Muscle Fatigue Lecture Notes PDF
Document Details
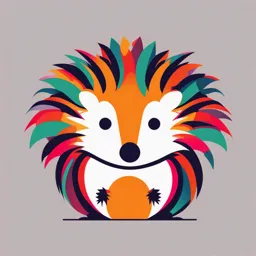
Uploaded by ThoughtfulRetinalite
UNSW Sydney
Dr. Frederic von Wegner
Tags
Summary
These lecture notes from UNSW Sydney cover muscle fatigue. The document includes a variety of details about muscle fatigue, including learning objectives, literature review, and various mechanisms.
Full Transcript
Muscle fatigue Dr. Frederic von Wegner WARNING This material has been reproduced and communicated to you by or on behalf o...
Muscle fatigue Dr. Frederic von Wegner WARNING This material has been reproduced and communicated to you by or on behalf of the University of New South Wales in accordance with section 113P of the Copyright Act 1968 (Act). The material in this communication may be subject to copyright under the Act. Any further reproduction or communication of this material by you may be the subject of copyright protection under the Act. Do not remove this notice Learning objectives - give a definition of muscle fatigue - describe changes in cellular excitability during muscle fatigue - describe metabolic changes in muscle fatigue - differences in slow- and fast-twitch fibres - fatigue-reducing mechanisms - explain what is meant by slow recovery - give examples of in vivo fatigue experiments - give an example of fatigue in disease Literature Textbooks: - Guyton-Hall, Textbook of Medical Physiology, pp. 86 ff. - Boron, Medical Physiology, pp.1257 ff. - Martini, Fundamentals of Anatomy and Physiology, pp. 318 ff. - Silverthorn, Human Physiology, pp. 417 ff. - Stanfield, Principles of Human Physiology, pp. 344+345 - Articles: - D.G. Allen et al., Skeletal Muscle Fatigue: Cellular Mechanisms. Physiological Reviews 88, 2008 - R.M. Enoka, J. Duchateau, Muscle Fatigue: what, why and how it influences muscle function. J. Physiol. 2008 - R.M. Enoka, J. Duchateau, Translating Fatigue to Human Performance, Medicine & Science in Sports & Exercise, 2016 - J.A. Kent-Braun et al., Skeletal Muscle Fatigue, Comprehensive Physiology, 2012 Muscle fatigue - definition(s) ‘Intensive activity of muscles causes a decline in performance, known as fatigue...’ (Allen & Westerblad, 2001) ‘Performing a motor task for long periods of time induces motor fatigue, which is generally defined as a decline in a person’s ability to exert force.’ (Lorist et al. 2002) ‘...CNS administration of caffeine increased treadmill run time to fatigue...’ (Davis et al. 2003) ‘…a fatiguing task was performed with the muscles of the left hand until the muscles were exhausted.’ (Edgley & Winter, 2004) ‘Fatigue is known to be reflected in the EMG signal as an increase of its amplitude and a decrease of its characteristic spectral frequencies.’ (Kallenberg et al. 2007) ‘...the sensation of fatigue is the conscious awareness of changes in subconscious homeostatic control systems...’ (St Clair Gibson et al. 2003) ‘The primary purpose of the study was to use functional magnetic resonance imaging (fMRI) to determine the association between feelings of mental fatigue and blood oxygen level dependent (BOLD) brain responses during a mentally fatiguing cognitive task.’ (Cook et al. 2007) Enoka & Duchateau, J Physiol, 2008 Muscle fatigue - definition(s) Def. fatigue: an exercise-induced impairment of motor performance … sensation of tiredness and weakness from neurological disorders Def. muscle fatigue: an exercise-induced reduction in the ability of muscle to produce maximal force or power (whether or not task fails) protocol: interrupt task and measure decline in maximum contractions (voluntary or electrical) - fatigue is not: task failure or complete exhaustion - fatigue: decrease in MVC force, submaximal force can often be sustained Fatigue mini summary: many factors and task-dependence Message: - there is no single factor that determines fatigue - there are factors: inside the muscle fibres (cells) in the extracellular medium surrounding the muscle fibres (Na+, K+) in peripheral nerves in the central nervous system (spinal cord, brain) - this lecture: factors related to muscle fibres themselves - CNS-fatigue: Lecture 18 - task-dependence: each muscle, for different tasks shows a different