transport of a substance that has a larger molecular size than the pore size, or NOT electrically fit to pass through channels, e.g. all sugars as Glucose diffuse by carriers. Mechanism: The substance (S) combines with a transmembrane protein carrier (C) on one surface forming → a C-S complex → conformational change → C-S complex changes position to the opposite surface and the substance leaves the carrier conformational change of the carrier again to return to original conformation to bind another molecule of (S) and the process is repeated. Properties of Facilitated Diffusion: NO energy (ATP) is required (i.e. passive). Same principles for simple diffusion are applied. The presence of the carrier is essential and, in its absence, facilitated diffusion will never occur. III-Osmosis: It's the free movement of the solvent molecules through a semipermeable membrane from the compartment of lower concentration to the compartment of higher concentration of the solution. 3 Or it’s the diffusion of water through a semi- permeable membrane from an area of high conc. of water to an area of low conc. of water. It is a passive mechanism (no energy needed) For only solvent molecules (e.g. water) It is an absorbing force. It depends on the number of particles in the solution rather than its concentration. For osmosis to occur: 1. The membrane must be permeable to water and impermeable to at least one of the solutes in the solution (i.e. semipermeable) 2. There must be a difference in solute conc. between the two sides of the membrane. N.B. * Osmole is the number of particles present in one mole of undissociated substance. * Mole is the molecular weight of a substance in grams. * So, for any substance: If not dissociated; 1 osmole = 1 mole If dissociated; No of osmoles = 1 mole of substance X number of particles in the solution Ex. 1 mole of glucose (1 particle if dissociated) = 1 X 1 particle = 1 osmole But, 1 mole of NaCl = 1 x 2 particles (if dissociated) = 2 osmoles Na+ Cl- (2 particles) The osmotic pressure: - It is the pressure required to stop osmosis. - It depends on number of particles not size of molecules 4 Normal plasma osmolarity = ~ 290 mosmol/L. Factors affecting plasma osmolarity: 1. Plasma Na+ conc. (~ 142 mEq/L). 2. Plasma glucose conc. (~ 126 mg %). 3. Plasma urea conc. (~ 20 - 40 mg %). 4. Plasma protein conc. (~ 7 gm %). Plasma osmolarity can be calculated from this equation; Plasma osmolarity = 2 (Na+) + 0.055 (glucose) + 0.36 (urea) mosmole/L mEq/L mg % mg% N.B. Tonicity is the osmolarity of the solution relative to that of plasma, Solutions compared to Plasma Osmolarity are 1. Isotonic 2. Hypertonic 3. Hypotonic Tonicity is equal to Tonicity is more than Tonicity is less than that of plasma that of plasma that of plasma osmolarity. osmolarity. osmolarity. They are the only If given i.v. -------- it If given i.v. ------ it solutions allowed to causes Shift of water causes Shift of water be given to the patient from cells into the into the cells leading parenterally (i.v.) blood leading to: to: Cell swelling or Ex. Cell shrinkage edema and cell or (crenation) 1. Glucose 5% rupture 2. NaCl 0.9% = (saline) 5 VI-Filtration: Definition; It means forcing a fluid to pass through a semipermeable membrane by creating a pressure difference (gradient). It is a passive mechanism (no energy needed) It is a filtrating force. It depends on creating a pressure gradient through a semipermeable membrane (direct relationship); i.e. the higher the pressure gradient, the greater is the rate of filtration. Importance: 1. Filtration of plasma to form tissue fluid. 2. Filtration of plasma in the kidney urine formation. 2-Active Transport: Characters: Transport against electric or concentration gradients. Active (i.e. requires energy either direct or indirect). Requires a carrier protein. Types of Active Transport: 2 types 1. Primary Active Transport: Energy is supplied directly from ATP. ATP → ADP + Pi + Energy Example: Sodium-Potassium (Na+-K+) pump: - It is present in ALL cell membranes. - Transport 3 Na+ out and 2K+ in. - Calcium ATPase pump - Hydrogen ATPase pump 6 2. Secondary Active Transport: It is a transport of one or more solutes against an electrochemical gradient, coupled to the transport of another solute down an electrochemical gradient. Types of secondary active transport: a. 2ry Co-transport (i.e. symport): All solutes move in the same direction “inside cell” Examples: Na+ – glucose Co-transport Na+ – amino acid Co-transport. b. 2ry Counter transport (i.e. antiport): Na+ is moving to the interior causing other substance to move out. Examples: Ca²+ – Na+ exchange. Na+ – H+ exchange in the kidney. Mechanism of secondary active transport: In the figure below: 1. Na+ is actively pumped out of the cell by Na+-K+ pump creating a concentration gradient for Na+ (high outside and low inside the cell). 