[LEC] BC 180 Notes.docx
Document Details
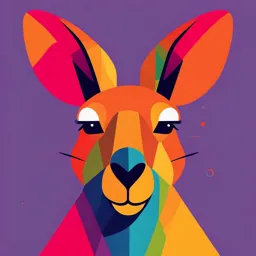
Uploaded by DazzlingFreedom
UP College of Medicine
Full Transcript
1.1. Biotechnology vs other fields Biotechnology: application of sciences to create different products Biochemical engineering: chemical engineering in biological sciences Pharmaceutical sciences: drug development (without the use of living organisms) 1.2. History of Biotechnology Ancient (pre-18...
1.1. Biotechnology vs other fields Biotechnology: application of sciences to create different products Biochemical engineering: chemical engineering in biological sciences Pharmaceutical sciences: drug development (without the use of living organisms) 1.2. History of Biotechnology Ancient (pre-1800): cheese, beer Classical (1800–1945): vaccine, crossbreeding, penicillin Modern (1945–present): manipulation of genetic material 1.3. Fields of Biotechnology Microbial: manipulation of microorganisms e.g. cloning into a vector Agricultural/aquatic: biotechnology for plants / animals Bt-modified organisms: pesticidal spores Genetically altered cats and zebrafish Bioremediation: contribute to reduction of environmental pollution Genomics: application of bioinformatics to analyze genomes Medical: whole spectrum of human medicine Pharmacogenomics: influence of genetic variation with drug response e.g. precision medicine or targeted drug therapy Regenerative medicine: treatment via gene therapy Regulatory: quality assurance and control A2: Classic Techniques of Molecular Biology and Recombinant DNA Technology 2.1. Classical paradigm and tools of molecular biology Paradigm Genetics Biochemistry Molecular biology Tools Restriction enzymes Essential in cutting foreign DNA Cuts unmethylated DNA sequences that are palindromic in character along an approximately 6 bp recognition site Types Type I: ATP-dependent; random (>1kbp from recognition sequence) Type II: non-ATP-dependent; ~25 bp from recognition sequence Type III: ATP-dependent; within recognition sequence Agarose gel electrophoresis Essential for visualization of differently sized DNA fragments Restriction fragment length polymorphism (RFLP): differences among people in their DNA sequences at sites recognized by restriction enzymes (application: DNA barcoding e.g., species identification) Normal cell: two bands along the MstII site Sickle cell anemia (characterized by a missense mutation): one band along the MstII site Recombinant DNA/Molecular clones DNA fragment: gene of interest Vector: where target gene is inserted: can self-replicate and have selectable markers Kinds Plasmids: 50bp to 20kb Cosmids: up to 45kb BACs (bacteria): 100 to 300 kb (not necessarily used for molecular cloning; if used, confirmed via blue-white screening) YACs (yeast): up to 1 Mb (more applied in organismal sequencing) Essential elements Origin of replication Antibiotic resistance gene Multiple cloning site Promoter Polylinker: inserted DNA fragments with multiple recognition sites; can be synthetically inserted via PCR Expression cloning Transformation: heat-shock, electroporation, etc. Transfection: calcium phosphate, liposome, etc. PCR DNA sequencing Blotting 2.2. Recombinant Protein Technology Natural (expensive, can have negative effects) vs recombinant (cheaper, safer, abundant) Vector selection Compatible with host system: prokaryotic vs eukaryotic Contains elements such as: Inducible (on/off switch e.g. lac operon) vs constitutive promoters Ribosome binding sites Tags Expression vectors for E. coli pBAD: inducible by arabinose Lac operon system: inducible by IPTG pET: produce recombinant protein up to 50% of total cell protein, host bacteria typically strain BL(DE3) with T7 promoter pGEX: encodes GST tag gene, useful for affinity chromatography Protein purification and detection Basic strategies: addition of tags, etc. Column affinity chromatography A3: Metabolic Engineering 3.1. Brief Background San Francisco: biotechnology capital of the world Genentech: leading pharmaceutical in San Francisco, California; pioneer of biotechnology in 1976 Academic/government institution Pharmaceutical companies 3.2. Metabolic Engineering Cells can serve as sustainable “factories” for beneficial biomaterials Ex: insulin derived from pancreas glands of pigs (contaminated by other proteins) vs. recombinant insulin (highly defined and high purity) Purposeful modification for enhanced production of a