Medical Physics Lecture 7: Electron Beam Dosimetry PDF
Document Details
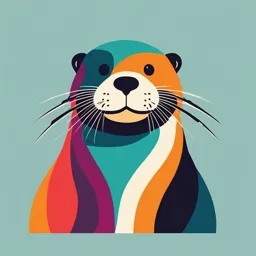
Uploaded by TriumphalHeliodor
Khalid Ibrahim Hussein
Tags
Summary
This document contains lecture notes on electron beam dosimetry, part of a medical physics course. It discusses topics such as electron mode, characteristics of electron beams, interactions with absorbing medium, and clinical considerations. The notes include diagrams and tables.
Full Transcript
M.Sc. MEDICAL PHYSICS Lect 07: Electron Beam Dosimetry Dr. KHALID IBRAHIM HUSSEIN Electron Mode ❑ Megavoltage electron beams represent an important treatment modality in modern radiotherapy, often providing a unique option in the treatment of superficial tumour...
M.Sc. MEDICAL PHYSICS Lect 07: Electron Beam Dosimetry Dr. KHALID IBRAHIM HUSSEIN Electron Mode ❑ Megavoltage electron beams represent an important treatment modality in modern radiotherapy, often providing a unique option in the treatment of superficial tumours. ❑ Electrons have been used in radiotherapy since the early 1950s. ❑ Modern high-energy linacs typically provide, in addition to two photon energies, several electron beam energies in the range from 4 MeV to 25 MeV. Dr. Khalid Ibrahim Hussein Electron Mode ❑ In electron mode the x-ray target is removed, scattering foils in place, and applicator in place to help restrict electron from leak out outside the treatment field. ❑ Electron beam are collimated by an applicator (cone) and cutout (insert). It is energy dependent, thin, and low atomic number (Not bremsstrahlung produce). ❑ The insert is placed close to the skin because the electron have a large spot size which result in geometric spread Cutout Cutout at at 95cm 95cm Dr. Khalid Ibrahim Hussein Electron Mode General shape of the depth dose curve ❑ The general shape of the central axis depth dose curve for electron beams differs from that of photon beams. Dr. Khalid Ibrahim Hussein Electron Beam Characteristics ❑ Electron beam central axis percentage depth dose curve exhibits the following characteristics: Uniform dose with rapid falloff Surface dose is relatively high (of the order of 80 % – 100 %). Maximum dose occurs at a certain depth referred to as the depth of dose maximum zmax. Beyond zmax the dose drops off rapidly and levels off at a small low level dose called the bremsstrahlung tail (of the order of a few per cent). Dr. Khalid Ibrahim Hussein Electron interactions with absorbing medium ❑ Electron beams are almost monoenergetic as they leave the linac accelerating waveguide. ❑ In moving toward the patient through: Waveguide exit window Scattering foils Transmission ionization chamber Air and interacting with photon collimators, electron cones (applicators) and the patient, bremsstrahlung radiation is produced. This radiation constitutes the bremsstrahlung tail of the electron beam PDD curve. Dr. Khalid Ibrahim Hussein Electron interactions with absorbing medium ❑ As the electrons propagate through an absorbing medium, they interact with atoms of the absorbing medium by a variety of elastic or inelastic Coulomb force interactions. ❑ These Coulomb interactions are classified as follows: ❑ Inelastic collisions with orbital electrons of the absorber atoms. ❑ Inelastic collisions with nuclei of the absorber atoms. ❑ Elastic collisions with orbital electrons of the absorber atoms. ❑ Elastic collisions with nuclei of the absorber atoms. Dr. Khalid Ibrahim Hussein Electron interactions with absorbing medium ❑ Inelastic collisions between the incident electron and orbital electrons of absorber atoms result in loss of incident electron’s kinetic energy through ionization and excitation of absorber atoms (collision or ionization loss). ❑ The absorber atoms can be ionized through two types of ionization collision: ❑ Hard collision in which the ejected orbital electron gains enough energy to be able to ionize atoms on its own (these electrons are called delta rays). ❑ Soft collision in which the ejected orbital electron gains an ❑ insufficient