2. A cotransport (or antiport) carrier binds both Na+ and substance X or substance Y. 7 3. Na+ moves from outside to inside according to its concentration gradient, while both of substance X and substance Y move against their concentration gradient (from outside to inside for substance X and from inside to outside for substance Y). 4. The transport of both X and Y is called secondary active transport because it occurs against concentration gradient making use of the energy derived from the concentration gradient of Na+ that is created by the active Na+- K+ pump. Figure: Secondary active transport I. Bulk transport:. 8 PHYSIOLOGY OF THE NERVE LECTURE (1) The Nerve Cell (Neuron): The nerve cell (neuron) is the structural unit of the nervous system. STRUCTURE OF THE NEURON: Each neuron is formed of 2 main parts: 1. The cell body (Soma). 2. The cell processes. 1. THE CELL BODY: It contains a large central nucleus with a well-marked nucleolus. The cytoplasm contains: a. Neurofibrils: delicate strands that pass the cell body and processes. b. Nissel Bodies: large triangular masses with high RNA content. They are absent from the axon hillock. Severe activity and anoxia cause disappearance of Nissel granules (Chromatolysis). c. Other organelles as in other cells (as Golgi apparatus, ER…etc). The neuron doesn't contain a centrosome and can never divide. 2. THE CELL PROCESSES: Are of 2 types; dendrites & axon. A. Dendrites: Which are short processes that extend out from the cell body and branch extensively. Functions: 1. Increase the surface area of the cell body (i.e., receptive field of neuron). 2. Conduct the nerve impulse towards the cell body. B. Axon: Which is a single long process that originates from a thickened area of the cell body called the axon hillock. Functions: 1. Conduct the nerve impulse away from the cell body and the dendritic zone. 2. The axon ends in a number of synaptic terminal buttons or axon telodendria. They contain vesicles filled with the chemical transmitter (e.g., Acetylcholine). The axons are called the nerve fibres. Figure 1: Structure of the nerve cell The axon is covered by: 1. The myelin sheath: it is a protein-lipid complex and act as an electric insulator. The myelin sheath is interrupted at nodes of Ranvier. 2. An outer nucleated layer called neurilemmal sheath or Schwann sheath. It regenerates the nerve fibre when it is cut and forms myelin sheath. It is absent in neurons of the CNS (the myelin sheath in CNS is formed by another type of cells called oligodendrocytes). According to myelin sheath, nerve fibres are classified into: A fibres (thick myelinated, ν of conduction ≈ 100 m/s) B fibres (thin myelinated, ν of conduction ≈ 10 m/s) C fibres (non-myelinated, ν of conduction ≈ 1 m/s) Figure 2: Myelin & Schwann sheathes Excitability: It is the ability of any living tissue to respond to a stimulus. It is a property of life. The nerve is one of the most excitable tissues. A Stimulus: It is any change in the environment surrounding a living tissue that causes it to react. Types of stimuli: Electrical – Chemical – Mechanical - Thermal. Electrical stimulus is preferred in practical experiments because: 1. They are similar to natural signals inside the body. 2. Their intensity and duration of application can be easily controlled. 3. They produce response without causing damage (i.e. They are reproducible or can be repeated) Factors affecting the degree of response to a particular stimulus: 1. The rate of application. 2. The strength of the stimulus 3. The duration of application. 