fatigue behaviour Reminder: excitation-contraction coupling - nerve impulse, ACh release at the motor end plate - generation of muscle action potential - AP propagation in transverse tubule system (TTS) - activation of the voltage sensor (Ca2+ channel, DHPR) - coupling of sarcolemmal voltage sensor and sarcoplasmic reticulum (SR) Ca2+ release channel (RyR) - intracellular Ca2+ release - Ca2+ activates cross-bridge cycle in the presence of ATP - ATP → ADP + Pi - Ca2+ reuptake into the SR Boron, Medical Physiology Experimental approaches to study fatigue Allen et al., Physiol Rev 2008 Experimental approaches to study fatigue Allen et al., Physiol Rev 2008 Experimental approaches to study fatigue Allen et al., Physiol Rev 2008 Experimental approaches to study fatigue Allen et al., Physiol Rev 2008 Three phases of cellular fatigue (fast-twitch) tetani without mitochondria Allen et al., Physiol Rev 2008 Three phases of fatigue in fast-twitch fibres (typical, some exceptions) Phase 1: - quick decline in force (10-20%), (tetanic) Ca2+ increases - decreased force production by cross-bridges Allen et al., Physiol Rev 2008 Three phases of fatigue in fast-twitch fibres (typical, some exceptions) Phase 1: - quick decline in force (10-20%), (tetanic) Ca2+ increases - decreased force production by cross-bridges Phase 2: - relative constant force - duration is fibre type dependent, capacity to do oxidative phosophorylation (exp.: cyanide, lower O2) - in whole muscle: continuous decay, average of diff. fibre types Allen et al., Physiol Rev 2008 Three phases of fatigue in fast-twitch fibres (typical, some exceptions) Phase 1: - quick decline in force (10-20%), (tetanic) Ca2+ increases - decreased force producion by cross-bridges Phase 2: - relative constant force - duration is fibre type dependent, capacity to do oxidative phosophorylation (exp.: cyanide, lower O2) - in whole muscle: continuous decay, average of diff. fibre types Phase 3: - rapid decline in force and tetanic Ca2+ - can be reversed by pharmacological stimulation of Ca2+ release from the SR (caffeine) – in isolated fibres! Allen et al., Physiol Rev 2008 Motoneuron and fibre-type dependency Purves, Neuroscience Fibre-type dependency Fast-twitch fibre (FDB) # tetanic contr. phase 1 phase 2 phase 3 easily fatigued Allen et al., Physiol Rev 2008 Fibre-type dependency Fast-twitch fibre (FDB) Slow-twitch fibre (soleus) # tetanic contr. phase 1 phase 2 phase 3 easily fatigued fatigue-resistant Allen et al., Physiol Rev 2008 Excitability: changes in Na+ and K+ - resting muscle fibre: intracellular K+ ~ 160 mM (millimolar) extracellular K+ ~ 4 mM - exercising muscle fibre: extracellular K+ can rise to 9 mM locally limited diffusion, blood: K+ approx. constant - Nernst equation: - rising extracellular K+ => depolarization rising intracellular Na+ => lower driving force for Na+ currents smaller action potentials Excitability: 1 - Action potential transmission - requirements for contraction: intracellular Ca2+ release - intracellular Ca2+ release depends on activation of the voltage sensor (DHPR) in the plasma membrane - only works if membrane potential is negative - single AP: no relevant K+ shift - repeated action potentials => extracellular K+ accumulation intracellular Na+ accumulation => membrane depolarization Boron, Medical Physiology Excitability: 1 - Action potential transmission - requirements for contraction: intracellular Ca2+ release - intracellular Ca2+ release depends on activation of the voltage sensor (DHPR) in the plasma membrane - only works if membrane potential is negative - single AP: no relevant K+ shift - repeated action potentials O I => extracellular K+ accumulation intracellular Na+ accumulation => membrane depolarization => Na+ channel inactivation = loss of excitability C Bagal, Channels 9(6), 2015 Excitability: 2- T-tubular conduction of the action potential - to achieve synchronous activation throughout the muscle fibre, the AP has to reach all voltage sensors at the same time - T-tubule membrane ~ 5 x surface membrane area - continuous activity: depolarization, Na+ channel inactivation => decreased excitability - high-intensity exercise: cell swelling