Cell Biology Lecture 1: Cells and Organelles PDF
Document Details
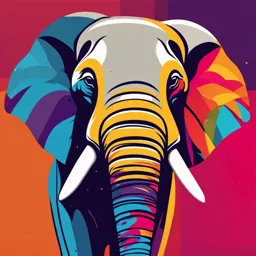
Uploaded by RazorSharpFoil
Tags
Summary
This lecture introduces the fundamental concepts of cell biology, including cell structure, organelles, and the distinction between prokaryotic and eukaryotic cells. It covers the basic properties of cells, the roles of the nucleus, mitochondria, and chloroplasts, and explores carbon fixation. The document aims to provide a foundational understanding of cell biology.
Full Transcript
Biol 266 – Cell Biology UNIT 1 “Cells and Organelles - I” What is “cell biology” anyway? The study of cells and their structure, function and behaviour Requires creative instruments and techniques Reductionist – studying the parts can explain the whole The cell = the fundamental unit of life...
Biol 266 – Cell Biology UNIT 1 “Cells and Organelles - I” What is “cell biology” anyway? The study of cells and their structure, function and behaviour Requires creative instruments and techniques Reductionist – studying the parts can explain the whole The cell = the fundamental unit of life, arises from pre-existing cell Cells are the smallest units exhibiting the characteristics of LIFE they are able to reproduce themselves by their own efforts Growth Division Epithelial cells Growth Division Nervous cells Growth Division Muscle cells Organelles are NOT the smallest units exhibiting the characteristics of life they are NOT able to reproduce themselves by their own efforts outside of the host cell Organelle = specialized structure in a cell that performs a specific function Viruses are NOT the smallest units exhibiting the characteristics of life they are NOT able to reproduce themselves by their own efforts; they use the host reproductive machinery Human Immunodeficiency Virus (HIV) The cell is the fundamental unit of life, the building block from which all organisms are constructed cells epithelial cell nervous cell muscle cell tissues epithelial tissue nervous tissue muscle tissue organs multicellular organism Cells are small: typically 5 – 20 µm (0.005 – 0.02 mm) in diameter bacterium Frog egg 2500 µm 1 µm Most cells are invisible to the naked eye, so scientists did not know of their existence prior to the invention of the light microscope in the 17th century Robert Hooke’s light microscope, 1665, later refined by Anton Leewenhoek A thin slice of cork, showing the network of pores Hooke called the pores cells (from the Latin cellula meaning “small room”) because they reminded him of the small rooms inhabited by monks living in a monastery Relative sizes of cells and cell components, and the units in which they are measured 1 m = 106 µm = 109 nm = 1010 angstrom (Å) Basic Properties of Cells 1. Life is the most basic property of cells. Cells can grow and reproduce in culture for extended periods. – HeLa cells are cultured tumor cells isolated from a cancer patient (Henrietta Lacks) by George Gey in 1951. – Cultured cells are an essential tool for cell biologists. HeLa: first human cells for extended culturing Basic Properties of Cells 2. Cells are highly complex and organized – Cellular processes are highly regulated. – Cells from different species share similar structure, composition and metabolic features that have been conserved throughout evolution. Levels of cellular and molecular organization Basic Properties of Cells 3. Cells possess a genetic program and the means to use it – Genes encode information to build each cell, and the organism. – Genes encode information for cellular reproduction, activity, and structure. 4. Cells are capable of producing more of themselves - Cells reproduce, and each daughter cell receives a complete set of genetic instructions. 5. Cells acquire and utilize energy – Photosynthesis provides fuel for all living organisms. – Animal cells derive energy from the products of photosynthesis, mainly in the form of glucose. – Cell can convert glucose into ATP—a substance with readily available energy. Basic Properties of Cells 6. Cells carry out a variety of chemical reactions, the sum of which is called metabolism 7. Cells engage in mechanical activities 8. Cells are able to respond to stimuli 9. Cells are capable of self-regulation Self-regulated development in the 10. Cells evolve sea urchin during normal (L) and cell separation experiments (R) Two types of cells Prokaryotic and eukaryotic - distinguished by their size and type of organelles. Prokaryotes