desired material Produce novel material Lessen cellular expenditure Decrease byproducts Degrade undesirable material Strategies and procedures Pathway design Systems biology Synthetic biology Strain Optimization Scaled up fermentation and purification 3.3. Pathway Design Even before designing pathways, identification of (renewable) source of raw bio-materials is done Identifying a biotransformation pathway Retrosynthesis: process of “deconstructing” target molecule into available starting materials Chemical reactions/processes Enzymatic processes Computational predictions can now be used to design the pathway 3.4. Systems Biology Challenges with proceeding with designed pathway Not all organisms have all the genes needed in the pathway Not all are suitable production strains Not all genes can be expressed heterologously Interrogation of complex biological systems through large-scale quantification of numerous biomolecules DNA–mRNA–protein–metabolites Possible scenarios: Overproducer; large-scale (ideal) Incompatible to lab conditions Imbalanced flux in pathway Accumulation of product is toxic Incomplete pathway in one 3.5. Synthetic Biology (Strain Optimization) Use of “workhorse” strains Perform genetic manipulation Knock-in/out genes Modifying promoters or adding repressors Taking a plasmid with high copy number Flux optimization Culture optimization pH (buffer) Temperature Aeration Osmotic pressure (salt) Carbon source Feed material concentration 3.6. Adaptive Laboratory Evolution Multiple cycles are necessary to screen synthetic strains = very low efficiency of evolution “Brute force” approach for screening greater number of strain variants Process: Unoptimized candidate strain is passaged until better-performing “evolved” strains are obtained Apply positive/negative pressure Positive: feeds the strain Negative: kills the strain Fittest strain is isolated Genome sequencing Reverse engineering Metabolic rewiring Note however that there is not one solution to a given biotransformation Exciting areas for metabolic engineering Bioremediation Drug delivery Protein engineering 3.7. Protein Engineering Purposeful modification of peptides and proteins for enhancement of existing or endowment of novel features Physicochemical properties Structure Function Structural Biology: structure relate to function; where protein engineering is anchored Ex: Akt kinases PH domain: allows its recruitment to the plasma membrane (via phospha- tidylinositols) Kinase domain: catalyzes protein phosphorylation via ATP/substrate binding Structure-based engineering does not always work as planned Tools Sequence optimization Non-natural aa incorporation Directed evolution Bioconjugations 3.8. Sequence Optimization Physicochemical / structural properties Solubility: increase hydrophilicity by adding charged amino acids Proteolytic degradation: prevent trypsin degradation by lysine and arginine mutations Redox sensitivity: remove cysteine residues Case study: Insulin therapies Types: Active, short-lived monomer Inactive, long-lived hexamer Insulin lispro Inversion into Lys-Pro in the B-chain C-terminus results into its clashing with the Arg of the other B-chain and opens up the surface Prevents the dimerization of insulin, locking it into the monomeric form, making it fast-acting Insulin glargine Introduction of two Arg in the B-chain C-terminus Increases pI from 5.4 to 6.7, precipitating it out at the physiological pH and making it less soluble and long-lived in bloodstream A-chain C-terminus Asn is degraded via deamination hence the need for Asn → Gly substitution 3.9. Directed Evolution Multiple rounds of random mutagenesis to allow screening a large mutational landscape and accumulate desired beneficial mutations However requires a functional screen i.e., an activity-based assay for peptides/proteins which mostly only attach to other proteins without activity Hence affinity based evolution platforms were developed where genetic material is connected to the protein Phage display Yeast display mRNA display This allows screening of protein-protein binding interactions and enriching mutational variants Essential for antibody drug research Frances H. Arnold: directed evolution of enzymes George P. Smith / Sir Gregory P. Winter = phage display of peptides and antibodies Case study: Peginesatide Small peptides were found to mimic erythropoietin (EPO) in its ability to control hematopoiesis