and formation of membrane vacuoles => t-tubular ‘obstruction’, impaired diffusion clearing of K+ and metabolites Aidley, The Physiology of Excitable Cells Excitability: in vivo? (... or only in vitro?) - during continuous maximal voluntary contractions (MVC) M. abd. pollicis: - clear decline in force … but maximum electrical stimulation shows larger compound muscle AP - in vitro: tetanic force more severely affected than single twitches in vivo: in vitro findings difficult to reproduce - summary: metabolic factors in vivo probably more important than electrical failure Metabolic processes in working muscle - ATP is the “fuel” used by the molecular motors (myosin head = ATPase) - ATP is constantly regenerated 1. creatine phosphate (CP) 2. glycogen = glucose store (glucose polymer), stored inside muscle fibres, large store in the liver 3. two ways of “burning” glucose: anaerobic (lactate), aerobic (CO2) Martini, Fundamentals of Anatomy & Physiology Metabolic processes in moderate and intense exercise Stanfield, Principles of Human Physiology Metabolic processes in exercising muscle - metabolic changes: ATP concentration is maintained high during exercise - different sources of ATP regeneration are activated during different phases of exercise - even during intense exercise, ATP probably doesn’t fall below 60% initial conc. - local ATP depletion in certain compartments (triad: t-tubule, sarcoplasmic reticulum) cannot be excluded - with decreasing ATP, free Mg2+ increases => inhibitory effects (“Ca2+ antagonist”) Allen et al., Physiol Rev 2008 Metabolic factors: 1 – inorganic phosphate (Pi) - prolonged muscle contraction => accumulation of anorganic phosphate (Pi) - Pi leads to: - decrease of myofibrillar force (Pi bound myosin head = weak actin-myosin binding Pi release = strong actin-myosin binding) - decreased Ca2+ sensitivity of motor proteins - decreased Ca2+ release from the sarcoplasmic reticulum (controversial in early phase) multiple effects on RyR (release channels), Ca2+ pumps and buffering - Pi makes a major contribution to phase 3 of fast-twitch fibre fatigue (reduced Ca2+ release) => Pi is an important metabolic factor in muscle fatigue Metabolic factors: 2 – lactate and pH (H+) - historically: lactate proposed as the main factor in muscle fatigue - metabolism produces lactate + H+ - temporal correlations between lactate/H+ inconsistent, causality unclear - side effects: changes in osmolarity => changes in ionic concentrations - beneficial effects of lactate overlooked => summary: lactate not a major contributor to fatigue Metabolic factors: 2 – lactate and pH (H+) - historically: lactate proposed as the main factor in muscle fatigue - metabolism produces lactate + H+ - temporal correlations between lactate/H+ inconsistent, causality unclear - side effects: changes in osmolarity => changes in ionic concentrations - beneficial effects of lactate overlooked => summary: lactate not a major contributor to fatigue - during exercise, pH drops from ca. 7.05 to ca. 6.5 (extreme) but fatigue also occurs with minimal pH changes - during recovery, force recovers much faster than pH - in experiments, isolated pH reduction even increases force - voltage sensor activation of Ca2+ release not affected - motor proteins slightly less sensitive to Ca2+, but free Ca2+ higher - acidic pH improves membrane excitability during fatigue => summary: direct effects on force production small, role in fatigue probably minor Metabolic factors: 3 – ATP and magnesium (Mg2+) - exercise: ATP initially stable, then decline (in fast fibres), with increase in Mg2+ (ATP = efficient Mg2+ buffer), example: ATP from 7 mM to 1.5 mM - force production is reduced only when ATP drops to micromolar conc. - Mg2+ increase reduces myofibrillar sensitivity to Ca2+ (additional to Pi effects) - Ca2+ release from the sarcoplasmic reticulum is strongly inhibited by Mg2+ - summary: Mg2+ limits intracellular Ca2+ release and motor protein function - think of protective effect also: Mg2+ prevents complete depletion of ATP sources by limiting Ca2+ release Reactive oxygen species (ROS) - oxidative metabolism releases reactive compounds most importantly: superoxide (O2- ), hydrogen peroxide (H2O2), hydroxyl radicals (OH ) - can leave muscle cells via anion channels, very short lifetime, react with “anything” - ROS production in active muscle is increased - increased production with higher temperature - ROS scavengers can reduce fatigue in vitro (e.g. superoxide dismutase, SOD enzyme) - in vivo: lack of convincing evidence (vitamin C, E) - fatigue-related mechanisms: - main targets: motor proteins, Na+/K+ ATPase - inconsistent effects on Ca2+ release Allen et al., Physiol Rev 2008 Shortening velocity and relaxation 1. fatigue reduces maximum shortening velocity - main factor: ADP increase - little effects: phosphate, H+, intracellular Ca2+ changes Shortening velocity and relaxation 1. fatigue reduces maximum shortening velocity - main factor: ADP increase - little effects: phosphate, H+, intracellular Ca2+ changes 2. fatigue slows down muscle relaxation - main factors: increases in ADP, Pi and H+ Fatigue reducing mechanisms - some of the discussed mechanisms not only impair muscle function but actually help to counteract fatigue effects - Examples: 1. slowing in relaxation => increases isometric force production, isolated twitches show more fusion when prolonged (more at low stimulation frequency) force production even when neurons are fatigued or muscle cannot produce APs Fatigue reducing mechanisms - some of the discussed mechanisms not only impair muscle function but actually help to counteract fatigue effects - Examples: 1. slowing in relaxation => increases isometric force production, isolated twitches show more fusion when prolonged (more at low stimulation frequency) force production even when neurons are fatigued or muscle cannot produce APs 2. muscle fibre swelling: mechanical effects improve shortening velocity and force production, counteracting other (negative) effects possible effect of creatine supplements! Fatigue reducing mechanisms - some of the discussed mechanisms not only impair muscle function but actually help to counteract fatigue effects - Examples: 1. slowing in relaxation => increases isometric force production, isolated twitches show more fusion when prolonged (more at low stimulation frequency) force production even when neurons are fatigued or muscle cannot produce APs 2. muscle fibre swelling: mechanical effects improve shortening velocity and force production, counteracting other (negative) effects possible effect of creatine supplements! 3. post-tetanic potentiation = short periods of muscle activity transiently increase subsequent force production: phosphorylation of myosin (regulatory light chain) Recovery mechanisms - recovery starts immediately after the fatigue-inducing activity stops - however, muscle function may continue to deteriorate for a while = postcontractile depression - speed of recovery depends on previous activity general rule: fast stimulation => fast recovery (e.g. 30 sec.) low-frequency stimulation => slow recovery (e.g. hours) Recovery mechanisms - recovery starts immediately after the fatigue-inducing activity stops - however, muscle function may continue to deteriorate for a while = postcontractile depression - speed of recovery depends on previous activity general rule: fast stimulation => fast recovery (e.g. 30 sec.) low-frequency stimulation => slow recovery (e.g. hours) moderate stimulation frequencies: stronger effects (larger arrrows) for: a) decreased Ca2+ sensitivity (blue) b) decreased Ca2+ release (red) Allen et al., Physiol Rev 2008 Recovery from fatigue (fast-twitch fibres) 50 Hz stimulation: fatigue and decreased Ca2+ 30 Hz stimulation, fatigue at stable Ca2+ - 100 Hz stimulation: max. force similar pre and post => max. Ca2+ activated force not reduced by fatigue => interpretation: fatigue effect mediated by myofibrillar Ca2+ sensitivity Allen et al., Physiol Rev 2008 Delayed recovery and training - some changes after prolonged exercise continue for hours - they cannot be explained by ATP or creatine-phosphate deficiency (recovery in < 1 hour) - partially explained by electrical properties, impaired muscle AP production - main mechanism: impaired Ca2+ release from the SR (DHPR-RyR coupling) Delayed recovery and