are all bacteria, which arose ~3.7 billion years ago. Eukaryotes include protists, animals, plants and fungi. All cells contain DNA (deoxyribonucleic acid) as a store of genetic information Prokaryotic cells Eukaryotic cells > From the Greek words pro, > From the Greek words eu, meaning “before”, and karyon, meaning “truly” or “well”, and meaning “nucleus” karyon, meaning “nucleus” > DNA is not segregated within a > DNA is segregated within a defined nucleus defined nucleus Prokaryotes comprise a single membrane-limited compartment outer membrane inner membrane Nucleoid a single circular DNA molecule, which is not surrounded by a Cytoplasm membrane separating Contains ~ 30,000 it from the cytoplasm, ribosomes (the sites the region of the cell of protein synthesis), lying outside the which account for its nucleoid granular appearance 1 µm Electron micrograph of a typical prokaryotic cell, the bacterium, Escherichia coli Common features of prokaryotic and eukaryotic cells Plasma membrane of similar construction Genetic information in DNA, using identical genetic code Both store chemical energy in the form of ATP Shared metabolic pathways (glycolysis, TCA cycle) Proteasomes (for protein degradation) of similar construction Features of eukaryotic cells not found in prokaryotic cells Nuclear envelope, separating nucleus from cytoplasm Complex chromosomes that compact into mitotic structures Membrane-bound cytoplasmic organelles Cytoskeleton with associated motor proteins Characteristics that distinguish prokaryotic and eukaryotic cells – Complexity: Prokaryotes are relatively simple; eukaryotes are more complex in structure and function. – Cellular reproduction: Eukaryotes divide by mitosis; prokaryotes divide by simple fission. – Genetic material: Packaging: Prokaryotes have a nucleoid region whereas eukaryotes have a membrane-bound nucleus. Amount: Eukaryotes have much more genetic material than prokaryotes. Form: Eukaryotes have many chromosomes made of both DNA and protein (histones) whereas prokaryotes have a single, circular DNA with no histone proteins. The nucleus is the information store of the cell The nucleus contains molecules of DNA (deoxyribonucleic acid) - extremely long polymers that encode the genetic specification of the organism The nucleus is surrounded by a double membrane, called the nuclear envelope The nucleus communicates with the cytosol via nuclear pores that perforate the envelope outer nuclear membrane perinuclear space inner nuclear membrane The nuclear envelope consists of: Two concentric membranes, called the inner and outer nuclear membranes The nuclear lamina, a fibrous network that provides structural support to the nucleus The nuclear pore complexes, the only channels through which molecules are able to travel between the nucleus and the cytoplasm The nuclear lamina – Supports the nuclear envelope, composed of lamins. – Integrity of nuclear lamina regulated by phosphorylation/dephosphorylation. – Human conditions: lamin A/C mutation causes Hutchinson-Gilford Progeria syndrome, lamin B mutation causes leukodystrophy (loss of myelin) – Mutations in lamin binding protein emerin cause Emery-Dreifuss muscular dystrophy (elbows, neck and heels become stiff, heart problems) Traffic through nuclear pore complexes (NPCs) 1. DNA-binding proteins: - histones, nonhistone proteins, activators and repressors of transcription 2. Messenger RNA (mRNA)-binding proteins 3. Components of the nucleus (lamins) 4. Ribosomal proteins 5. Shuttling nuclear transport receptors (importins): deliver other proteins to the nucleus Traffic through nuclear pore complexes (NPCs) 1. mRNAs (together with mRNA-binding proteins) 2. tRNAs (transfer RNAs) 3. 40S and 60S ribosomal subunits (complexes of ribosomal RNAs [rRNAs] and ribosomal proteins) Nuclear pore complex (NPC) Model of a vertebrate nuclear pore complex (NPC). The structure consists of several parts, including a scaffold that anchors the complex to the nuclear envelope, a cytoplasmic and a nuclear ring, a nuclear basket, and eight cytoplasmic filaments. Structure of Nuclear Pore Complex and its Role in Nucleocytoplasmic Trafficking Huge complex (15-30X mass of a ribosome) that exhibits octagonal symmetry. Channel: 20-to 30-nm-wide FG (phenylalanine-glycine) domains form a hydrophobic sieve that blocks the diffusion of larger macromolecules (greater than about 40,000 Daltons). Nuclear pore complex (NPC) Model of a vertebrate