signaling EPO = 34 kDa Peptides = 2 kDa (<15 aa) Some amino acids are conserved which are essential for binding to the EPO receptor More engineering strategies are done to produce the final drug Charged terminals are modified as it may inhibit receptor binding & signal protease degradation Dimerization increase concentration of peptide into the receptor Unnatural amino acid incorporation e.g. PEG stick to blood proteins and enhance receptor binding 3.10. Non-natural Amino Acids Amino acid can also be chemically synthesized, in addition to ribosomal synthesis This allows more access to more functionalities and expansion of features Case study: GLP-1 analogs Peptide that acts similar to insulin, increasing glucose utilization by binding to GLP-1 receptor It is short-lived; cleaved by a protease DPP4 To optimize GLP-1, a sugar was introduced to an amino acid to stabilize it against DPP4 cleavage Benchmark GLP-1 analogs: liraglutide and semaglutide 3.11. Bioconjugations Chemical or enzymatic modification of existing chemical moieties within a peptide/protein Amino acid bioconjugation e.g. Lys → N-hydroxysuccinimide (NHS) esters or Cys → Maleimide Peptide modification: primarily use tags to introduce Downside of tag-and-modify Introduction of large, artificial sequences Stochastic/random modification without precision Hence, click reactions are developed Click tags are very small, generally smaller than peptide motifs; produces high yield and selectivity products by carbon-hetero bond formation reactions Carolyn R. Bertozzi / Morten Meldal / K. Barry Sharpless = development of click chemistry and bioorthogonal chemistry Case study: Antibody-drug conjugates (ADCs) Chemotherapy (efficient killing of cancer cells but also kills normal cells) vs immunotherapy (highly specific to cancers cells but inefficient killing) ADCs have been developed to link cytotoxic drug to an antibody, resulting to high specificity, efficient killing of cancer cells 3.12. Synthesis / Summary Biochemistry vs chemical biology Biochemistry: study biomolecules in their natural biological context Chemical biology: study of biomolecules by introducing chemical alterations to create more biology 3.13. Biology as a Basis for Biochemical Engineering Since DNA sequence completely defines an RNA, which subsequently completely defines a protein, then any desired protein can be manufactured by cellular machinery Via large-scale fermentation given gene is inserted to host Cohen and Boyer: use of restriction enzymes for recombinant DNA technology While genetic engineering leverages the catalytic machinery of the cell to manufacture proteins, metabolic engineering goes one step further, enabling the cell to manufacture enzymes to tune the cell's own catalytic machinery. Furthermore, metabolic engineering empowers the cell to carry out a wider variety of chemical reactions. Whereas genetic engineering enables the cell to make proteins, metabolic engineering enables the cell to make vitamins (5), antibiotics, chemotherapeutics (6), other small-molecule drugs, industrial polymers, dyes, fuels (7), and other specialty chemicals. All that is required is a host cell with the necessary starting materials and the necessary DNA genetic material coding for the pathway enzymes. Complexities of cellular manufacturing Metabolic flux: rate of turnover of molecules through a metabolic pathway; metabolically engineered pathways suffer flux imbalances; solved by modulating expression of enzymes, directed evolution, control of spatial organization Metabolic burden: increased levels of non-essential proteins robs the cell of its building blocks Genetic instability: genetically engineered are less stable than plasmid-free non producer cells; caused by loss of and mutations in plasmid DNA vectors Future of biochemical engineering Genetic engineering: production individual proteins Metabolic engineering: introduction of individual metabolic pathways in cells Future: engineering entire new cells = synthetic life In 2010, J. Craig Venter created the first cell with a synthetic genome Complete genetic system is reproduced by chemical synthesis, starting with digitized DNA sequence in a computer A4: Protein Analysis, Expression, and Purification 4.1. Protein Analysis and Purification Analysis of protein extract Protein quantitation (A280) DHFR enzyme assay Good purification scheme = good purification level & yield High