training - some changes after prolonged exercise continue for hours - they cannot be explained by ATP or creatine-phosphate deficiency (recovery in < 1 hour) - partially explained by electrical properties, impaired muscle AP production - main mechanism: impaired Ca2+ release from the SR (DHPR-RyR coupling) - delayed recovery is important for training and exercise physiology - incomplete recovery leads to progressive impairment of performance - risk of vicious cycle: reduced performance => more training => incomplete / inefficient recovery - increased risk of lesions, training less rewarding Summary: fatigue-related mechanisms Allen et al., Physiol Rev 2008 Example 1: young vs. old Exp. A: young (30.5 ± 2.5 y) vs. old (77.2 ± 1.4 y) - max. torque maximal shortening and lengthening contractions dorsiflexor muscles, 5 sets x 30 contractions, 1 contr./3.5 sec., 60 sec pause between sets - Results: - young stronger than old (38.3 ± 3.1 Nm) vs. old (28.6 ± 1.3 Nm)) - old show greater decline in max. torque (old: 42.1%, young: 27.1%) - same for shortening and lengthening contractions Enoka, Duchateau, J Physiol 2008 Example 1: young vs. old Exp. A: young (30.5 ± 2.5 y) vs. old (77.2 ± 1.4 y) - max. torque maximal shortening and lengthening contractions dorsiflexor muscles, 5 sets x 30 contractions, 1 contr./3.5 sec., 60 sec pause between sets - Results: - young stronger than old (38.3 ± 3.1 Nm) vs. old (28.6 ± 1.3 Nm)) - old show greater decline in max. torque (old: 42.1%, young: 27.1%) - same for shortening and lengthening contractions Exp. B: young (21.5 ± 4.4 y) vs. old (71.3 ± 2.9 y) - submaximal contractions (20% MVC) with elbow flexors - 8 force-matched old-young pairs (male), stop: task failure, task = target torque - Results: - old: 22.6 ± 7.4 min., young: 13.0 ± 5.2 min. - “amount of fatigue” = torque reduction at failure equal for both - “kind of fatigue”: in young, faster increase in surface EMG amplitude perceived exertion higher, arterial pressure+heart rate higher Enoka, Duchateau, J Physiol 2008 Example 2: male vs. female Exp. A: - strength-matched men vs. women for 20% MVC in elbow flexors - Results: - no difference in max. duration of sustained contractions, 819.3 ± 306 s vs. 864 ± 391 s (+ same torque reduction at failure, same EMG patterns (bursts, amplitude), heart rate, arterial pressure) - intermittent contractions (50% MVC, 6 s contr., 4 s rest): women perform longer Enoka, Duchateau, J Physiol 2008 Example 2: male vs. female Exp. A: - strength-matched men vs. women for 20% MVC in elbow flexors - Results: - no difference in max. duration of sustained contractions, 819.3 ± 306 s vs. 864 ± 391 s (+ same torque reduction at failure, same EMG patterns (bursts, amplitude), heart rate, arterial pressure) - intermittent contractions (50% MVC, 6 s contr., 4 s rest): women perform longer Exp. B: - isometric contraction of knee extensors (25% MVC) - blood flow occluded: men and women similar (165±20 s, 180±20 s) (even though men were stronger) - not occluded: women sustain longer => interpretation: sex difference in metabolic pathways contributing to ATP production Enoka, Duchateau, J Physiol 2008 Pathological fatigue in disease Example: Myasthenia gravis - autoimmune disease, immune system produces antibodies against the skeletal muscle Acetylcholine receptor (AChR) => AChR needed to activate skeletal muscle cells with ACh - ACh receptors blocked (not activated) by antibodies => more ACh needed for the same force production => early fatigue by ACh vesicle depletion Pathological fatigue in disease Example: Myasthenia gravis - autoimmune disease, immune system produces antibodies against the skeletal muscle Acetylcholine receptor (AChR) => AChR needed to activate skeletal muscle cells with ACh - ACh receptors blocked (not activated) by antibodies => more ACh needed for the same force production => early fatigue by ACh vesicle depletion - diagnostics: - Simpson’s test: voluntary eye elevation for >30 seconds => early fatigue of eye muscles => ptosis (eyelids drop) and double vision - repetitive electrical muscle stimulation => decreasing muscle response (surface EMG) (“decrement”) Toyka, Neurology 2006 Thank you!