nuclear pore complex (NPC). Three dimensional reconstruction of a portion of a nuclear pore complex showing the localization of individual nucleoporin molecules within the structure Structure of Nuclear Pore Complex and its Role in Nucleocytoplasmic Trafficking Huge complex (15-30X mass of a ribosome) that exhibits octagonal symmetry. Channel: 20-to 30-nm-wide FG (phenylalanine-glycine) domains form a hydrophobic sieve that blocks the diffusion of larger macromolecules (greater than about 40,000 Daltons). Binding of GTP (activation) requires a protein called a GEF (guanine nucleotide exchange factor) and hydrolysis of GTP to GDP (inactivation) requires a GAP (GTPase activating protein). Pi GAP (active) (inactive) effectors Ran-GTP Ran-GDP (e.g. importin b) GEF GTP GDP Import of proteins through the NPC Proteins synthesized in the cytoplasm are targeted for the nucleus by a nuclear localization signal (NLS), e.g. P-K-K-K-R-K-V having basic residues. 1. Proteins with an NLS bind to an NLS receptor (importin a/b heterodimer). 2. The protein/importin complex associates with cytoplasmic filaments. 3. The protein/importin complex passes through the NPC….. 4. …..and associates with a GTPase called Ran. Import of proteins through the NPC Proteins synthesized in the cytoplasm are targeted for the nucleus by a nuclear localization signal (NLS), e.g. P-K-K-K-R-K-V having basic residues. 1. Proteins with an NLS bind to an NLS receptor (importin a/b heterodimer). 2. The protein/importin complex associates with cytoplasmic filaments. 3. The protein/importin complex passes through the NPC….. 4. …..and associates with a GTPase called Ran. 5. The RanGTP-importin b complex is transported back to the cytoplasm where Ran is converted to RanGDP and brought back in to the nucleus. Importin a is returned to the cytoplasm via a protein called exportin. endoplasmic nuclear pore outer The outer nuclear membrane is reticulum complex membrane continuous with the rough endoplasmic reticulum (ER), and the space between the inner and outer nuclear membranes is continuous with the lumen of the rough ER perinuclear outer membrane inner membrane space inner membrane nuclear pore nuclear nucleolus chromatin rough endoplasmic complex lamina reticulum Eukaryotic and prokaryotic ribosomes Early origins of the nucleus A suborganelle of the nucleus, the nucleolus, is a factory where ribosomes are assembled Ribosome assembly in the nucleolus 1. Ribosomal proteins are imported to the nucleus from the cytoplasm 2. These ribosomal proteins are then delivered to the nucleolus and assemble on pre-rRNA (pre-ribosomal RNA) 3. The pre-rRNA is cleaved to form several rRNAs 4. Ribosomal proteins and rRNAs assemble to form the 40S and 60S ribosomal subunits 5. These subunits are exported to the cytoplasm (fully assembled ribosome is too large to fit through pore, ensuring protein synthesis takes place in cytoplasm) The chromosomal DNA is packed into chromatin fibers with the aid of specialized proteins Chromatin The complexes composed of eukaryotic DNA and proteins are called chromatin Chromatin contains about twice as much protein as DNA The major proteins of chromatin are the histones Histones are small proteins (11 to 23 kDa) containing a high proportion of basic amino acids (arginine and lysine) that facilitate binding to the negatively charged DNA molecule DNA P P P P - - - - Chromatin + + + + Arg Lys Arg Lys Histones 5 major types of histones H1 H2A H2B H3 H4 Histones are very similar among different species of eukaryotes Chromatin also contains an approximately equal mass of nonhistone chromosomal proteins (more than a 1000 different types) The basic structural unit of chromatin is called the nucleosome Nucleosome core Linker Nonhistone particle protein DNA Histone H1 The DNA is wrapped around an octamer of histones H2A, H2B, H3 and H4 in a nucleosome core particle and sealed by histone H1. This produces a 7-fold compaction of the DNA. Non-histone proteins bind to the linker DNA between nucleosome core particles Higher-ordered structures of chromatin Formation of 30 nm fibers are dependent upon histone interactions and further increase compaction by ~6 fold Higher-ordered structures of chromatin The 30 nm fibers are then organized into 80-100 nm supercoiled loops, possibly stabilized by a protein called cohesin. Mitotic chromosomes represent the ultimate in chromatin compactness with a ratio of 10,000:1 (1 µm of mitotic chromosome