Laser_1_merged.pdf
Document Details
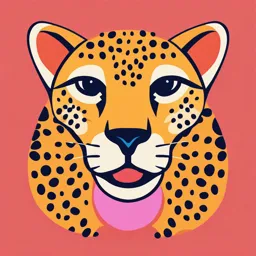
Uploaded by PanoramicRealism4179
Full Transcript
Laser Technology Master Class about Laser Technology How do lasers Questions: work? What is a laser? Laser Principle...
Laser Technology Master Class about Laser Technology How do lasers Questions: work? What is a laser? Laser Principle What types of laser are there used in modern industry What are the most critical process parameters? What are laser used for? How accurate can a laser cut? Can laser weld Stainless steel Is laser light Which materials can ? dangerous? be laser cut? Which thickness? Dr.-Ing Mahdi AMNE ELAHI, Prof. Dr.-Ing Peter PLAPPER Laser Technology About (Peter PLAPPER) Practical experience in planning, optimizing and demounting of laser systems. Worked in Europe and US. Head of Laser Technology Competence Center Completed 11 PhD supervisions (50% related to laser) 40 + publication related to laser welding Reviewer of EU research, journals, … Twitter: @PeterPlapper phone: +352 46 66 44 58 04 Mail: [email protected] Dr.-Ing Mahdi AMNE ELAHI, Prof. Dr.-Ing Peter PLAPPER Laser Technology About me (Mahdi AMNE ELAHI) Bachelor and master’s degree in material science and engineering Process engineer Head of QC and Lab. (currently Nik Aluminium Kaveh) Doctoral researcher (FNR-industrial fellowship grant) Post-Doctoral researcher Advanced product/process expert at Glass Competence Center LinkedIn: https://lu.linkedin.com/in/mahdi-amne-elahi-400a36b7 Google Scholar: https://scholar.google.com/scholar?hl=en&as_sdt=0%2C5&q=Mahdi+Amne+Elahi&btnG= Mail: [email protected] Dr.-Ing Mahdi AMNE ELAHI, Prof. Dr.-Ing Peter PLAPPER Laser Technology Structure of this course 1 Introduction 7 Quality 13 Welding 2 Fundamentals 8 Process defects 14 Brazing 9 Hybrid NC 15 Welding of 3 Beam sources machines dissimilar materials 4 Laser matter 10 Hybrid NC 16 Welding of interaction machines Polymers 11 Hardening & 17 Welding of other 5 Beam guidance softening materials 6 Safety 12 Cutting, drilling ablating 18 Summary Dr.-Ing Mahdi AMNE ELAHI, Prof. Dr.-Ing Peter PLAPPER Laser Technology Learning outcomes Qualification goals / acquired competencies: You know the different laser types and can explain the differences You can explain the creation and propagation of laser light You assess which laser beam source is suitable for which applications You can suggest concepts for new laser applications You distinguish parameters that influence absorption of laser beam energy You know which process parameters are most critical You can describe areas of industrial application of lasers You are informed about cutting edge research results and understand the scientific methods. Dr.-Ing Mahdi AMNE ELAHI, Prof. Dr.-Ing Peter PLAPPER 5 Lecture 1 Introduction to laser technology Agenda: Scope of this course Differences of laser light to “normal” light Main parts of a laser system Technological aspects Commercial aspects Learning summary Dr.-Ing Mahdi AMNE ELAHI, Prof. Dr.-Ing Peter PLAPPER 6 Literature: Laserunterstütztes Stanzen, Fraunhofer-Institut für Produktionstechnologie & WZL RWTH Aachen Emonts, M.: , Lasersystemtechnik, Fraunhofer-Institut für Produktionstechnologie & WZL RWTH Aachen Brecher, C.: Laserunterstütztes Fräsen hochfester Werkstoffe, Laserunterstütztes Zerspanen, Fraunhofer- Institut für Produktionstechnologie & WZL RWTH Aachen, 04/2011 Deutges, D.: Harte Keramik laserunterstützt drehen, WB Werkstatt+Betrieb, Carl Hanser Verlag, München, 09/2011 - Laserunterstütztes Scherschneiden, BLECH InForm, S.30-33, Carl Hanser Verlag, München, 03/2009 - Süzük, K.: Laserunterstütztes Drehen von Keramiken, Mechanisches Werkstoffverhalten, RWTH Aachen, - Kuhn, D.: Laserunterstütztes Gesenkbiegen, www.blechnet.de, 2009 Klocke, F.: Abtragen, Generieren Und Lasermaterialbearbeitung, Band 3, Springer Verlag, 2006 Brecher, C.: Laserbearbeitung, Fräsen, Keramik; Mehrachsige