degree of purification but poor yield leave little protein for experiments High yield with low purification leaves many contaminants in the fraction 4.2. Expression Systems Bacterial systems Grow quickly but has difficulty expressing large proteins Alternatives: Yeast (Pichia pastoris e.g,, used to infect sex cells) Virus (Baculovirus)–insect cell system Mammalian cell (optimal) Yeast cells Single-celled eukaryotes, but similar to bacteria in reproduction Strains: S. cerevisiae Pichia pastoris Cloning scheme: double crossover recombination event occurs between gene of interest and linearized plasmid containing viral recombination region Grows quickly and allows expression of large proteins Baculovirus Insect viruses with dsDNA genomes (insect larval host) Plasmid into baculovirus DNA and undergoes lysogenic cycle Used for difficult-to-express protein with low level of risk Can express large proteins but grows very slowly Mammalian cell line Optimal: all necessary processes can be achieved correctly Eukaryotic vectors: human adenoviruses and retroviruses, SV40 Selectable marker gene: DHFR Cells: derived from CHO cell line Transfection Transient: for plasmid introduction (e.g. electroporation); foreign gene need not be integrated into the genome Stable: for protein expression Selection Methotrexate (MTX) selection DHFR gene makes cells resistant to MTX Gene is expressed in increasing MTX levels Generally used to test the function of a protein in vivo rather than produce proteins in large amounts Best expression systems for: Large proteins (>100kD): eukaryote Small proteins (<30 kD): prokaryote Glycosylation essential: baculovirus or mammalian cells High yields, low cost: E. coli Post-translational modification: yeast, baculovirus, eukaryotes 4.3. Cell Culture In vitro analysis of multicellular eukaryotes Types: Primary: finite life span Continuous: abnormal, immortal Maintenance Growth environment: 37C, 5% CO2 Growth media: dependent on cell type General medium requirements: bulk ions, trace elements, sugars, amino acids, vitamins, choline, inositol, serum, antibiotics Primary cells Most closely represent, as it is directly taken from, tissue Advantages Avoids ethical objections contrast to in vivo Provides relevant results than cell lines Cost-effective Disadvantages Limited growth potential and eventually die Characteristics may change with subsequent passage Primary vs continuous Primary only last at most 20 weeks, have moderate consistency, but need higher cost to optimize culture conditions Continuous have relatively culture costs, but subject to genetic drift Primary can become continuous via transformation Continuous cells (Cell lines) Have inherent gene mutations that allow escape of cell proliferation regulation processes Makes them useful “workhorses” in most laboratories Stem cell lines Have the ability to self-renew or differentiate to various cell lines Cell culture transfection Introduction of foreign DNA into eukaryotic cells May be stable or transient Methods Calcium chloride + DNA = calcium phosphate complex will be endocytosed into the host cell, albeit low efficiency Lipofection uses lipid vesicles to transiently introduce DNA, about 30-40% efficiency Electroporation uses electronic pulses Goals Expression of protein product Analysis of effect of DNA on cell function 4.4. Genetically modified organisms (GMO) Also known as transgenic organisms Organism with altered genetic material using engineering DNA from different sources are combined into one (chimera) to create a new set of genes Traditional breeding vs genetic engineering Traditional: hybrid cross of domesticated and wild; transfer of other genes cannot be controlled Genetic modification: specific insertion of a gene Recombinant proteins, gene resistance, and enhanced properties Transgenic animals can be used for commercial large-scale production e.g. rhAT in transgenic goats, ANDi the first transgenic primate carrying GFP gene Bt–modified organisms allow for insecticidal effects without conferring human toxicity Golden rice engineered to produce beta-carotene, niacin, iron, essential minerals, etc and thus improve vitamin A content Rice lacks four enzymes to produce beta-carotene, the precursor of vitamin A Fruits e.g. virus-resistant papaya, mosaic-resistant squash, enhanced tomato, herbicide-resistant sugar beet, non-browning apple A5: Genome Editing 5.1. Vaccine Production