length contains ~1 cm of non-compacted DNA). As a cell prepares to divide into two daughter cells, its chromatin condenses into chromosomes that can be distinguished in the light microscope. Human mitotic Chromosomes can be arranged chromosomes labeled in a karyotype (a preparation with different specific of homologous pairs ordered fluorescent dyes. according to size; may be used to screen chromosomal abnormalities). Heterochromatin and Euchromatin Euchromatin returns to a dispersed state after mitosis. Heterochromatin is condensed during interphase. Constitutive heterochromatin remains condensed all the time. – Found mostly around centromeres and telomeres. – Consists of highly repeated sequences and few genes. Facultative heterochromatin is inactivated during certain phases of the organism’s life (X- inactivation) Mitochondria play a critical role in the generation of metabolic energy in eukaryotic cells They oxidize carbohydrates and lipids to produce the basic chemical fuel adenosine triphosphate (ATP) by a process called oxidative phosphorylation ATP is used in a variety of energy-requiring reactions within cells Because mitochondria consume oxygen and release carbon dioxide in the course of ATP production, the entire process is called cellular respiration, from its similarity to breathing Morphology Depending upon the cell type and physiological conditions, mitochondria can have different overall structures Mitochondria can appear as a highly branched, interconnected tubular network. Micrograph of a mammalian fibroblast that has been fixed and stained with fluorescent antibodies to a mitochondrial (green) and a cytoskeletal (red) protein The balance between fusion and fission is a major determinant of mitochondrial morphology Single mitochondrion: ~4 µm length Observations of fluorescently labeled mitochondria within living cells have shown them to be dynamic organelles capable of dramatic changes in shape: - they can fuse with one another (fusion) or split into two (fission) Fission is induced by contact with endoplasmic reticulum (ER) tubules. GTPase (like Ran) Mitochondria arise from preexisting mitochondria by fission Mitochondria are surrounded by a double-membrane system, consisting of inner and outer mitochondrial membranes separated by an intermembrane space. The inner membrane forms numerous folds (cristae), which extend into the interior (or matrix) of the organelle. Its surface area is substantially increased by its folding into cristae. The mitochondrial matrix contains: Enzymes responsible for the oxidative breakdown of carbohydrates and lipids via the citric acid cycle, and enzymes required for the expression of mitochondrial genes Several identical copies of circular DNA molecules (mitochondrial genome) Special mitochondrial ribosomes Inner mitochondrial membrane: The principal site of ATP synthase Outer membrane: Contains enzymes that convert lipid substrates into forms that are subsequently metabolized in the matrix Energy (ATP) production in the mitochondrion intermembrane space inner membrane matrix This electron transport generates a proton gradient across the inner membrane, which is used to drive the production of ATP by ATP synthase In the process of oxidative phosphorylation, high-energy electrons from NADH and FADH2 are then passed along the electron-transport chain in the inner membrane to oxygen (O2) Pyruvate and fatty acids enter the mitochondrion, are broken down to acetyl CoA, and are then metabolized by cytosol the citric acid cycle, which sugars lipids produces NADH and FADH2 Electron transfer Types of carriers 1. Flavoproteins, contain NAD+, FAD+ prosthetic groups 2. Cytochromes, contain heme prosthetic groups Fe3+ → Fe2+ (e.g. cytochrome c) 3. Copper-containing proteins, Cu2+ → Cu1+ 4. Ubiquinone (or coenzyme Q), only carrier to not associate with a protein 5. Iron-Sulfur proteins Glycolysis – generation of 2 ATP and 2 NADH What happens to the pyruvate? Is there oxygen (O2) present? YES: oxidative phosphorylation to generate lots of ATP NO: fermentation, regenerates NAD+ so the cells can further metabolize glucose and produce small amounts of ATP (yeast, muscle cells under stress, tumour cells) ethanol lactic acid The TCA cycle (aka Krebs cycle or citric acid cycle) A stepwise cycle where substrate is oxidized and its energy conserved. The two-carbon acetyl group from acetyl CoA is condensed with the four-carbon oxaloacetate to form a six- carbon