amount of energy to be able to ionize matter on its own. Dr. Khalid Ibrahim Hussein Electron interactions with absorbing medium ❑ Elastic collisions between the incident electron and nuclei of the absorber atoms result in: ❑ Change in direction of motion of the incident electron (elastic scattering). ❑ A very small energy loss by the incident electron in individual ❑ interaction, just sufficient to produce a deflection of electron’s path. ❑ The incident electron loses kinetic energy through a cumulative action of multiple scattering events, each event characterized by a small energy loss. Dr. Khalid Ibrahim Hussein Electron interactions with absorbing medium ❑ Electrons traversing an absorber lose their kinetic energy through ionization collisions and radiation collisions. ❑ The rate of energy loss per gram and per cm2 is called the mass stopping power and it is a sum of two components: ❑ Mass collision stopping power ❑ Mass radiation stopping power ❑ High energy electrons lose energy at a rate of ❑ 2 MeV/cm water. ❑ Depth of penetration is 0.5cm/MeV ❑ Or Energy/2 Dr. Khalid Ibrahim Hussein virtual source position ❑ In contrast to a photon beam, which has a distinct focus located at the accelerator x ray target, an electron beam appears to originate from a point in space that does not coincide with the scattering foil or the accelerator exit window. ❑ The term “virtual source position” was introduced to indicate the virtual location of the electron source. Dr. Khalid Ibrahim Hussein virtual source position ❑ Effective source-surface distance SSDeff is defined as the distance from the virtual source position to the edge of the electron cone applicator. ❑ The inverse square law may be used for small SSD differences from the nominal SSD to make corrections to absorbed dose rate at zmax in the patient for variations in air gaps g between the actual patient surface and the nominal SSD. Dr. Khalid Ibrahim Hussein virtual source position ❑ Typical example of data measured in determination of virtual source position SSDeff normalized to the edge of the electron applicator (cone). Dr. Khalid Ibrahim Hussein virtual source position ❑ For practical reasons the nominal SSD is usually a fixed distance (e.g., 5 cm) from the distal edge of the electron cone (applicator) and coincides with the linac isocentre. ❑ Although the effective SSD (i.e., the virtual electron source position) is determined from measurements at zmax in a phantom, its value does not change with change in the depth of measurement. ❑ The effective SSD depends on electron beam energy and must be measured for all energies available in the clinic. Dr. Khalid Ibrahim Hussein Dosimetric Characteristics of the Electron Beam Dr. Khalid Ibrahim Hussein Electron Beam Characteristics Dr. Khalid Ibrahim Hussein DOSIMETRIC PARAMETERS OF ELECTRON BEAMS ❑ Typical electron beam depth dose parameters that should be measured for each clinical electron beam Energy R90 R80 R50 Rp E(0) Surface (MeV) (cm) (cm) (cm) (cm) (MeV) dose % 6 1.7 1.8 2.2 2.9 5.6 81 8 2.4 2.6 3.0 4.0 7.2 83 10 3.1 3.3 3.9 4.8 9.2 86 12 3.7 4.1 4.8 6.0 11.3 90 15 4.7 5.2 6.1 7.5 14.0 92 18 5.5 5.9 7.3 9.1 17.4 96 Dr. Khalid Ibrahim Hussein Practical shielding application using Rp ❑ Shielding skin surface from electron beam treatment using electron beam of 9 MeV ( loses 2MeV/cm in water. ❑ The calculated required thickness of a shielding material using bolus is given by – 9MeV/2Mev/cm = 4.5cm of bolus. ❑ If we want to use Lead (ρ=11.34 g/cm3) ~ 10g/cm3 ❑ Then the required thickness of lead is 9MeV/2MeV/cm/10 =0.45cm Dr. Khalid Ibrahim Hussein Howe Work ❑If we want to used Tungsten shield for shielding eye from 6 MeV electron given to lower lid. What will be the best thickness of Tungsten to be used if we have two thicknesses available 2mm and 3mm. ❑Why we prefer always to use Lead instead of Tungsten? Dr. Khalid Ibrahim Hussein DOSIMETRIC PARAMETERS OF ELECTRON BEAMS ❑ Similarly to PDDs for photon beams, the PDDs for electron beams, at a given source-surface