lec4.docx
Document Details
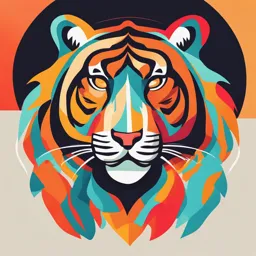
Uploaded by SafeMahoganyObsidian
Full Transcript
I'm exhausted. It's a little easier getting microbial memes. There's more keywords and topics, you know. is a little more niche. or have to like, literally create them myself. Yeah. which is not possible. And okay. okay, guys. so just a couple of things before we start. Can you hear me on the on the...
I'm exhausted. It's a little easier getting microbial memes. There's more keywords and topics, you know. is a little more niche. or have to like, literally create them myself. Yeah. which is not possible. And okay. okay, guys. so just a couple of things before we start. Can you hear me on the on the speaker phones. or you just hear my voice. Kind of both has have the recordings. Has anyone tried the recordings? That accounts they're good. Okay, good. Good. Good. Okay. So before I start today, just a couple of things. So one thing I sent an email to everyone. But and in case you haven't already responded about it yet. please consider it. It's asking for anyone who is able to be a volunteer notetaker for people in need of help for notetaking. So if you are interested in able, please send me an email, and I'll connect you with those students. Any other announcements. Again. Midterms believe it's February sixth, and March seventh, I believe so just, you know. Keep mind of those things, and I'll be clear about what will be on actually midterm one versus what will be punted to? You know the phase of the class that midterm 2 will cover. Okay, any other questions that people have so far material or just things about the class seems kind of. And shepherd raise us a little bit. Okay? Another. So just to get an idea if some of you might have been. you know, wandering off into this video that you see here. It's you know, a video that's taken of a 7 day old Chick embryo. It's not through time. It's just one singular time shot snap and it's using really cool methods to do 3 dimensional reconstruction of you know whether it's gene expression or protein localization of something within the embryo. So here I don't remember exactly what the marker is. But basically, this is a 7 day old chick. And you see the nervous system essentially of the chick. So everything that's highlighted in, you know white basically being highest expression and kind of intermediate expression, being kind of yellowish. And you can see, you know, a lot of the images I've showed you, Macobulji, if you click that class. And in other classes you've seen when it comes to gene expression. It's kind of this two-dimensional image, and you can kind of see where in space and time. in fixed tissue, the expression of some transcript is, or the localization of some protein is. And that's very informative, super also really fascinating and super cool. But just so, you know, there's lots of really awesome, amazing and fascinating and high resolution techniques that have been developed over the last few years that have enabled us to visualize and describe again, you know, spatial temporal aspects of gene expression. In really, truly 3 dimensions. So you can fully see how genes and proteins are functioning towards constructing tissues in the context of Donal biology. But also, you know, methods like this have been used in many other disciplines as well, not just restricted to development. Also, some of you. I came and asked after class. You know, II mentioned this similar biology. But just to reiterate, you know, we're interested in people joining the lab. There's various degrees of how much time investment and also how long term type of investment in lab opportunities if you are interested, especially for those who haven't heard this Mini kind of announcement haven't taken one of my classes yet again. My, we have a lab here at U Ottawa. And what's happening? Screen here. Let me see. I fix that might affect a lot of slides. Comp. Well, it's rajukumarlab.com. Oh, yeah. So it's right here that was underneath. So rajukumarlav.com just my last name lab Com. And there's a join us. Tab. click it. There's some information not just for joining our lab. There's some useful information, General, for undergrads thinking about joining a research group. So if you're interested, feel free to click. Oh, also Every now and then there'll be some interesting points that were brought up after class that figured I'd bring up again, you know, in the next class one student had asked me we were talking about fossils, if you remember correctly, from some of the last slides, and of course, going from one slide about fossils. future slides. Later, talking about DNA and dinosaurs all kinds of stuff, and so inevitably. You get questions related to, you know. Can you get DNA out of fossils right? And although you can't actually, in the last half a decade or so, scientists have developed methods to actually visualize, using really cool different types of microscopy and lighting methods to actually visualize the protein structure in fossils like rock fossils. So you can actually kind of reconstruct to some degree the type of protein overall protein structures that fossils had for certain certain types of structural proteins. And then the question was, Well, what about like? Say, for example, amber fossils right? Little bit more prefers for preserve specimen also. No. you can't get DNA out of that. But you can do really cool things now with stuff preserved in amber fossils. It's not just restricted to insects. Obviously they're the most common. Well, Inverter, it's the most common thing you'd find in in amber fossils, in the context of animals. But you can find whole vertebrates in fossils. You know I have. I have a friend who was studying actually an entire gecko that was preserved in an amber fossil from millions and millions and millions of years ago. And the cool thing about that is, it's not just that you can see these different organisms. Of course, the amber fossil and image them using techniques like the one I just showed you in that video. We've now developed methods that you can actually use. Micro ct, so micro so think about Cts that hospitals do. So. There's micro versions of that Unknown Speaker 00:07:21 and you can use micro Ct approaches or Nano Ct approaches actually Rajendhran Rajakumar 00:07:26 to actually go into the amber fossil effectively and see all the layers you can see inside the specimen, and you're able to reconstruct or say it was the gecko, for example, their entire skeleton, and even some soft tissue as well in their bodies. I saw, you know, my friend, show me last year there was ants preserved in fossil in an amber fossil. And there was a cocoon. And you can actually see the pupae in the cocoon using these micro Ct type methods so non-invasive, non-destructive methods of actually studying the structures, including internal anatomy and internal structures of things preserved in fossils. But then, of course. I saw where the question was going, and it was very drastic, part kind of thinking and getting at like, can you get DNA, you know, from extinct animals, and in fact, you can. So there are animals that are able to preserve their DNA in and their cells for that to some degree in a fossilized, extinct type form. So, for example, you know, there's famous specimens found in the Arctic. I want to say is more on the Siberian side. But I don't remember exactly it was, but the species is Siberian woolly mammoth. So those of you don't know wooly mammoth. They're like these ancestors of elephants. These big, hairy super hairy elephants from the north. and although they're probably distributed more across Europe and North America, too. and actually they found a woolly mammoth, entire body specimens preserved in the ice, and they isolated and, like the tissue like, was incredibly preserved, and they were able to isolate cells. DNA tissue everything from the specimen, and since then, however many years it's been, they've done so many cool things and study, including even sequencing the genome of the bully Mammoth, which is crazy to think of. but it's true. Imagine the woolly mammoth genome exists and published. You can go and check it out compared to elephants, for example. But it's more sci-fi than that. So they were able to actually isolate nuclei from these preserved samples. And there's labs across the world, including George Church's lab at Harvard Medical School in the Genetics Institute Lab. It was like, right right below the lab that I was at in my second postdoc. the George Church lab. They're trying to basically take the nucleus of the woolly mammoth and actually insert it into oocytes of elephants. whether it was African or or Indian elephant. I don't remember exactly what they're doing now. But just let's just say elephant. and and then use an elephant surrogate mother to try to basically resurrect the woolly mammoth it's called like de extinction, as they call it. How obviously controversial! What is the relevance like? What are we gonna learn from? You're gonna have, you know is the whole point. Just to have zoos with Willy mammoths running around, I mean, effectively, like, you know, kind of the Jurassic Park Jurassic world thinking. I don't know. You feel free to go check websites out is pretty fascinating at the very least. Certainly. Foo for thought Borderline Science Fiction to reality. So yes, coming to that, you can do some stuff. And you could study the I mean, there are some really obvious basic fundamental biology. You can learn from these specimens, too. Like I said, you have their whole genome sequence. Imagine how informative that was to for just elephant evolution in general, but also, you know, very fascinating that you can get genomes of