citrate. During the cycle, two carbons are oxidized to CO2, regenerating the four-carbon oxaloacetate needed to continue the cycle. Each pyruvate that enters the mitochondrion generates: 4 NADH (3 from the TCA cycle, 1 from production of Acetyl-CoA) 1 FADH2 1 GTP So, including glycolysis, after the TCA cycle there is a TOTAL of: 10 NADH 2 FADH2 2 ATP 2 GTP During oxidative phosphorylation, it is estimated that each NADH molecule generates ~2.5 ATP molecules and each FADH2 molecule generates ~1.5 ATP molecules (enters the electron transport chain further downstream of NADH). Therefore, the overall yield of ATP molecules from a single glucose molecule is ~30. The complexes of the electron transport chain 1) Electrons derived from either NADH (via complex I or NADH dehydrogenase) or FADH2 (complex II or succinate dehydrogenase) are passed to ubiquinone (Q or UQ), a lipid-soluble molecule II 2e- FAD+ FADH2 Succinate Fumarate 2) The electrons are then passed from coenzyme Q (a.k.a. ubiquinone) to complex III (or the cytochrome b-c1 complex) II 2e- FAD+ FADH2 Succinate Fumarate 3) Electrons are then transferred to cytochrome c, a peripheral membrane protein, which carriers electrons to complex IV (or cytochrome oxidase) II 2e- FAD+ FADH2 Succinate Fumarate 4) Complex IV transfers electrons to molecular oxygen to form H2O within the matrix II 2e- FAD+ FADH2 Succinate Fumarate 5) The electron transfers in complexes I, III and IV generate energy, which is used to pump protons from the matrix to the intermembrane space, establishing a proton gradient across the inner membrane. The energy stored in the proton gradient is then used to drive ATP synthesis as the protons flow back to the matrix through complex V (or ATP synthase) II 2e- FAD+ FADH2 Succinate Fumarate ATP production is blocked by metabolic poisons such as cyanide, sodium azide and carbon monoxide – bind to catalytic sites of complex IV. Electron transport can be “uncoupled” from ATP synthesis by endogenous proteins (uncoupling proteins) such as UCP1 in brown adipose tissue. UCP1 dissipates the H+ gradient and the energy of electron transfer is released as heat rather than a high- energy ATP molecule. Chemicals (e.g. 2,4-dinitrophenol (or DNP)) also dissipate the proton gradient and prevent ATP production. Therapeutic mechanism: Reduced [ATP] causes the cell to oxidize stores of fat to replenish the [ATP]. Therefore, burn fat resulting in weight loss (in theory). Mitochondrial disorders Disorders that are due to abnormalities in mitochondrial structure and function most dramatically affect muscle and nerve tissues because of their high demand for ATP. Ragged-red fibers in skeletal muscle of a patient suffering from the Myoclonic Epilepsy and Ragged Red Fibers (MERRF) disorder. The red-stained “blotches” just beneath the cells’ plasma membrane are due to abnormal proliferation of mitochondria that have decreased cytochrome c oxidase (complex IV) activity. Mitochondrial disorders Buildup of ROS in mitochondria cause a 10-fold increase in mutation rate of mitochondrial (mt)DNA compared to nuclear DNA. Thus, long-lived cells (nerve cells, muscle cells) may accumulate undesirable mtDNA mutations that can result in adult-onset neurological disorders (e.g. PD) and contribute to aging. Heroin contaminated with a drug called MPTP caused young adults to present with PD-like tremors. MPTP interferes with complex I. PD patients have a decrease in complex I activity. Yeast “petite” colonies arise from loss of mitochondrial DNA. They cannot respire and hence can only utilize fermentable carbon sources (e.g. glucose) but not non-fermentable carbon sources (e.g. glycerol, ethanol). Origins of mitochondria Endosymbiont theory: mitochondria (and chloroplasts) are derived from smaller prokaryotic cells that took up residence in a eukaryotic cell. Support for the theory: 1. Outer membrane of bacteria and mitochondria contain porins 2. Inner membrane of bacteria and mitochondria contain the lipid cardiolipin 3. Mitochondria arise from pre-existing mitochondria via fission 4. Mitochondria and bacteria contain a single, circular DNA 5. Mitochondrial ribosomes are similar to those of bacteria (70S) Chloroplasts resemble mitochondria in that both contain a permeable outer membrane and a relatively impermeable inner membrane Stroma is analogous to the mitochondrial matrix. A third membrane is formed by the thylakoids (orderly stacks are called grana). Chloroplasts: are