Muscle Fatigue Biom2012 Lecture 1 PDF
Document Details
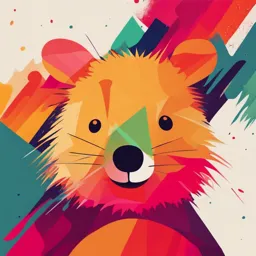
Uploaded by EasiestChrysanthemum3413
University of Queensland
Prof Bradley Launikonis
Tags
Summary
This document is a lecture on muscle fatigue. It discusses muscle excitation-contraction coupling and different types of muscle fatigue. The document also includes information about the determinants of the resting membrane potential and muscle fiber types.
Full Transcript
Muscle fatigue biom2012 (lecture 1) Prof Bradley Launikonis [email protected] Overview Reiterate normal Excitation-Contraction coupling What is muscle fatigue? (in exercise, disease) Characterise types of muscle fatigue Discuss factors involved in each type Brief backgr...
Muscle fatigue biom2012 (lecture 1) Prof Bradley Launikonis [email protected] Overview Reiterate normal Excitation-Contraction coupling What is muscle fatigue? (in exercise, disease) Characterise types of muscle fatigue Discuss factors involved in each type Brief background. Muscle cells are also called ‘fibres’ (same thing). Massive in comparison to other cells. Outside of the cell (extracellular). Inside of the cell (intracellular). ‘myo’= muscle, outside inside ‘sarco’ = flesh ~ 3 mM K+ ~ 140 mM K+ ~ 1 mM Mg2+ ~ 1 mM Mg2+ ~ 104 mM Na+ ~ 4 mM Na+ ~ 95 mM Cl- ~ 3 mM Cl- ~ 2 mM Ca2+ ~ 0.0001 mM Ca2+ ~ 7 mM HCO3- ~ 7 mM HCO3- Determinants of the resting membrane potential (EM) The RMP(EM) is the potential at which there is no net current across the membrane (ie. the sum of all the currents carried by the different ion species is zero). This does not mean that each type of ion is in equilibrium - this only occurs for a given type of ion at its own Nernst (or equilibrium) potential, and clearly the Nernst potential for the various ions are very different. EM is the potential where the total of all the inward currents is equal to the total of all the outward currents. The current carried by a particular ion species depends on how permeable the cell membrane is to that type of ion. The most permeable ions (K+, Cl− and Na+) will have the biggest effect. The Goldman-Hodgkin-Katz (GHK) equation allows EM to be calculated from the concentration differences for each ion and its relative permeability (PX). PK [K]o + PNa [Na]o + PCl [Cl]i GHK Equation: EM = 58 log10 −−−−−−−−−−−−−−−−−−−−−−−−−−− (in mV) PK [K]i + PNa [Na]i + PCl [Cl]o Thus, if the ratio of PK : PNa : PCl is 1 : 0.01 : 2 EM = 58 log10 3 + 0.01 x 104 + 2 x 3 = 58 log10 (0.0304) = -88 mV 140 + 0.01 x 4 + 2 x 95 What happens when permeabilities change? Exercise (to start now): calculate the resting Em, if: the normal ratio of PK : PNa : PCl is 1 : 0.01 : 2 Was changed to PK : PNa : PCl is 1 : 0.01 : 1 or PK : PNa : PCl is 1 : 0.01 : 0 or PK : PNa : PCl is 1 : 0.01 : 2 or PK : PNa : PCl is 1 : 10 : 2 The ECl is negative (about the same as the normal resting Em). What effect would changing Cl permability have on the rate of depolarization and repolarization of the Em during an action potential? Would it be faster or slower (easier or harder) to change? Muscle types 1. Striated (striped) - Cardiac (constancy the key) - Skeletal (strength, speed and agility required) 2. Smooth (visceral) In all muscle types, contraction is initiated by a rise in intracellular ionised CALCIUM concentration (ie. [Ca2+]), in response to ELECTRICAL and/or PHARMACO-CHEMICAL stimulation. Note: (Here, skeletal muscle will be used as a model to discuss muscle fatigue) Terminology Sarcoplasm, myoplasm, cytoplasm and intracellular are all terms used to describe the inside of the muscle cell/fibre. ‘ATPase’ or ‘pump’ is used to describe an active process by which energy in the form of ATP hydrolysis is used to translocate a certain ion across a membrane against its concentration gradient. Functions of muscle in mammals 1. Locomotion 2. Respiration 3. Blood circulation 4. Digestion 5. Excretion 6. Reproduction 7. Vocalisation 8. Vision 9. Thermogenesis General characteristics of skeletal muscle fibres Muscle cells extend from tendon to tendon Length is from millimetres (mm) to tens of centimetres (cm) Diameter of fibres is Human skeletal muscle fibre – nuclei (blue) 60x magnification between 5 to 80 m All skeletal muscle fibres are multinucleated cells, with peripheral nuclei. Structural overview of adult skeletal muscle Length: mm to many cms Skeletal muscle Each skeletal muscle fibres is innervated by one motor neuron Motor unit = basic unit of motor function = one motor neuron together with all muscle