distance SSD, depend upon: Depth z in phantom (patient). Electron beam kinetic energy EK(0) on phantom surface. Field size A on phantom surface. Dr. Khalid Ibrahim Hussein DOSIMETRIC PARAMETERS OF ELECTRON BEAMS ❑ PDDs of electron beams are measured with: ❑ Cylindrical, small-volume ionization chamber in water phantom. ❑ Diode detector in water phantom. ❑ Parallel-plate ionization chamber in water phantom. ❑ Radiographic or radiochromic film in solid water phantom. Dr. Khalid Ibrahim Hussein DOSIMETRIC PARAMETERS OF ELECTRON BEAMS ❑ Measurement of electron beam PDDs: ▪ If ionization chamber is used, the measured depth ionization distribution must be converted into a depth dose distribution by using the appropriate stopping power ratios, water to air, at depths in phantom. ▪ If diode is used, the diode ionization signal represents the dose directly, because the stopping power ratio, water to silicon, is essentially independent of electron energy and hence depth. ▪ If film is used, the characteristic curve for the given film should be used to determine the dose against the film density. Dr. Khalid Ibrahim Hussein DOSIMETRIC PARAMETERS OF ELECTRON BEAMS Dependence of PDDs on electron beam field size. ❑ For relatively large field sizes the PDD distribution at a given electron beam energy is essentially independent of field size. ❑ When the side of the electron field is smaller than the practical range Rp, lateral electronic equilibrium will not exist on the beam central axis and both the PDDs as well as the output factors exhibit a significant dependence on field size. Dr. Khalid Ibrahim Hussein DOSIMETRIC PARAMETERS OF ELECTRON BEAMS PDDs for small electron fields For a decreasing field size, when the side of the field decreases to below the Rp value for a given electron energy: Depth dose maximum decreases. Surface dose increases. Rp remains essentially constant, except when the field size becomes very small. Dr. Khalid Ibrahim Hussein Electron Beam Calibration ❑Calibrate to 1 cGy per monitor unit at dmax for each energy. ❑10 x 10 or 15 x 15 cm2 filed size/ Cone ❑SSD configuration( 100cm to water surface) ❑Set output at refrence depth(dmax) Dr. Khalid Ibrahim Hussein DOSIMETRIC PARAMETERS OF ELECTRON BEAMS ❑ Unlike in photon beams, the percentage surface dose in electron beams increases with increasing energy. ❑ In contrast to photon beams, zmax in electron beams does not follow a specific trend with electron beam energy; it is a result of machine design and accessories used. Dr. Khalid Ibrahim Hussein DOSIMETRIC PARAMETERS OF ELECTRON BEAMS PDDs for oblique incidence. ❑ Angle of obliquity α is defined as the angle between the electron beam central axis and the normal to the phantom or patient surface. Angle = 0 corresponds to normal beam incidence. ❑ For oblique beam incidences, especially at large angles the PDD characteristics of electron beams deviate significantly from those for normal beam incidence. Dr. Khalid Ibrahim Hussein DOSIMETRIC PARAMETERS OF ELECTRON BEAMS ❑ Percentage depth dose for oblique beam incidence Dr. Khalid Ibrahim Hussein DOSIMETRIC PARAMETERS OF ELECTRON BEAMS Depth dose for oblique beam incidence ❑ Obliquity effect becomes significant for angles of incidence α exceeding 45o. ❑ Obliquity factor OF (,z) accounts for the change in depth dose at a given depth z in phantom and is normalized to ❑ 1.00 at zmax at normal incidence = 0 ❑ Obliquity factor at zmax is larger than 1 Dr. Khalid Ibrahim Hussein DOSIMETRIC PARAMETERS OF ELECTRON BEAMS ❑ The output factor for a given electron energy and field size (delineated by applicator or cone) is defined as the ratio of the dose for the specific field size (applicator) to the dose for a 10×10 or 15 x15 cm2 reference field size (applicator), both measured at depth zmax on the beam central axis in phantom at a nominal SSD of 100 cm. Dr. Khalid Ibrahim Hussein DOSIMETRIC PARAMETERS OF ELECTRON BEAMS Therapeutic range ❑ Depth of the 90 % dose level on the beam central axis (R90) beyond zmax is defined as the therapeutic