the largest and most characteristic organelles in the cells of plants are the sites of photosynthesis – a series of light-driven reactions that creates organic molecules from atmospheric carbon dioxide (CO2) perform photosynthesis during the day light hours and thereby produce ATP and NADPH, which in turn are used to convert CO2 into sugars inside the chloroplast H2 O CO2 LIGHT Photosynthetic Photosynthetic electron-transfer ATP carbon-fixation SUGARS reactions, “light NADPH reactions, “dark reactions” reactions” O2 In plants, the chloroplasts and mitochondria collaborate to supply cells with metabolites and ATP The plant contains many cells that lack chloroplasts and therefore cannot produce their own sugars and ATP. Therefore for most of its ATP production, the plant relies on an export of sugars from its chloroplasts to the mitochondria that are located in all cells of the plants. Most of the ATP needed by the plant is synthesized in these mitochondria. Leaf cells Root cells Three of four stages in photosynthesis (the “light reactions”) take place in the thylakoid membranes: 1. Absorption of light by green pigments (chlorophylls) attached to proteins 2. Electron transport to generate a H+ gradient 3. Synthesis of ATP and NADPH Each photosynthetic unit contains several hundred chlorophyll molecules. One member of the group—the reaction- center chlorophyll—transfers electrons to an electron acceptor. The other chlorophyll molecules act as harvesting antennae and rapidly transfer photons to the reaction center (antenna pigments). Electron transport generates a H+ gradient across the thylakoid membrane with the lumen containing a higher concentration of protons compared to the stroma. Photosynthetic units are found in two large protein complexes called Photosystems I and II (PSI and PSII). These complexes are embedded in the thylakoid membrane. When the reaction center of PSII is excited by a photon, water is split into O2, protons are released and the electrons enter the transfer chain. The electrons are transferred from PSII to the cytochrome b6-f complex by the lipid-soluble plastoquinone (Q). Electron transfer through cytochrome b6-f allows for the entry of more protons into the lumen of the thylakoid. Plastocyanin, a lumenal protein of the thylakoid, transfers the electrons to PSI, which in turn passes the electrons to a water- soluble molecule called ferrodoxin. This Fe-S protein associates with ferrodoxin-NADP+ reductase (FNR) to produce NADPH. The ATP synthase of the thylakoid membranes then couples proton movement into the stroma to ATP synthesis. Herbicides target: PSII (diuron, atrazine, terbutryn) by blocking electron transport through this complex PSI (paraquat) by competing with ferrodoxin for electrons, which are transferred to O2 to create reactive oxygen that damages chloroplasts and kills the plant. It also diverts electrons from complex I in humans with similar effects. Comparison between chloroplasts/photosynthesis light reactions (PSLR) and mitochondria/oxidative phosphorylation (OP) Both generate ATP, and both use a proton pump to do so. Both contain DNA and ribosomes. Both are surrounded by a double membrane. Chloroplasts contain an additional third membrane (thylakoid) while the inner mitochondrial membrane forms cristae Mitochondria rely on both a proton gradient and a charge gradient across the inner membrane to generate ATP, while chloroplasts rely on a proton gradient (the charge is neutralized by the permeability of the thylakoid membrane to ions such as Cl- and Mg2+). The electron transfer chain of both organelles is composed of a number of large protein complexes. The terminal electron acceptor in OP is O2 and in PSLR it is NADP+. OP requires O2 and produces CO2 as a byproduct while PSLR produce O2 and (in the Calvin cycle) utilize CO2. Carbon fixation and the Calvin cycle H2O CO2 LIGHT Photosynthetic Photosynthetic electron-transfer ATP carbon-fixation SUGARS reactions, “light NADPH reactions, “dark reactions” reactions” O2 6RuBP + 6CO2 + 18ATP + 12 NADPH → 6 RuBP + C6H12O6 + 18ADP + 18Pi + 12NADP+ Rubisco is highly inefficient (fixes only 3 CO2/sec). Therefore, cells need abundant amounts (50% of leaf protein). Hence, given the number of plants on earth, it is the most abundant protein on the planet (5-10kg of rubisco for every human). Consumes 18 ATP/glucose molecule. Oxidative phosphorylation of that glucose molecule results in ~30 ATP, therefore net gain.