1. Effect of the rate of application: A suddenly applied stimulus of certain intensity is more effective than when a weaker stimulus is applied and then gradually increased to the same intensity as the previous one. 2. Effect of the strength of the stimulus and its duration of application: This is best illustrated by the Strength-Duration (SD) Curve. Figure 3: The Strength Duration (SD) Curve From the curve above, it is concluded that: The stronger is the stimulus, the shorter is the time needed to excite and vice versa. There is a minimal or threshold intensity called (Rheobase, R) which can stimulate. It is the minimal intensity of a current of a very long duration which can stimulate or, it is the minimal galvanic current which can stimulate. The Utilization Time (UT): it is the time needed by the Rheobase (R, Threshold) to stimulate. The Chronaxie (C): It is the time needed by a current of double the rheobase (2 R or 2 threshold) intensity to stimulate. Significance: It is used to compare excitability of different tissues. The shorter the chronaxie, the greater is the excitability. (Excitability of the Nerve ˃ Skeletal m. ˃ Cardiac m. ˃ Smooth m.) The Minimal Duration (t): it is minimal time needed for a stimulus to produce a response. If the duration of application is less than (t), no response whatever the strength of the stimulus is. Significance: In diathermy, the use of high voltage alternating currents for a very short period of time less than (t) during each phase causes rapid oscillation of ions leading to heating of tissues without stimulation. This is used in electrocautery to stop bleeding. _____________________________________________________________ N.B.: Cathode Ray Oscilloscope (CRO); is used to record the electrical activity of the neuron. It registers rapid events measured in milliseconds (ms) and rapid changes in potential in millivolts (mV). Microelectrodes: Very fine glass capillary pipettes with pointed tips and filled with KCl (as electric conductor). Figure 4: Cathode Ray Oscilloscope THE RESTING MEMBRANE POTENTIAL (RMP): Definition: It is the potential difference between the inner and outer sides of the membrane under resting conditions. It is -70 mV (with the negativity inside). Causes of RMP: 1. Selective permeability of the membrane. 2. The Na+ - K+ pump. I. Selective Permeability of the membrane: The resting membrane is 50-100 times more permeable to K+ than Na+. This is due to: 1. The membrane Na+ channels are closed under resting conditions, while the K+ channels are opened. 2. The Na+ channels are guarded by Ca2+ from outside. 3. The hydration energy for Na+ is greater than that for K+. Thus, the size of hydrated Na+ ion is greater than that of K+ (i.e., the hydration energy is inversely proportionate with the atomic number). II. The Na+- K+ Pump: It is an electrogenic pump. It transmits 3 Na+ ions to the exterior for each 2 K+ ions transmitted to the interior. Thus, creating negativity (-) inside and positivity (+) outside the nerve membrane. The pump is an active process (i.e., it requires ATP and Na+- K+ ATPase). It is stimulated by either excess Na+ or excess ATP inside the cell, and excess K+ outside the cell. It is inhibited by decreased temperature (i.e., cooling) or by a drug called ouabain (a drug used in heart failure). PHYSIOLOGY OF THE NERVE LECTURE (2) The Nerve Impulse: It is a physicochemical disturbance produced by a stimulus of threshold intensity or more, and is propagated in the form of a wave along the nerve fiber. It is accompanied by: 1. Electric changes. 2. Excitability changes. 3. Metabolic changes. 4. Thermal changes. The Action Potential (AP): Definition It is a series of electric changes that accompanying the nerve impulse. It is formed of: 1. Latent Period (LP). 2. Spike Potential (SP). 3. After Potentials. The Monophasic Action Potential (MAP) It is recorded by means of two recording microelectrodes (one inside and the other outside the axon of a neuron) connected to a CRO and placed at a distance from the stimulating electrodes. The following electric changes (phases) are recorded: 1. The Stimulus Artifact (SA): It is a short period of irregular deflection of the baseline of CRO at the moment of application of the stimulus. Cause: It is due to current leakage (i.e., electrons) from the stimulating electrodes to the recording electrodes along the extracellular fluid. 