extinct animals. I mentioned my microbology class. The same thing happened with the Neanderthal genome, and how important that was for biomedicine. and won the Nobel Prize last year. But in any case just interesting question that came up after last lecture. so just to reiterate kind of a slightly different version of the table that I had in the last lecture. But think of, consider them both as study tools for understanding these 3 modes of specification also related to some questions that people asked after class. just to just to kind of recap it. You have 3 modes of cellular specification during key phases of development. polynomial specification, conditional specification and syncytial specification. This one is very hard to imagine without seeing it happen. So which you're gonna see in a few slides from these 2 the names kind of lend a little bit of insight and or clues into what what they're trying to represent. So autonomous specification is where cells regardless of their external environment, physical, chemical environment, they get to a point where once they're specified, they're locked in that they are in an autonomous fashion. They're specified and it's influenced, as you see here. by the localization of transcription factors in the cytoplasm and inherited by the cells containing that cytoplasm, so say we were looking at the 4 cell stage of an embryo. the cytoplasm. In each of those 4 cells they were derived from the 2 parental cells. Right? So 2 parental cells had to set their own cytoplasms, and those cytoplasms were divided amongst and contributed to the cytoplasm of those daughter? 4 cells, right? So depending. So as if you were one of those 4 daughter cells. Which of those 2 parental cells did you actually come from? Are you the result of a cleavage event of them? And that tells you that's where your cytoplasm is derived from. Why, that's important is because those 2 daughter cells originated from one, the original fertilized egg, right? The one cell stage, and that has its own open. You know, cytoplasm. But in that open cytoplasm? Is not this equally distributed? Equal distribution of proteins and micro rnas and different rna molecules and various other signaling factors? They're actually asymmetrically distributed? Even within the cytoplasm of that single cell. and depending where the cleavage event happens. Now, when you get to the 2 cell stage, one of those 2 cells have some aspects of the cytoplasm of that first cell and the other cell, the 2 stage cell stage. We'll have another aspect of the cytoplasm. and that already is creating an asymmetry of distribution of these molecules. So these resources that will instruct what those cells will specify, what they will become. And we looked at it, and techniques in previous lectures of how do you trace, following these cleavage events? What cells give rise to which cells. well, along with the physical nature of, you know these cells divide and see them. What they're becoming. Think about what's inside, too. Don't just think about these spheres, these cells, these, you know, circular spherical type things dividing. And you know, lineage tracing, just watching where they go. It's also think about what's inside them and how what's inside them is being distributed throughout the entire sphere. That is the embryo that eventually very soon will become thousands, tens of thousands, hundreds of thousands of cells. Okay. So in any case, punish specification. You're you're watching individual cells as these cleavage events are occurring. And after they're they are, they're and they're influenced in terms of this specification by the factors within. They're influenced from the within rather than from outside conditional specification. In contrast to, you know, thinking about the word autonomous. It's really dependent, context-dependent. It really depends on the condition the cells are in. And by condition, I mean the micro. So micro environment. So what cells are they touching? What is the physical environment? Are they moving? Are they migrating with other cells, too? Are they moving as individual cells? How is that movement, changing the physical contacts they're making with their cellular tissue environment. And then, of course, in parallel to all of these physical contacts and interactions. and imagine. Remember, I'm not going to do a whole cell bio lecture today. But you know, cells contacting each other. They're communicating through extracellular matrices, factors, adhesion molecules which we will cover a little bit. Actually in, you know, in the coming slides and lectures. But also even mechanoreceptors on the surface of cells, that when they're perturbed, they actually relay through that physical perturbation cell signaling pathways within a cell can influence transcription within those cells and all kinds of different things. So physical environment can have a profound impact. Many different cellular contexts. And when I'm talking about conditional specification. that's what I'm referring. That's one of the 2 things I'm thinking about physical. And then, of course, more intuitively, the chemical environment. Of course. you know, transcription factors, hormones, paracrine factors, whatever molecules that are actually floating around in that micro environment of the embryo in close contact. High density around you as a cell can highly influence what you, how you were specified. What you become. So that's conditional specification. Is that pretty clear? That's the difference between the 2. Okay, good. Because that'll really help you think about specification as we're getting to more complex examples. And then you have this weirdo syncic specification. So transfusion factors form gradients within a large cell contains many nuclei, not not many cells in the number of many nuclei. Okay. the nuclei express genes depending on the ratios of these transcription factors that are distributed through this entire cell. So it's a little. It's quite different than these other 2. It's not about cell-cell communication and distribution of cytoplasms across cleavages of cells. In these early cleavage events. There is no cleavage events. The it's nuclei that are actually dividing and propagating. And where they are in this large one cell stage means it influences. We're how close, approximately they are to some molecules in that cytoplasm, in that cell versus other nuclei that might be more further away from certain types of molecules again, very hard to imagine without seeing it, which we will look at very soon. Okay, so we talked a lot about autonomous specification examples. Last class, we'll get right into conditional so example of conditional specification. so we're looking at amphibian development developing amphibian here. I'm almost certain it's a frog. And you have at the blastial stage at this point. So imagine these cells that are highlighted in kind of reddish here, and imagine in a typical embryo that's not perturbed by external forces like by a researcher. those cells, once they undergo their migration, and where they have to go and what they proliferate into give rise to. Eventually, they might contribute to say cells within the back of the of the adult. Okay, so imagine you do no kind of manipulations. Normal development happens. Those cells that are marked here. They would give rise to effectively like this part of this tadpole. Right now. what happens if you take those cells and you transplant them either in the same embryo or in a different embryo in the region of the embryo that normally would give rise to the eventual, you know, tissue in the ventral side of the tadpole, like the belly of the tadpole. Will you get back cells forming in the belly, because they might, you know, moving, and, geographically speaking, that might influence where they end up. So maybe in the ventral side. But is it like back cells, types in the ventral side. So dorsal cell types in the ventral side? Or are you actually having those back cells becoming belly cells, so to speak. and what happens is in a conventional sense is that they might go. They might migrate to the ventral side, influenced by now you transplanting them in a totally different region of the embryo. But they become they're influenced by and become well. Let me just say, break that down. They're influenced by the other ventral cells that they're now being introduced to. Unknown Speaker 00:19:15 and that influences what they become which are belly cells or eventual cell types. Rajendhran Rajakumar 00:19:19 So they change their their their identity effectively. And that's an example of conditional specification. Another example of conditional specification is, if you were to say you take a glass needle, or you take some forceps or something, and you just remove a whole chunk of cells off an embryo. You see, kind of have like a invite right under this beauty. So what happens? Does the animal develop with some missing structures? Well, it turns out it doesn't. As you can see in this image here. It's just fine. And what happens is that the other cells learn the adjacent regions of the cut where the wound is. they will start to contribute to regenerative processes and also redifferentiation processes so that they can now start to become and compensate for the cells that were removed. basically filling in that gap effectively. And so these cells now as groups, so not just thinking about individual cells, but sees cells as kind of a group in concert with each other. Now, due to this condition condition, which is like basically this injury that the embryo experienced compensate. And now they research, can, you know, compensate for that injury. Okay, again, 2. Transplantation example and a removal example. Experiment. Example. To