fibres it innervates Size of the motor unit varies between 3-6 muscle fibres in muscles in which fine movement control is necessary (some ocular muscles, muscles in the hand and arm) to 2000 muscle fibres in muscles responsible for power (gastrocnemious, trapezius). In mammals, the strength of contraction is normally determined by the number of motor units which are activated Classes of fibres Twitch fibres Have one excitatory synapse in the middle region of the fibre Generate ‘all or none’ action potentials Respond with ‘all or none’ contractile events → twitches Involved in the rapid movement of parts of the body. Twitch fibres (cont’) Slow-twitch fibres are fatigue resistant, (oxidative) Fast-twitch fibres can be either glycolytic or oxidative and glycolytic → rapid movements. Superfast MHC type I antibody, texas red 2o antibody CreaT antibody, FITC (green) 2o antibody Tetani When twitch fibres are stimulated at high enough rate, there cannot be a fusion of the action potentials because they are ‘all or none events’, but there is a fusion of the [Ca2+] transient responses in the muscle fibre which is further reflected into the complete or incomplete fusion of the ‘twitch’ force responses. The completely or incompletely fused force responses are known as ‘complete’ or ‘incomplete tetani’ (also fused or unfused). When twitch fibres are stimulated at high enough rate The fused tetanus can be several fold greater than the twitch force. Fused 0.15 mN tetanus Unfused tetanus 3 Twitches Twitch 7Hz 15Hz 50Hz 400 ms (40 ms apart) (35 ms apart) (20 ms apart) Muscle Fatigue Multifactorial 1 Many aspects 1) CNS and PNS 2 2) Neuro-muscular junction? 3) Muscle itself. 3 (including t-tubules) We will focus on 3. ‘Muscle’ Fatigue Biom2011 revision T- tubule Myoplasm SR Inhib. Kd ~0.1mM 2+ Mg ATP DHPR Stimulatory site Ca 2+ 2+ Mg Act. Kd ~1M RyR1 What is ‘Muscle Fatigue’? Typically defined as a decline in peak force production and muscle power output associated with intense or prolonged muscle activity. Muscle fatigue depends on: - intensity and duration of stimulation - muscle fibre type Broad classification of muscle fibre types: a) Slow-twitch fibres (Type I) b) Fast-twitch fibres (Type II) Muscle fibre types: a) Slow-twitch fibres (Type I) - red appearance, many mitochondria, - lower force output, fatigue ‘resistant’, - postural or repetitive role b) Fast-twitch fibres (Type II) - white appearance, fewer mitochondria, - high force output, fatigues faster, - recruit for ‘explosive’ contraction Note: there are actually many varieties of fibres with different isoforms of myosin II, and some having more mitochondria. Types of muscle fatigue: a) High frequency fatigue b) ‘Metabolic’ fatigue c) Long duration fatigue Types of muscle fatigue: a) High frequency fatigue - fast onset - fast recovery b) ‘Metabolic’ fatigue c) Long duration fatigue extracellular space sarcolemma intracellular space Na+ K+ Contractile proteins Ca2+ Ca2+ Ca2+ Ca2+ sarcoplasmic reticulum (SR) Ca2+ Ca2+ Ca2+ Ca2+ Ca2+ High Frequency Fatigue in a toad gastrocnemius muscle: (100 Hz, direct stimulation). Fast onset (~5sec) 30 s Fatigue (decline) Force 1 min rest 100 Hz 100 Hz extracellular space sarcolemma intracellular space K+ buildup depolarizes t-tubule → Na+ channel inactivation → Action Potential failure in t-tubule K+ K+ → no activation of DHPR molecule no Ca2+ release X Ca2+ Ca2+ Ca2+ Ca2+ sarcoplasmic reticulum (SR) Ca2+ Ca2+ Ca2+ Ca2+ Ca2+ extracellular space sarcolemma intracellular space With short rest, K+ is pumped back into the cell and/or diffuses out of the restricted space. The membrane K+ repolarizes and the Na+ channels reset. Na+ Ca2+ Ca2+ Ca2+ Ca2+ sarcoplasmic reticulum (SR) Ca2+ Ca2+ Ca2+ Ca2+ Ca2+ High Frequency Fatigue in a toad gastrocnemius muscle: (100 Hz, direct stimulation). Fast recovery (~98% initial level in 1 min) 30 s Fatigue (decline) Force 1 min rest 100 Hz 100 Hz 3mM Cl- Zero Cl- Recovery from high frequency fatigue (Dutka, Murphy & Lamb, 2008 J Physiol) No Cl- extracellular space sarcolemma intracellular space K+ movement repolarizes fibre K+ build-up depolarizes t-tubule K+ K+ Ca2+ Ca2+ Ca2+ Ca2+ sarcoplasmic reticulum (SR) Ca2+ Ca2+ Ca2+ Ca2+ Ca2+ with Cl- extracellular space sarcolemma intracellular space K+ build-up reduced by Cl- repolarizing fibre K+ does not build-up in t-tubule Cl- Cl- K+ K+ Ca2+ Ca2+ Ca2+ Ca2+ sarcoplasmic reticulum (SR) Ca2+ Ca2+ Ca2+ Ca2+ Ca2+ High Frequency Fatigue Fatigue (decline) Force Cytoplasmic Ca2+ 100 Hz 30 s time