2. The Latent Period (LP): It is an isopotential period (baseline) following the stimulus artifact. Significance: a) It corresponds to the time (t) of propagation of the impulse from the stimulating electrode to the recording electrode through the nerve. b) If this time (t) is known and the distance (d) between the stimulating and recording electrodes is known, the velocity (v) of conduction along the nerve fiber can be calculated from the following; ν = d/t 3. The Spike Potential & After Potentials: The first electric change that accompanies a nerve impulse is a gradual decrease in membrane potential from -70 mV to -55 mV (i.e., DEPOLARIZATION). At membrane potential -55 mV, the rate of depolarization increases rapidly. The firing level (FL): is the membrane potential at which the rate of depolarization changes from gradual to rapid. It is -55 mV in the nerve. After reaching the FL, the membrane rapidly depolarizes to reach ZERO POTENTIAL (ISOPOTENTIAL) and continues to overshoot in the positive direction to approximately +35 mV. This is called REVERSAL OF POLARITY. The membrane potential then starts to fall back to the resting level (RMP). This is called REPOLARIZATION. Repolarization is rapid at first (until the membrane is 70% repolarized), then the repolarization becomes gradual when the membrane potential approaches the RMP (i.e., in the last 30% of repolarization). The SPIKE POTENTIAL: is the rapid depolarization followed by the rapid repolarization The NEGATIVE AFTER POTENTIAL (i.e., AFTER DEPOLARIZATION): is the slower repolarization at the last 30% of repolarization. When the RMP is reached, the membrane potential overshoots in the direction of hyperpolarization. This is called AFTER HYPERPOLARIZATION or POSITIVE AFTER POTENTIAL. Figure 1: Monophasic AP IONIC BASIS OF ACTION POTENTIAL: I. Ionic basis of Depolarization: Depolarization in the nerve occurs due to the opening of voltage-gated Na+ channels that start to open at -63 mV but all of them open at -55 mV (i.e., firing level). Opening of Na+ channels → Na+ influx according to electrochemical gradient. The membrane reaches the zero potential (i.e., isopotential) and Na+ continues to diffuse in reversing the polarity (i.e., reversal of polarity). At +35mv: rapid closure of Na+ channels occur (i.e., depolarization stops and repolarization starts … Due to: 1. Decreased membrane permeability to Na+ due to rapid closure of voltage- gated Na+ channels (become inactivated). 2. Reversal of electric gradient for Na+ (i.e., as the inside becomes positive repelling any further entry for Na+. II. Ionic basis of Repolarization: Repolarization occurs due to the maximum opening of voltage-gated K+ channels at +35 mV in addition to the rapid closure of Na+ channels. K+ channels opening → results in K+ outflux according to its electrochemical gradient. This occurs rapidly at first (rapid repolarization) and then becomes prolonged (gradual repolarization) due to delayed closure of K+ channels. K+ outflux restores the +ve charges on the outer surface and brings the membrane potential towards resting level (i.e., RMP). N.B.: K+ channels open slowly. It starts to open just after the opening of Na+ channels but it reaches its maximum opening at reversal of polarity, then remains open for a longer time and closes slowly. III. Ionic basis of After Potentials: a) Negative after potential: It is a gradual repolarization due to delayed outflux of K. b) Positive after potential: It is overshooting in the direction of hyperpolarization due to prolonged K+ outflux. When the membrane reaches the RMP, the Na+- K+ pump will restore the normal ionic distribution to keep the Na+ concentration more outside and the K+ concentration more inside. Figure 2: Phases & Ionic basis of AP. Excitability changes: General Rule: The closer the membrane potential (MP) to the firing level (FL), the greater the excitability of the nerve (i.e. the greater the ability to reach the FL and produce a nerve impulse) Excitability Changes (Phases) during the Action Potential: Figure 3: Excitability Changes (Phases) during the Action Potential 1. Absolute refractory period: Excitability is completely lost. It corresponds to the ascending and early part of the descending limb of the spike. 