give you an idea of what? How, what conditional specification is capable of doing. Okay? So now, probably, arguably, the most profound experiment that has been done in embryology when it comes to understanding specification, and arguably one of the most important pioneering experiments that has ever been done is this experiment that you see right here? So basically where to start. So you have a sea urchin embryo that you see here and again, sea urchins you're going to see throughout the class extremely important model organism for developmental biology. having a lot to do with the fact that in the 18 hundreds people were usually sea urchin embryos right off the bat to start asking basic questions about cleavage cell specification, what cells become? Where do they go? That kind of thing? Unknown Speaker 00:21:33 And huge reason for that which I might have mentioned a little bit before, but just to reiterate that Rajendhran Rajakumar 00:21:37 it's very easy to see the cells. And they're big, and they're very, very easy to manipulate and very easy to culture. So they survive really well, so on and so forth. So they're just really great in the lab. So you see the spore cell stage. Now, if you remove any like external envelopes that these 4 cells are in that are protecting them in the marine environment, of course, that they're existing. And you remember, these are Co chains. We're talking here. And you were to separate each of these 4 cells in isolation. So you would think that again, people. And up to this point autonomous specification was the rule of the world of of the land, so to speak. That's what people thought was. The only thing that happened with embryos was autonomous specification like the examples we looked at the other day. Right? But in this case each one of these cells were capable of reconstituting in an entire sea urchin larvae, all structures intact the whole, the whole whole frickin thing was basically recapitulated with each one of those cells. which is crazy. Because again, when you're tracing these cells and you're watching them divide into further further generations, they eventually contribute to different aspects of the embryo. But when you separate them at these kinds of stages. they're able to recapitulate the entire thing. Now, of course, they don't look identical. There's some kinds of asymmetries and some of the patterning. And if you were to think about these to scale which they're probably approximately to scale. you know normal Plutus larval stage of the sea urchin embryo versus these ones that are a result of this experimental manipulation. They're a little smaller. Okay? But otherwise the entire body plan is basically intact. So let me just make sure I went over the key things here. Each cell can form a smaller but normal version of what's supposed to happen. yeah. Oh, they are all drawn to same scale. There you have it. So. The person who did this name's Hans Driesch. or a German embryologist in the late 18 hundreds. and he had done a series of other experiments, and they're all inspired by people who preceded him. Weisman, August Weisman, who actually is the pioneer of the germ theory, which I'm not going to get into today. But maybe we'll talk about in stem cells. But in any case, and then rue and many other really important embryologists in the in the mid to late 18 hundreds. that I've really been showing autonomous specification being again the the go to understanding cells are locked in. They're programmed already to become what they need to become. They don't have this kind of capacity, this potential to literally make the entire organism again, each and of themselves. So this conditional specification. And so just to give a little historical tangent, I'm not going to read this. All you guys can have it in your notes, but basically in a nutshell. Hans Driesch. He was really interested in the physics and the mathematics of embryology, which is again showing you how how ahead he was of his time and thinking about that, because we're only really addressing figure out methods and addressing them. The biophysics and mathematical modeling of embryology like now. And so he was very much into thinking about the embryo as its program, almost like how we would think of automatons or robots. Now like a circuit, a program that unfolds. Okay. but when he did this experiment he lost his shit so much that he just quit science and became a philosopher. He's like screw this. I'm out. and he just he's gone. He quit. He was like literally the best embryologist of his time he was one of the best scientists of his time. Remember, this is the time of like the Origin of Species, Charles Darwin. Biology, as an entire discipline was going from natural history into these new realms genetics was starting to Mendel time. This is the time of Mendel, actually more than even maybe older days of Darwin, the time of Mendel, and and a lot of things were going down. And imagine if this dude had stuck around and kept was inspired by this, and just kept going with it. Let the data speak for what it is, and and and let it influence his future experience. He was like Nope, and he just like dropped the mic, and he was out, became a philosopher, started studying all kinds of other random things. I kid you, not I kid you not. But that's how crazy this was. And imagine you just take you take this embryo. It's dividing, and whatever you take a cell out and like it just makes the entire thing again. What the heck is that that's literally what he was seeing before his eyes. And it's not just that he saw that happen. It's that it was in stark contrast to what people were doing in other model emerging model organisms in develop biology. The ones I talked about last class for examples of autonomous specification completely. You wouldn't. This wouldn't happen in those organisms, but it did in the Sea Urchin. It does in many other organisms, too. And again, this is the extreme version of conditional specification where you can. Each individual cell has this capacity, yes, question what they need to become. But there's this idea of well, so here's the question, what do they need to become? So? If you remember one of the last, some of the last slides of last class you had this certain self stage of I don't remember what what it was. It might have been a tunicit, but in any case, if you were to take one of those cells depending on where they were when you isolate, some became the ectoderm, and then you isolate another one, and they'll become, you know, the mesoderm mesenchym, and was the other thing probably contributing to some of the endoderm or something like that. So they had already start to. And as it's going a little bit further, they're already starting to that need the qualification of that that point they are already locking into contributing to certain lineages. Remember how we had a slide, whereas, like, I look like a tree and was like 4 cell stage 8, cell stage 1632, 64. Here's each of these 64 cells, what they become, and they'll become exactly the same thing every single time. Right? We can trace exactly their history back to the origin. Well, in this case, and if you isolate them they'll still become one of those very specific. You know lineages right in this case completely different. And that's what I'm saying. Why was so shocking? Is that in this case you isolate cells at a similar stage is what you saw there like 4, 8 cell stage whatever, and lo and behold! It recon recapitulates the entire animal, each of the cells rather than just key portions of the animal, like just the muscle and some of the endoderm, or maybe, and like the gut, or just the nervous system, and some of the skin, or something like that. Okay? Yeah. Great question. Yes. Unknown Speaker 00:28:18 itself. Rajendhran Rajakumar 00:28:21 Yes, yes, yes. So these individual cells. They don't just go from this and start like morphing into this crazy sea urchin larvae. They now the cleavage events still continue, they divide, divide, divide, divide, divide, but it's just that their daughter and granddaughter and sort of so on and so forth, cells that emerge from them are capable of making the entire animal rather than just a sub part of it. So before this was 4, it was one. And so imagine, let's pretend these this cross lines, aren't there? Imagine this is once on. Well, also, let's imagine these nuclei, aren't there as well? Okay, there's just one nucleus, one cell. Okay, in that one sphere. somewhere at the top are some factors. some on this side, some on this side, some on that side. I'm really simplifying, of course, right? When the division occurs, this cell has a certain fraction of those factors that's different than what the fraction of factors that this one has. And so already, at this stage, they're starting to divide some factors. Right? And that's that's autonomous specification is a huge part of that. I mean, that is a ton. I mean, actually, that is really autonomous specification. That's happening. Or let's not say autonomous specification. But that's that's the distribution of molecules that happens in every embryo where, in autonomous specification, it is highly influential. It locks things in it's the dictation of what happens while in these embryos, although there are asymmetries of distribution of factors, it doesn't lock them in. They're still capable. They saw the plasticity, the potential the stemness, as we'll learn about stem cells in soon, lectures to actually be able to recapitulate all, all. What would have happened if they had all of those factors as normal. Yeah. okay? Great question. Yes. equally Unknown Speaker 00:30:22 self-organ. Rajendhran Rajakumar 00:30:23 Yes, you will accept Unknown Speaker 00:30:25 