Traditional method: making recombinant gene to produce recombinant protein as vaccine Kariko & Weissman: discovered that base-modified mRNA can be used to block activation of inflammatory reactions mRNA vaccines: delivery via lipid nanoparticles Allows transport through cell membranes Protects RNA from being broken down Moderna and Pfizer: used lipid nanoparticles with PEG (which make them last longer in the body) Limitation: need for low temperature Bivalent vaccines Advantage: you can make different variants of mRNA very rapidly mRNA vaccines for other diseases currently in clinical trials 5.2. Genome editing Eukaryotic model organisms Saccharomyces cerevisiae Drosophila melanogaster Caenorhabditis elegans Mus musculus Knockout mice Studying of genetic diseases and efficacy of treatments Classic ways of genome editing Site-directed mutagenesis Cassette mutagenesis Use of viral vectors Site-directed mutagenesis PCR-based technique of changing DNA at a desired position e.g. SNP, missense, deletion, etc. Determines effect of DNA sequence change on function Example: site-directed modified subtilisin have reduced activities Cassette mutagenesis Variety of mutations already introduced to gene of interest Use of viral vectors Random (anywhere in the genome) vs targeted insertion 5.3. Analysis of Gene Function RNAi or post-translational gene silencing Produces siRNAs that silence complementary sequence Degradation of mRNA blocks gene functions Function: immune response against foreign RNA Synthetic siRNAs Limitation: only produces atransient outcome as it only targets mRNA, allowing continuous transcription of the DNA sequence; siRNA-based treatments should be continuous Gene disruption/knockout Mutated gene is introduced to embryonic stem cell Normal gene “knocked out”by foreign gene via homologous recombination Gene knock-in Genome editing vs genetic engineering Genome editing: in situ alteration of DNA sequence DSB DNA repair mechanisms: NHEJ and HDR Genome editing mechanisms Zinc finger nucleases (ZFNs): zinc finger DBD anchored to engineered nuclease FokI which makes a nonspecific DS break TALENS gene editing - single nucleotide resolution: TALENs DBD (have longer recognition sequences than ZFNs) anchored to FokI CRISPR Variants: can induce gene silencing or enhance transcription Medical applications (gene knockout) Industrial applications Genome-edited crops Gene drives Systems of biased inheritance Potential uses: population suppression or replacement Gene drive mosquitoes engineered to fight malaria will pass resistance to Plasmodium to its offsprings Metabolic Engineering and Functional Food Research Volk et al. (2022) Chemical Reviews, 123 (9), 5521-5570. The Three Revolutions - Molecular biology (1953 - elucidation of DNA structure) - Genomics (1976 - emergence of Genentech) - Convergence Organism selection - Prokaryotic expression system is not fruitful for some eukaryotic molecules (e.g. hydrophobic membrane proteins) - There are “non-model” organisms for production of products such as itaconic acid and 2-propanol/acetone Strategies and tools - Strategies for strain design (knowledge-based design, evolutionary and combinatory, ) - Push-pull-block (push - increase flux, pull - decrease, block - remove competing pathways) - Directed and continuous evolution - Tools - Genome editing (classical vs CRISPR/Cas9) - Static vs dynamic regulation - Omics tools (NGA for DNA, RNA; LC-MS for proteins, metabolites) - Genome-scale models (GEMs) - Machine learning Applications - 1979: synthetic human insulin expressed in E. coli Functional food - foods that offer health benefits beyond their nutritional value - long-term effects are pronounced by long-term consumption (mostly disease prevention function) - Examples: Yakult, green tea - Obesity strongly associated with metabolic syndrome (diabetes) - Hypertrophic adipocytes - Natural products - Cyanidin 3-o-glucoside (Cy3G): black soybean seed coat extract prevented body weight gain and increase in white adipose tissues -Matsukawa et al (2015) Journal if Nutritional Biochemistry 40 (2017) 77-85. - Anthocyanin-rich foods: bignay, baligang or lipote, duhat - Purple sweet potato is rich in 3,4,5-tricaffeoylquinnic acid - Artemisinin: effective antimalarial agent - Flavonoids: olives’ phenolic compounds (apigenin-7-glucoside) on leukemia - Cell differentiation therapy (HL60: cancerous version of common myoloid progenitor)