range for electron beam therapy. ❑ R90 is approximately equal to EK/4 in cm of water, where EK is the nominal kinetic energy in MeV of the electron beam. ❑ R80, the depth that corresponds to the 80 % PDD beyond zmax, may also be used as the therapeutic range and is approximated by EK/3 in cm of water. Dr. Khalid Ibrahim Hussein DOSIMETRIC PARAMETERS OF ELECTRON BEAMS Profiles and off-axis ratio ❑ A dose profile represents a plot of dose at a given depth in phantom against the distance from the beam central axis. ❑ Profile is measured in a plane perpendicular to the beam central axis at a given depth z in phantom. Dose profile measured at a depth of dose maximum zmax in water for a 12 MeV electron beam and 25×25 cm2 applicator cone. Dr. Khalid Ibrahim Hussein DOSIMETRIC PARAMETERS OF ELECTRON BEAMS Profiles and off-axis ratio ❑ Two different normalizations are used for beam profiles: The profile data for a given depth in phantom may be normalized to the dose at zmax on the central axis (point P). The dose value on the beam central axis for z zmax then represents the central axis PDD value. The profile data for a given depth in phantom may also be normalized to the value on the beam central axis (point Q). The values off the central axis for z zmax are then referred to as the off-axis ratios (OARs). Dr. Khalid Ibrahim Hussein DOSIMETRIC PARAMETERS OF ELECTRON BEAMS Flatness and symmetry ❑ According to the International Electrotechnical Commission (IEC) the specification for beam flatness of electron beams is given for zmax under two conditions: ❑ Distance between the 90 % dose level and the geometrical beam edge should not exceed 10 mm along major field axes and 20 mm along diagonals. ❑ Maximum value of the absorbed dose anywhere within the region bounded by the 90 % isodose contour should not exceed 1.05 times the absorbed dose on the axis of the beam at the same Dr. Khalid Ibrahim Hussein depth. DOSIMETRIC PARAMETERS OF ELECTRON BEAMS Flatness and symmetry ❑ According to the International Electrotechnical Commission (IEC) the specification for symmetry of electron beams requires that the cross-beam profile measured at depth zmax should not differ by more than 3 % for any pair of symmetric points with respect to the central ray. Dr. Khalid Ibrahim Hussein CLINICAL CONSIDERATIONS Dose specification and reporting ❑ Electron beam therapy is usually applied in treatment of superficial or subcutaneous disease. ❑ Treatment is usually delivered with a single direct electron field at a nominal SSD of 100 cm. ❑ The dose is usually prescribed at a depth that lies at, or beyond, the distal margin of the target. Dr. Khalid Ibrahim Hussein CLINICAL CONSIDERATIONS Dose specification and reporting ❑ To maximize healthy tissue sparing beyond the tumour and to provide relatively homogeneous target coverage treatments are usually prescribed at zmax, R90, or R80. ❑ If the treatment dose is specified at R80 or R90, the skin dose may exceed the prescription dose. ❑ Since the maximum dose in the target may exceed the prescribed dose by up to 20 %, the maximum dose should be reported for all electron beam treatments. Dr. Khalid Ibrahim Hussein CLINICAL CONSIDERATIONS Isodose distributions ❑ Isodose curves are lines connecting points of equal dose in the irradiated medium. ❑ Isodose curves are usually drawn at regular intervals of absorbed dose and are expressed as a percentage of the dose at a reference point, which is usually taken as the zmax point on the beam central axis. Dr. Khalid Ibrahim Hussein CLINICAL CONSIDERATIONS Isodose distributions ❑ As electron beam penetrates a medium (absorber), the beam expands rapidly below the surface because of electron scattering on absorber atoms. ❑ The spread of the isodose curves varies depending on: Isodose level. Energy of the beam. Field size. Beam collimation. Dr. Khalid Ibrahim Hussein CLINICAL CONSIDERATIONS Isodose distributions ❑ A particular characteristic of electron beam isodose curves is the bulging out of the low value isodose curves (