the beginning. Rajendhran Rajakumar 00:30:27 so it's that. Well, so there's I guess there's kind of 2 ways to answer this question. Let me think of what's the simplest? basically, they're getting influenced by no cells anymore. So the influences that would have caused this one to boot, to give give rise to something because of these 3 cells impacting it are this one being more influenced by its contact with this one and this one, then say this one. And of course these are 4 cells. Imagine they're 8 and 16. They're starting to become some movement, too. So now it's like you remove the cell. And they no longer have these key, supportive types of and also constraining types of interactions with their cellular neighborhood. And so now they're like. I can do anything, basically. Okay, while you do the same isolations in an autonomous specification condition. They aren't like I can do anything. They just become what they were ought to become. Okay, make sense. That is also a great question. Yes. Unknown Speaker 00:31:24 smaller. Rajendhran Rajakumar 00:31:27 Yeah, I actually don't know. II have no idea. And I'm not even sure if there, if it's totally been elucidated it even in sea urchin systems. Yet even to this day. But again, the question for those of you didn't hear is, if you, you know, once you do this, these division experiments where you separate the cells, allow them to develop on their own? Why are they smaller. but otherwise reconstitute the shape and morphology of the normal, untreated one? I'm not so sure it could be a little bit of of a numbers game of now these cells, although they were able to recapitulate what and recreate the neighborhoods that they had when there are 4 cells. And beyond. maybe during that process there's a trade-off of the allocation of resources of molecules within the cytoplasm, including molecules, not just for patterning the body, but also for growing the body. so that could be something to do with it. But I actually that's a guess. That's my best guess. I don't know for sure, and I don't think really anybody does. Yes. I don't know if I wrote this here, let me actually double check. In my known notes. But yes, it would. You know what? For the sake of time I won't check my notes. But yes, it it can. It can happen to add at later stages, not a whole lot later, but to certain degree, later stages. Yes, just super clean cut at stages like this. The key takeaway is that we need to separate cells when they're at the 4 cell stage, or if you separate cells at the 8 cell stage. If an organism is in the category of conditional specification, those separated cells have a lot of potential. Maybe that potential will eventually start to Peter off when many, many cleavage events under fold for that organism. But when you compare that to organisms that are autonomously, autonomously specified, whether you isolate cells at the two-cell stage, or 4 or 8 or 16, they have way less potential than organisms, the cells of organisms that are conditional, so that relative Dicot, like distinction. That's the most important thing to take away and thinking about those 2 categories. Okay, yes. yes, exactly. yes, precisely so. Again, like thinking about it, and from the perspective of stem cells, are they as totally potent as cells that are from organisms that are under exhibiting conditional specification? No, they're not nearly as totally potent as the ones that you see here. Yes, exactly, which is crazy. When you think about that, it's quite profound that so early on certain organisms. You know, cells lose that incredible stemness degree. Now, it's not to say that they can't. They don't have the capacity to make many different things right like, you know, you look thing about the tunic example. The other day some of the that cell will contribute to something as distinct as the muscle versus say, the gut. Okay? So it can make muscle cells or gut cells like it's great, great, great, great, great, great granddaughters, right? But they contribute. And so that cell, if you were to isolate that you give it muscle conditions, factors, it can influence it to become eventually give rise to muscle cells versus gut factors to gut. But thing is, you can't give it factors or an environment that are more actodermal or some of the other cells that what they would have become it wouldn't really it. They're already locked in while these cells. man, you don't even have to give them any conditions. They're just free for all they have total stemness, and they recapitulate the whole animal. Yes. it! It kind of depends. And actually, maybe they'll punt that to when I actually talk about twinning and stuff like that in later lectures, it's better with a visual, that one. So maybe if you have more questions, we'll keep going it also. So future, several slides might also help you think about some of this stuff. Okay, except for these slides. So these slides just mix it up completely. Everything. We just worked out all the logic we just thought about here in index completely shatter that to bits. Okay, what they do they do something way more radical that you really have to take a second to think about what's actually happening even myself. When I was learning about multiple biology. It was really hard for me to grasp one. It was not explained very well. 2 like, I don't know. It's just. It's way different than the kind of examples you've seen so far. So imagine. So here you have beautiful like Nu, the nuclei of these drosophila monogaster embryos have been stained so that you can actually see the nuclei of of the of the embryo. Okay? And over here is kind of a cartoon reconstruction of some of those stages. Right? Okay. so, so, actually, this is, this is one particular stage stage 13. So what? So this here. I'll talk about it in more detail in. In a minute or 2. So let's let's just focus on this leftmost column over here. So you got sperm fertilizes an egg boom. First, my tag division and a couple more divisions and stuff starting to happen. Okay, within this new zygote form of zygote. What is that stuff? Well, it's not. This sphere divides into 2, divides, into 4, divides into 8. Like all of the other organisms we've talked about in more detail so far. Instead, it just stays the way it looks. But inside the nuclei. And you know the skipping steps, you know, cycle 3 cycle 7. Obviously the nuclei inside are what are actually dividing So so these are so still frames from a time last movie with developing the software, remove nuclei that are pre mitotic blue and actively dividing in mitosis purple. So that's what you see over here. But the important thing, maybe the the note that I was looking for isn't quite over here. Oh, yeah. So this part over here nuclei are dynamically ordered within the sensation of the early whoops, early embryo through cyos structures that actually keep them together. So again, you've got nuclei that are dividing chromosomes. So this DNA replication things that you normally would think of happening in cell cycling except you don't have cell membranes forming a cytokinesis. You just have nuclei. They're expanding. One becomes 2 becomes 4 becomes 8, and the nuclei are actually dividing and rather than forming cell membranes around themselves to separate each other as individual cells, nuclei and individual cells. You have cytoscle elements which I'm going to show you in the next slide that basically form like a skeleton like a protein, cytoskoskeleton, or framework or scaffold that keeps them separate from each other within the larger embryo. So at the beginning you'll have some nuclei that are within the internal part of the embryo. and there's whole regions of that zygote of that sphere that does not have nuclei distributed within it. But don't you don't have to wait too long until you know you get to subsequent cycles, and then, all of a sudden, you've got tons of nuclei within the embryo So once it happens, once you have nuclei from the anterior to the posterior of the fly embryo. Then they do what's called nuclear migration. So they actually migrate to the surface a good fraction of them. And so now they're the majority of them are actually on the periphery. Some of those will migrate to the posterior in a very specific posterior. Most region, and those are actually nuclei that are the nuclei of the pulse cells which contributes to the germ line the germ cells of the embryo of the animal. So what will be their sperm cells or egg cells. And when they become adults. So you've got cells nuclei moving to the to the periphery. Some stay in the middle Unknown Speaker 00:39:18 and Rajendhran Rajakumar 00:39:19 You have a couple more divisions. After they've migrated to the periphery to kind of like. See them? They're more numerous compared to this one here, but they're still kind of all along the periphery. And then the key moment happens. Cellularization happens. All of these cells off of the court off the periphery membranes start to form around the nuclei. And now they're separated by cellular interface so completely different than anything you've learned. Probably even in so I mean. Maybe you talked about flies and statistical you know formation in flies in your cell biology classes. I'm hazarding on the on the side of I highly doubt it. Also, partly because it's quite mysterious in terms of a lot of aspects of how it's happening and what happens when you perturb different things. but in a differentobiology class it's everything, because flies are one of is the most important genetic model in the world for the last. God knows how many decades. and understanding these key phases we have to otherwise, how the heck are we going to use it as that strong model where we can tink, tinker with genes and gene