2. Relative Refractory Period: Excitability is starting to return to normal, but it is still below normal. It corresponds to the last 2/3 of the descending limb of the spike. 3. Supernormal Phase of Excitability: Excitability is greater than normal and subthreshold stimuli can produce a response when applied during this phase. It corresponds to the negative after potential. 4. Subnormal Phase of Excitability: Excitability is less than normal. A stronger stimulus (more than the threshold) is needed to excite. It corresponds to the positive after potential. Factors Affecting the Nerve Excitability: A. Factors that Decrease the Excitability: These factors are called nerve stabilizers. 1. Local anesthetics: e.g., cocaine which decreases the permeability of the nerve membrane to Na+ → decreases the excitability. 2.Effect of ions: High Ca++ concentration in the extracellular fluid → decreases the permeability to Na+ → decreases excitability. Low K+ concentration in the extracellular fluid → increases the RMP (i.e., hyperpolarization) → decreases excitability (e.g., as in a hereditary disease called familial periodic paralysis). 3.Cooling, ↓blood supply, O2 lack, and acidity: ↓ excitability. B. Factors which Increase the Excitability (Any condition that causes depolarization (i.e., membrane potential is closer to the firing level) → the nerve becomes more excitable). 1. Warming & alkalinity → increases excitability. 2. Effect of ions: Low Ca++ concentration in the extracellular fluid →↑ the permeability to Na+ → increases the excitability. High K+ concentration in the extracellular fluid → decrease K+ outflux (by decreasing its conc. gradient) → ↓ the RMP (i.e., depolarization) → increases the excitability. N.B. The active form of calcium is the ionized form. Ionization of calcium is increased in an acidic medium (i.e., ↓pH) and vice versa. Therefore, changes in the pH of ECF affect nerve excitability indirectly by changing the rate of ionization of calcium (which guards the Na+ channels on the nerve fiber). The ALL or NONE Law: It states that: Under constant conditions: When a single nerve fiber is stimulated, either it doesn’t respond at all (if the stimulus is subthreshold), or it responds by a nerve impulse (if the stimulus is threshold or more). The nerve impulse is always of the same magnitude and duration whatever the intensity of the stimulus that produced it". It is obeyed by a single nerve fiber (axon), a single skeletal muscle fiber, and the whole cardiac muscle. Conduction of Nerve Impulse: 1. Conduction in Non-myelinated nerve fiber: When the adequate stimulus is applied, we get a reversal of polarity at the site of stimulation (the outer surface becomes negative) The negative outer surface acts as a cathode for the neighboring segments (which are positive). Positive charges from the membrane in front of and behind the area of reversal of polarity (i.e. current sink) will be drawn into it →↓ the membrane potential (depolarization) in front of and behind the initial segment. If this depolarization is of sufficient magnitude → reversal of polarity at these new sites, the impulse is conducted as a wave of reversal of polarity. 2. Conduction in Myelinated nerve fiber: The myelin sheath is an electric insulator. The adequate stimulus produces a reversal of polarity at the active nodes of Ranvier. The positive charges on the outer surface of the next node of Ranvier jump into the active node of Ranvier and this second node therefore becomes depolarized and activated to stimulate the next and so on. The jumping of depolarization from node to node is called saltatory conduction. Significance of Saltatory Conduction: 1. It is rapid. 2. Consumes less energy (i.e., saves energy). Figure 4: Conduction in myelinated and non-myelinated nerve fibers Orthodromic & Antidromic Conduction: When a nerve fiber is stimulated in the middle, the nerve impulse produced can travel in both directions. The natural conduction inside the body is from the dendrites (receptive field) → cell body → axon → axon terminal where the transmitter is released at the synapse (orthodromic conduction). Conduction in the opposite direction is called antidromic conduction. The impulse stops at the dendrite since there is NO chemical transmitter to be released.