laserunterstützte Fräsbearbeitung; 2010 Brecher, C.: Produktionstechnik im nächsten Jahrzehnt – innovativ und integrativ, WZL & RWTH Aachen, 2010 Bausch, S.: Produktion Zerspanungstechnik, MM Maschinen Markt, Band 36, 2011 Michael, F.: Laseruntersütztes Fräsen reduziert die Prozesskräfte, Zerspanen, MM MaschinenMarkt, 01/2011 Zäh, M. F.: Laserunterstütztes Fräsen, Laserbearbeitung, Fräsen, NE-Metalle, Bundesministerium für Bildung und Förderung, wt Werkstattstechnik online, H.7/8, Springer-VDI-Verlag, 2011 Arntz, K.: Hybride Produktionstechnik, Wettbewerbsfaktor Produktionstechnik, AWK Aachener Werkzeugmaschinen Kolloquium, Shaker Verlag, 2011 LUGB: Laser-Unterstütztes Gesenk-Biegen mit integrierten Diodenlasern, Trumpf & TU Wien, 2009 Perrottet, D.: Zweckbündnis aus Licht und Wasser, Mikroproduktion, Synova, 2. Ausgabe, 2005 Nowotny, S.: Präzisionsschweißen mit laserbasiertem, hybriden Fertigungsverfahren, Fraunhofer IWS, Jahresbericht 2003 6 Difference laser light vs. “normal” light Laser light is - Monochromatic - Parallel - Coherent - High energy density „white“ light is - Polychromatic - Divergent - Different phase - Low-intensity Dr.-Ing Mahdi AMNE ELAHI, Prof. Dr.-Ing Peter PLAPPER A conventional light source, e.g. the sun or a light bulb, emits „white“ light (a mixture of all visible wavelengths) in all directions. The achievable energy density is some W/cm². 1. A laser beam source emits light of a single (narrow band of) wavelength, e.g. 1064 nm, thus, monochromatic light. 2. Due to the stimulated emission of photons, the light is coherent, all light waves are in the same phase. 3. Furthermore, the optical resonator of the laser beam source emits only parallel laser beams. Hence, a laser beam can be guided and formed very precisely and with its beam characteristics it is perfectly suited for the application in the production technology. Light intensities up to several MW/cm² can be achieved. 1.Temporal Coherence: The light waves maintain a constant phase relationship over time. This allows the laser to produce a very narrow spectrum of light, often of a single color or wavelength. 2.Spatial Coherence: The light waves are in phase across the beam's cross-section, allowing the laser to be focused to a very small spot and travel long distances without spreading out much. 7 Laser Technology Structure of manufacturing technologies according DIN 8593 Laser are used for almost all manufacturing technologies Laserbased manufacturing technologies Change Primary Forming Separating Joining Coating Material shaping properties Stereo- Laser- Cutting Welding Coating Hardening Lithography bending Drilling Brazing Additive Annealing Laser sintering Ablation Mfg. Laser Laser - generating assisted forming 8 Source: Institut S. Al-Sayyad, Kedziora, M Amne Soudure, Plapper, Univ. Elahi, 2016 Luxembourg, 2020 Plapper, 2020 2020 Dr.-Ing Mahdi AMNE ELAHI, Prof. Dr.-Ing Peter PLAPPER 8 Laser Technology Main parameters of a laser system Wavelength [nm … µm] Power [mW…kW] Pulse duration t [fs … ms] Energy density [J] Pulse repetition rate [Hz … MHz] Coherence length [mm..km] Polarization Focal position [mm] Beam diameter [mm] Divergence [mrad] Power and Energy density [W/cm2] [J/cm2] Spot size Standoff Source: edmundoptics.de Dr.-Ing Mahdi AMNE ELAHI, Prof. Dr.-Ing Peter PLAPPER 9 Laser Technology Laser material processing Typical manufacturing technologies texturing 109 Specific energy [J/cm²] 104 Power density [W/cm²] 108 ablation, drilling 10³ Plasma 107 welding vaporizing 10² 10 6 brazing 101 cutting melting 10 5 100 104 heating hardening 10-6 Modif. from Graf – Hügel and others 10-4 10 – 2 Interaction time [s] Dr.-Ing Mahdi AMNE ELAHI, Prof. Dr.