networks, and and see how different molecules influence cells and movements, and what they become, and so on and so forth. so on that note. it's all about the nuclei rather than the cells when it comes to syncytial specification. Now, what's happening at the cell cycle 3, it's sorry cell cycle 13. So pre-cellarization they haven't. They haven't separated by membranes yet. So this is like the most mature embryo in terms of how many nuclei have that have divided. But cellularization has not happened yet around those nuclei. So you have this embryo and compare this structure to the embryo you had, you know, very early on. So as these nuclei are expanding, they're dividing, and they're separating and moving within the giant, the giant cell that is the zygote at this point. That's the nuclei. But there were molecules dumped into the embryo that came from molecules that were actually in the sperm coming from the father and molecules that duringogenesis that were pumped into the egg. that's coming from the mother. Okay? And so these factors then include many different things, transcription factors, microns, all kinds of different molecules. Those are not. Again, as I kind of mentioned earlier, they're not equally distributed in this structure. There are some molecules that are only over here, some that are only over there, and more generally. especially these early phases. There's some molecules that aren't just like in Place X or place y like the at very tip of one end or the very tip of the other. They actually form gradients from different poles of the embryo, which I'll show you what I mean by gradients in a few slides from them. So the molecules and the and the nuclei are being distributed asymmetrically differentially throughout the entire embryo. And when cellularization happens at this stage cell cycle 13, then all of these now finally, cells within the embryo, they have completely different distributions of molecules within their membrane. and that completely influences the nuclei in those now newly formed cells. How those nuclei behave, how their genomes are actually activated and inactivated in different aspects of of its genome, to create its own proteins, its own transcription factors, its own microns, its own molecules within each one of these now cells that you see in this, you know, cycle 14 embryo, that now have membranes along this periphery. You see the pulse cells over here. So cell cycle 13. So what's happening in this entire column here? So this column? Here again, you see, cells in blue are prem mitotic and cells in purple are dividing and undergoing mitosis. And this is this is these are 5 images that are taken. of an embryo that's still alive. And there you. There's transgenic. So transgenic flies where you can actually visualize. You know, the nuclei at the same time is visualizing the state of the nuclei. In this case they're undergoing division. And what you see here and cell cycle 13. Just to give you an idea of how fascinating and dynamic this process is. They actually, they actually the nuclear division happens in waves. So it starts at this pool. And then you can see over time. And by time I'm talking like seconds. Okay. like this entire process you have here? I don't know. Do I say, see time? Yeah, like, look, these are hours and minutes, like 2 and a half hours, 3Â h. This whole process unfolds. That's also why just offler model organisms. The entire phase of embryogenesis is like 17Â h. It's less than a day depending on the temperature. It's raised then. And so you can do really cool experiments very fast. Again, we'll get that more detail when we talk about flies more generally in a later lecture. But so you see that, like the cells don't all divide, you know. Sorry the nuclei don't all divide, you know, ubiquitously, at the same rate, same time across the embryo. It's actually these beautiful mechanisms in place to orchestrate how these processes unfold in this case it's actually happening as a wave from the left side of this image all the way to the right side, the nuclei will undergo my to, they'll actually undergo division. Yes, question. Unknown Speaker 00:44:48 Andreo. Rajendhran Rajakumar 00:44:49 it's it's still like outside, inside. Kind of relative again, you know, it's like they're on the most most outermost periphery. Let's just say that. yeah, let's yeah, yeah, they are. They are. I mean, it's a little bit more obvious. Well, yes and no. I mean again, like solarization happens here, and you it's hard to see. But you can kind of see the difference here versus here. They're a little bit more protected, a little bit more encapsulated in the cellular. Of course, now they're cells and not just nuclei, right? Everything here and earlier, we're talking about nuclei, not cells while here and beyond. We're talking about cells. So yes, at this stage they're a little bit more vulnerable, presumably, but they're still part of the embryo. They're still very much part of this. Yeah, the periphery. Any other questions? Yes. Unknown Speaker 00:45:43 so the so it's not the germ list. So there's so just to make sure distinction of terms. So when I say Rajendhran Rajakumar 00:45:49 germ layers, that's like, you know, the ectoderm endoderm mesoderm germ cells, though I know it's some words are fully loaded germ cells. Those are the things that become sperm or egg. And so the pull cells, they contribute to the things that are the sperm and the egg, the germ cells that makes sense, no somatic, everything else of the body of the animal. Yes. after cellularization Unknown Speaker 00:46:19 on the middle. Rajendhran Rajakumar 00:46:22 you know I'm going to be honest with you. I'm not totally sure I'm not sure. Yeah, I'm there's one or 2 of possibilities. They either don't do much except for maybe some signaling regulation purposes or they actually do one. Because, remember, like. there's going to be a lot of cool movements you're about to see in a video. I'm about to show you they might also start to. Now, now, it's not just gonna be like things looking like it's exclusively for free right? They might just start integrating it you into specific part of the embryo. But I don't know for sure. Yes. Good question. One more time. Yeah. I might have some slides coming up about that? If not, then I'll revisit that question. Okay. okay, so just a very quick visual of all these are the notes I want. I was looking for in the previous one. So so just kind of a cartoon rendition of like viewing the nuclei that you see over here. So they're separated from each other. These nuclei by these filament, these filamentous-looking, like things. They're basically cytoskeletal elements. that are basically creating these nuclei as islands. They're isolated from each other. Okay, and so that's it's it's but it's not a cell. It's not a cell membrane. It's just nuclei. any other things I wanted to mention about that. Yeah. So again, you know, this, this was the part I wanted to read to you. You know, it's nuclear undergo S phase or DNA replication. Sister chromatins get pulled apart, reassemble a nuclei so kind of like how you would think about stellular division. It's just this nuclear division that's specifically happening here. So again, cytokinesis doesn't happen. What? The heck? Right? It's totally totally weird. And again, like what you see, especially in this cartoon, is very easy to and imagine nuclear dynamically ordered within this decision of the early embroidery positions, using these set of skilled elements that you see kind of highlighted in green here to keep them kind of associative each other, but still separate. So this is kind of like at the stage later and then beyond. So now, this is showing the cellular move cellular movement, not nuclei anymore. This is from the very beginning of this, video cells are moving and tissues. They're starting. They're specifying, differentiating. Tissues are getting specified now. There's more going on in this video than just time. Okay, the embryo. you know, say, like anterior to the left and posterior to the right for simplicity. It's actually rotating. Okay? So you can kind of see, like along the whole circumference of the sphere. Okay. we're not just looking at like this one side of the sphere and seeing what's going down. It's actually rotating on an axis like in like 3 60 rotation through the video. So you're like what? That's a lot of movement going on, I mean, yes, don't get me wrong. There's a ton of crazy cellular movement that's going on in the embryo. But there's a lot more action going on the video than it might first look, there's actually this rotations. You can kind of see, not just the anterior posterior, but also what's happening in the dorsal ventral differences of the embryo, the backside, and the and the belly side of it. So just to show you the video again, give you an idea. So the beginning. See at the beginning how you can kind of see the cells. So this stage being kind of like what you see. what's pretty, what's pretty much at this stage over here. So solarization has happened has happened. And then what happens after this? What happens after this 3Â h? Mark what happens to the rest of the 14Â h of embryogenesis of drosophila. That's this movie. So again, now imagine it's rotating. Also the embryo for you to visualize. So you see, here's this one side. We'll talk about that in a bit. It's rotating, rotating, rotating. Here's 180 degree angle of that same set I just highlighted. That's pretty well. This closes. We'll go over these stages, you'll you'll see in much more detail, step by step, in coming lectures. Okay. So now, telling a little bit of more about syncytial specification. So remember, I was talking. I gave a little clue about molecules being distributed within the cytoplasm of that whole embryo and they're distributed way before sellerization happens right. Well, how does that look like? And also I mentioned the word morphigen. I mentioned the word gradient or the I. Actually, I don't think I did say morphigene, but I did say gradient. So these molecules can form gradients of themselves across some direction of the embryo. So here's some examples which we'll get into way more detail as the class goes on. But just to give you kind of a visual. So this is molecule called Bitcoin. You can see at the anterior of the embryo anterior's left posterior is right. Ok. Head left, but right in green is highlighting Bitcoin protein expression. So Bitcoin is expressed highest at the most anterior most part. and it starts to die off as you go away from that interior region. Okay, and this is protein. And this is protein that actually is coming from that's deposited by the mother into the egg. This Bitcoin protein. It wasn't. It wasn't a gene, a bitcoin gene that was transcribed from one of their this organisms nuclei and then translated in what's one and work? It's even before cells, membranes are there. They're not even cells. Yet this Bitcoin protein is distributed in a pattern like you see here we were to graph it, y axis being Bitcoin intensity, X-axis being, are you more anterior more posterior. The distribution of the protein, you can see, is really high at the anterior, and then and then it peters it like sigmoids off into nothingness as you go more posterior. So you see, then, that's what we would call a gradient. So there's a gradient of bitcoid in the cytoplasm of the entire embryo. It's high up here, and then it goes down. and then huddle is a posterior ma, a protein that's in that was deposited by the mother in the, and it localizes in the posterior, it's highest, most than the posterior, and goes down towards the anterior. So you have these 2 opposing gradients of these molecules. And if you're nuclei right in the embryo and you have cellularization that's happening here. So you're free, floating nuclei. No, no, sorry, not free, floating, floating nuclei. And they're not free, floating in the sense that they have cytoscalital elements that are still keeping them in their respective positions. Right? So you have nuclei that are, you know, distributed along the anterior to posterior axis. This is a simplification of these columns, but let's just go with it. So if you're over here. You have a ton of bitcoin present near you, and a little bit of of caudal the posterior mark. If you're way over here, you have, like no Bitcoin protein, lots of caudal protein that these nuclei are exposed to, and when cellularization happens those cells take in different amounts of bitcoin or caudal protein depending on where they were. You know, when the solarization happened in respect to where those proteins were localized were distributed within the entire embryo. and that creates this this ratio across anterior posterior gradient. There's a ratio of how much Bitcoin versus caudal protein translate that. How much head you know anterior protein to how much posterior protein each cell has, and depending on how much anterior protein you have versus posterior, you'll be a more anterior type structure that you'll give rise to. If you have more posterior protein versus anterior, you'll become a more posterior structure. so they pour, for they help contribute to this anterior pursuit specification. Eventually we'll give to major regions of the embryo soon after cellularization happens, which is cells that will give rise to the head, the thorax, the abdomen, and the more posterior part of the abdomen of the adult fly. Okay. and and just. A little preview of of things to come come very soon. These molecules that have the ability to dramatically influence morphogenesis. As these key events are occurring like making something more head-like versus more. But like they're called morphogens. So they're not just proteins. They are a special class of proteins called morphogens, because they form gradients like you see here, these are actually called morphogen gradients. But we'll get into that more detail later. So like, just to think about an experiment here. If you had a normal embryo. okay, normal developing fly embryo. It has Bitcoin messenger, Rna, that that you know Bitcoin protein in the most anterior part. Of the embryo. So at this stage it's it's bit, Mrna that was deposited by the mother. A cellularization happens. It begins to be translated into protein. So over here, Bitcoin protein, there's nothing anywhere else, or Rna, there's nothing anywhere else. You have the stage where now you, the embryo, knows where it's anteriormost. Part of its head will become the rest of the head region, thorax, the abdomen, and the most posterior part of the abdomen. If you were to look at a mutant embryo, that is a mutation of bitcoin. What happens? Well, again, Bitcoin is important for form in the head. You remove Bitcoin. They don't make a head. They just make posterior identity structures. So they have. you know, an abdomen and 2 bots, basically, you know, coming up well, I mean the abdomen's kind of their whatever. You have an abdomen and 2 most posterior parts of the abdomen on either side of this. So all of these more interior, like structures. from a little bit to more to the most anterior-like structures. They're gone because they don't have the key morphogen to help instruct and specify them what to become in those particular regions of the embryo. and we'll get into more expansions of the experiment. There's more, there's more cool parallel experiments that have done to this, but we'll get to that in a later lecture, just to give you a preview of now a little preview of the genetics of development. And how you can study developmental genetics. It is a thing. Okay, Toronto landscape is selfish maturation. So now let's zip in time. I just showed you an experiment that came from like the 1980 S. And I'm going to show you experiments that came from the 2,010 s. Like 2,017. It was published, or 18, probably 17. So now, zebrafish embryo. you're like, wow! Those look funky. We will explain. Go through the whole process of how these funky things become the way that they become. We'll have a whole part of electron zebrafish development later in the class. But just going with it with me. Here you have these different thing entities here is trying just basically a cartoon rendition trying to represent. These are different developmental stages of zebrafish embryos. So you can take, take an organism at different developmental stages. You can actually diso for each one of them. Okay, you can dissociate their cells into single cells. put them through a microfluidics device. I'm not gonna get into the complications of the microfluidics device now. But basically, the way it works is individual cells are basically you through pressure are pushed through this microfluidics device, and as they're going through they're then insert, they're immersed into a bilipids or will or oil. However, you wanna view it or think about it. And basically in each oil droplet in each one of these lipid droplets is a single cell. So they're basically isolated as single cells, and then in parallel to that, when they're encasing these lipid droplets in this microfluidics device, they're also in case there's other factors to allow for barcoding all of the transcripts into in that particular, that that cell, particular cells being expressing at that given point in time in development. and the reagents to sequence all the transcripts in that single cell. So this is a method that allows you to do single cell transcriptomics. So each one of these cells of, say, this embryo, you separate them, and you can do the entire transcriptome of each one of the cells of that embryo, and you can do with this stage? You can do it, that stage. And each one of these stages that time point those time points. Can you can separate them in data analysis by giving them different colored barcodes or different sequence barcodes. And so you can actually visualize You know, these cells of this embryo that give rise to the head region of the zebrafish versus these cells. Down here they give rise to the tail. What is their what is the activity of each one of those cells genome at that point in time and development. What are the all the transcripts that are being made? And you can do that for all the cells in some bureau. and and this one and that one so across the entire process development to get an idea about the identity in high ohmic level precision of each of these cells at each of these time points. And that's a whole new world of gerontobiology that we are now at with the this revolution in genomics of single cell and and and transcriptomics, single-cell transcriptomics. So just to give you an idea of like how this, how this works. you're not going to be tested on this. I'm just trying to give you an idea of like where we're at now, the crazy stuff that people are doing to imagine. Once upon a time in the early 80 s. We were trying to figure out this one Bitcoin factor. What does it do when you mutate it in in an embryo. And where is it now? We're looking at all of the transcripts, all of the genes in the entire genome of an animal. Where are they expressed in the embryo at any given point in time so completely different? You know, Giant, jump, leap in technology that we're at now. So, to give you an idea, these are cells of zebrafish. So 4Â h post fertilization. these are cells that were isolated from many, many embryos at exactly that same stage. And at this stage most of the cells don't really have any kind of specification yet. But there are 2 populations of cells that will give rise specifically to the endododerm Unknown Speaker 01:00:47 and epidermal cells. Rajendhran Rajakumar 01:00:49 If I'm looking at the legend property. as you see, as you go to 6Â h, post fertilization 8Â h, 10Â h, 14Â h, 18Â h, 24Â h. So this is kind of like a 24Â h. First day post fertilization time window. You can see not only the structuring of these cells, how they're structured with each other in terms of differences of gene expression they have amongst each other right like, if this isn't just like, you know, this cell has a little more of gene A than Gene B. And this cell has a little more of gene B than Gene a. And this third cell has none of the no gene, A or gene B express. That's just one gene cells talking about thousands of cells. We're talking about all the genes, all the transcripts that are possible. Right? So that's how these graphs are being constructed. I'm not gonna get into the statistical methods of making teeny plots. But just going with me here. What you're tracking color is probably the best way to what to absorb from this example, you can notice how more colors are appearing after these few hours are passing between each of these panels. What's happening here is that these cells that were undifferentiated, start taking on certain cell fates, and they start to take being specified and taking on particular identities that now start to include things like neural cells. or the germ, you know, mesoderm cells. endoderm cells, epidermal cells, so on and so forth. And if you were to try to re again, think about not just that was that was you know, across that one date period. But if you were, look at all of embryonic development. and each one of these colors. So the colors in this image here they represent different cells coming from a different time point in development. Right? So blue would be the most coming from embryos that are the most developed. Gray embryos cells are coming from embryos that are the least development. You know, yellow and red kind of intermediate. For example. now, if you were going to reconstruct the similarities and differences of all of the gene expression of cells coming from those various things. you. It ends up generating this tree looking thing where basically, you have cells that are, you know, gray, because, you know, they're represented for reflecting cells coming from this early embryo. And you, basically, they begin to kind of differentiate into more and more specialized cell types. And you can see that actually, those more differentiated cell types are more enriched in time from more later developing embryo, because it's in that later stage of development. When those cells become terminally differentiated, they take their very, very specialized cell type identity, and you can see them at the tips of the branches of the all of this tree. so to speak. So these developmental trees is kind of a new thought, a new motif that people are starting to talk about now with the advent of single cell transcriptomics. So this was the breakthrough of the year in 2,018, in all sciences. And you know, 5, 5 and a half. Whatever years have passed since then, and you can only imagine what people are now doing with technologies like this. Pretty incredible. just to kind of push forward a little bit the information that these trees are that you can analyze these trees. So this is the tree you just saw. But now we're just looking at 3 dimensions. To. To be honest, it's it's actually a dimensionless thing for the most part, because it's representing many, many, many dimensions of gene expression differences between things. But you can see here different regions that give rise to different parts of the embryo like, so that this these cells contribute to the telencephalon. These cells to the epidermis, these cells highlighted here, the ender derm, for example. an auto cord precursor. Now gene cascades. So each one of these trees that are flushing really quick, these heat maps. They're the expression of each gene of the genome of the animal. Across all these cells you'll see some cells that are gray. They don't express that gene, some cells that are super red, they express a ton of that gene and some that are yellow. They express an intermediate around the gene. So this is, we're talking about tons of data here. we're talking about of all these cells across all these demental stages which cells are expressing specific which genes. So you can look at each gene one by one. How are they expressed? What's the pattern across all these cells across all these developmental times. And then you can look at cells that representing clusters through using markers. Is it going to give rise to say, the nervous system cells that represent the nervous system or muscle cells. And just say, for example, those muscle cells you look at the cluster that are supposed to be only muscle cells, or all the genes that are expressed in those muscle cells across time. Younger muscle cells, intermediate age muscle cells fully maturely specified, terminally differentiated muscle cells. So just tons and tons of information, as if we didn't already have enough information with the advent of genomics in the 2,000 s. Now we have single cell Omics, which is just it's almost too much to be honest with you. Okay. but that's that's just how we do. Okay. so I'm gonna go over a couple of a couple of things that I cover in much greater detail in molecular biology. But our key things to understand for this class, so so might be a review from any of you. Some things might even look very familiar, probably because I took it from this class to help inform the molecular biology class, because some of these slides were just really good. But just an overview. Okay? So you've got at the top here a nucleus with your genome, the cell's genome at the bottom. Here you have this fully processed, mature protein. It's in terms of its structure and any kind of chemical modifications that has been done to it. And it's been localized. In this case. It's it's a it's a membrane protein that's been localized at the membrane of the cell. And it's doing some cool membrane function, whatever. Right? Well, there's a whole lot of steps that go in between that process that includes, you know. Of course. you can replicate. You know your and your your nuclear information and contributing to cell division. Let's say we're just looking at a single cell. So you have DNA. There's transcription that happens. You have messenger. Pre-messenger running. That is processed splicing. Yeah. Alternative isoforms. All that kind of stuff. you know. Have a mature messenger, Rna. It's transported out of the nucleus into the cytoplasm. Barbosomes are waiting there to translate those mature transcripts and by trends through translation, they become. They're translated into proteins and those proteins. various different other molecule, chaperones and complexes take on and also sub self self emergent properties form 3 dimensional structures, and then are potentially further modified by other small little chemical modifications on the protein that could influence their function. So that's that's what had what's happened that's going on from DNA to Rna to protein. and many of these stages can be regulated can be influenced to dictate which genes are being activated or repressed. What transcripts are being made from those active genes where those transcripts going? Are they going to the anterior of the embryo like the fly. I just showed you Bitcoin, Mrna. or are they distributed everywhere which cells are transcribing more of more gene x versus gene y proteins are okay. Proteins might be in all the cells of the embryo at a certain stage, but in some of those cells that particular protein has been chemically modified. You know a methyl group has been added to it, or something like that, any simple kind of organic chem modifications, right? And all of a sudden, now it's active in those sub. So cells, while it's still inactive. In all the other cells. there's many different regulatory processes that can happen going from DNA to protein, and all of those are are checkpoints. They're not just checkpoints. They're all opportunities for cells to tinker with. that can eventually influence what their identity will become and where they will go and what they're gonna contribute in terms of the formation of tissues, organs, and the body plan of the animal. So again, this figure. You know, I start the whole microbiology class. And I ended with this figure, you know, again, like cells replicating this replication. But of course, transcription Rna processing translation, protein formation. Remember all the cells that we're looking at in these embryos as we're watching embryogenesis unfold in different organisms. These are processes that are happening at differential rates in different cells, and that can influence dramatically the process of development. So again. you know, the molecular biology of development is essentially kind of what I'm hinting hinting at here. And why that's important is because, again, like each of these cells have a nucleus. And you know, that's the entire genome of the animal. And you know, genomes. They have in them the potential to give rise to many different things. But it's how it's act. Different parts of the genome in that of that nucleus are activated or inactivated. And it's through these different combinations that you have cells behaving and becoming the way that they do right? Okay. But just to put again a little bit forward further to an extreme of the potential of things. 2 things. One think about the sea urchin. We just saw the sea urchin cells. the they have the known nuclei, and somehow beautifully, when they're isolated through because of conditional specification, they can orchestrate their genomes in such a way and through the division events. such that they can recapitulate the entire animal right? Another beautiful example of genome potential to think about during the developmental process is or is the animal cloning the, you know, incredible milestone in? I don't know. Well, let's say deval topology. At the very least, I don't. I guess that's the discipline that would take ownership of this incredible work. So, Dolly, the Sheep. maybe you guys that name might not ring a bell to your generation anymore. But back in the day this was the first, you know, cloned animal. How did this happen? Well, if you took outer cells or memory cells from a nuclear donor and you took an egg from an osoid donor, you removed the nucleus out of the egg and so you enucleated it. And you took the nucleus from these other cells, these memory cells, and you transplanted it into this nucleus lists like lack of nucleus egg. You zap! It don't ask me what exactly is happening with these lightning bolts. but it's it's I think it's actually a lot simpler than it looks. It's really just well, you'll learn in the fertilization lecture a little bit more clues about how important bioelectricity is to this very important activation of the embryo Genesis project process. But how they did it artificially. Let's just call them lightning bolts. Okay? So when you activate embryogenesis and one cell becomes 2 cells and eventually give rise to a full-blown. You put that into now a surrogate and it gives rise to Dolly. So this happened. okay, when did it happen? I don't know. Like 2,000 something. maybe late 90 s. Or early 2,000 summer around there before you guys report and you know what Dolly actually gave birth to. It's fully capable of giving birth to its own progeny. In particular it was gave birth to. I think his name was bonnie cute super cute. Okay? But the point is that the nucleus of the actual nucleus that was donated? It came from Unknown Speaker 01:12:25 a memory cells. Could it come from other cells Rajendhran Rajakumar 01:12:27 of the nuclear donor. And so it's just nuclei that now, once it's given this egg context and it's apt to initiate embryogenesis. Those nuclei again, think about conditional specification. Think about the context. Think about how now. The genome that was supposed to just make mammary cells now is a genome being used to eventually contribute, through all of its progeny of cell, the of nuclei that are in the progeny cells after cellular division. The entire animal. That is this sheep. Okay. So cloning is a great example to really show you and bring home the power of the genome, so to speak. Okay, gene expression talk to. We're talking a little bit about that. In some of the previous slides there's many different techniques to look at gene expression. We'll get into them in great detail soon. But just to give you an idea that genes are of cells of thousands and thousands of cells in the embryo, they're not all, not all expressed at the same levels. At the same time. they can be expressed at different levels at the same time. They can be expressed in some cells at one time, then turned off at a later time. But at later time the other cells are expressing the gene. So there's a lot of space and time dynamics when it comes to the activity of genes in cells, and that activity that that activity of genes can dramatically influence what those cells become and the structuring and formation of tissues. So again, like the developmental genetics which you can do, a whole class just called gene activity or development or dental genetics. I've taken them as grad as a grad course. You probably give multiple grad courses of it. Now, considering how much advancements have happened since they took in like 2,007 or something. But just again, to give you an idea, little preview of what we're going to get in the class. Here's this gene oddskipped. Why does it call that? We'll talk about it later. You can kind of see it at the stage 5 embryo of a fruit fly. At this stage is hundreds of thousands of cells anterior, the left posterior at the right. Usually that's always the case when I'm showing flying wheels. So you have these stripes of expression where it's really highly expressed along the cells along each of these stripes. And in between these stripes. It's kind of lower expression levels. Then, at this later stage. these domains, these stripes become even more resolved, more distinct. Where you have again, like A region of the embryo, where the gene on script is highly expressed of cells in this that are within this region that is represented by the stripe and then cells in between those regions. 0 expression of of that gene on skip, this very important transcription factor for setting up segment identity. And the way that we're visualizing. And then, of course. odd skipped in flies is a highly conserved gene for figuring out the anterior posterior segmentation process of an animal of the embryo's body plan. We have odd skipped also in our genome. It's just called odd, Skip, related one. You're gonna see a lot of things like that throughout the class. We discover the genes first and flies, and then we realize, oh, wow! Humans have them, too. We'll just kind of name them after the fly genome are really the cornerstone for genetics, don'togenics, don'tology, and many other disciplines in biology. You will see that theme recurring through a class. So in in developing, there's probably a mouse embryo doing probably should say. is, yeah, it's most embryo. So you see, in its kidney heart. In this more anterior structures is actually odd skips and very important for the formation of the palate of the embryo of you know, the craniot. Think about cranial facial development. It's also important for the developing heart and developing kidney kind of see? Really bright here and right here. So very important for whether it's mammalian development insec development. Very important transcription factor that functions during development to help set up the formation of certain structures or organs along the anterior to posterior gradient and the colors that you see here. For those of you who aren't familiar with this technique is a technique called in situ hybridization, where you're able to visualize transcripts of a certain gene, M. Messenger, Rna. Of a certain gene which represents that gene has been activated in those particular cells. We will go through the process of institutization later. But just again, to give you an idea, you're seeing at these particular stages, which cells are by visualizing either with color reaction that you see of just, you know, regular light, or you can also use fluorescence, mean methods as well. So imagine they see a fluorescent images of these stripes just ways to visualize in space and time which cells are expressing in gene or not. Okay? And I'm good. I'm going to be skipping through some different aspects of molecular biology. Just again, to give you guys some keywords, some lingo that's going to be relevant for the rest of the class. Okay? So talked about active part of the genome, inactive part of the genome. How? How is our new nuclear information actually organized within our our nucleus? How's our genome organized. And the way that it is organized is it's you have our our DNA that's actually wrapped around these. These octomurs, these structures that are called awesome octoma specific, more specifically called histone octomurs. So the set of 8 histone proteins. So they're in pairs. It's actually 4 histone proteins and couple and couplets. They form kind of this little well, yeah, again, an octoma is probably the best way to call it is octa, because there's 8 subunits and DNA represented in gray in this picture here. It's kind of wrapped around each of these octomurs. You have some DNA that's kind of linking bridges between each one of them. And now how open and separate each of these octumers are, with different regions of your genome wrapped around each one of them depends on whether you have u chromatin, or open. accessible, active regions of chromatin which you see here, where you have dense package, chromatin, all aka heterochromatin that are inactive. Inactive regions of chromatin. That are inaccessible for transcription factors like oddscript you saw in the previous slide that can activate genes in the part of the genome that are in these regions here so active regions, inactive regions. Each one of these histones have what are called a histone tail can I see like floating off of them. It's just amino acids that are part of the protein that are just a little bit more accessible in the exterior part of this octum. and this is still until can be modified, which I might explain in couple of slides from here. you have histone, 2 b, 2, a 4 and 3, and there's doubles of each one of them the other. Their other versions are just below here. and histone one in case anyone's ever curious. It helps form kind of bridges. It helps. It helps keep them together, basically in compact forms. More typically. but really h, 2, a, 2 B and 3 and 4, and the most important, and these histone proteins are in everything from us to yeast. These are extremely important, highly anciently conserved proteins that organize DNA to to influence their activity. okay, maybe we'll stop on this slide for today. So steps in the production of beta globin and hemoglobin we'll talk about in next class. But it's basically trying to break down for you again, just some basics of transcription and translation and protein modifications. And then we can start after a few slides, get more and more into the really cool stuff of development again. Alright!