-Ing Peter PLAPPER All of these effects are relevant during laser materials processing. There exist distinct combinations of laser intensities and interaction times where specific effect of laser–material interaction dominates. The figure presents one such plot showing the regimes of various laser–material interactions and their applications in materials processing. Dahotre, Harimkar: Laser Fabrication and Machining of Materials, 2008, Springer Science+Business Media 10 Laser Technology Laser material processing – Laser beam welding Welding depth penetration in 1.0 mm EN-AW1050A, P=400W, = 1075 nm Source: Université du Luxembourg Dr.-Ing Mahdi AMNE ELAHI, Prof. Dr.-Ing Peter PLAPPER 11 Laser Technology Laser market Global market development Source: Optec Consulting, internet Dr.-Ing Mahdi AMNE ELAHI, Prof. Dr.-Ing Peter PLAPPER 12 Laser Technology Laser market Revenue by technology Source: Industrial lasers, internet Dr.-Ing Mahdi AMNE ELAHI, Prof. Dr.-Ing Peter PLAPPER 13 Laser Technology Laser market Beam sources Source: Optec Consulting, internet Dr.-Ing Mahdi AMNE ELAHI, Prof. Dr.-Ing Peter PLAPPER 14 Laser Technology Laser application in Automotive industry Dr.-Ing Mahdi AMNE ELAHI, Prof. Dr.-Ing Peter PLAPPER 15 Laser Technology Introduction Learning control Name specialty of laser light. What is difference of laser beam vs. “normal” light? List main parameters of a laser system Allocate manufacturing processes as a function of power density and exposure time Which energy density is required for welding? Explain impact of increasing welding speed to welding depth penetration. Which are the three most frequent manufacturing technologies for laser? Dr.-Ing Mahdi AMNE ELAHI, Prof. Dr.-Ing Peter PLAPPER 16 Laser Technology Content Creating a laser beam Atoms, waves and light – electromagnetic radiation 1. Light as an electromagnetic wave 2. Polarized light 3. LASER: Light Amplification by Stimulated Emission of Radiation 4. Creation of Laser – Light 5. Characteristics of laser beam caustic 6. Difference of laser light and “normal” light 7. Learning control Dr.-Ing Mahdi AMNE ELAHI, Prof. Dr.-Ing Peter PLAPPER Polarized light refers to light waves in which the vibrations occur in a single plane. Normally, light waves vibrate in multiple planes, but when light is polarized, these vibrations are restricted to just one plane. There are a few ways light can become polarized: Reflection: When light reflects off a surface like water or glass, it can become polarized. Transmission through a Polarizing Filter: Special filters can block certain planes of light waves, allowing only light vibrating in one plane to pass through. Scattering: Light can become polarized when it scatters off particles in the atmosphere, which is why the sky appears polarized to some extent. Polarized light is used in various applications, such as reducing glare in sunglasses, improving contrast in photography, and in liquid crystal displays (LCDs). Atoms and light – Laser Laser light is - Monochromatic - Parallel - Coherent - High energy density „white“ light is - polychromatic - divergent - Different phase - Low-intensive Dr.-Ing Mahdi AMNE ELAHI, Prof. Dr.-Ing Peter PLAPPER A conventional light source, e.g. the sun or a light bulb, emits „white“ light (a mixture of all visible wavelengths) in all directions. The achievable energy density is some W/cm². 1. A laser beam source emits light of a single (narrow band of) wavelength, e.g. 1064 nm, thus, monochromatic light. 2. Due to the stimulated emission of photons, the light is coherent, all light waves are in the same phase. 3. Furthermore, the optical resonator of the laser beam source emits only parallel laser beams. Hence, a laser beam can be guided and formed very precisely and with its beam characteristics it is perfectly suited for the application in the production technology. Light intensities up to several MW/cm² can be achieved. 2 Light as electromagnetic wave Electromagnetic spectrum 400 nm 800 nm 790 THz 380 THz Source: http://imagine.gsfc.nasa.gov/docs/science/know_l1/emspectrum.html, Dr.-Ing Mahdi AMNE ELAHI, Prof. Dr.-Ing Peter PLAPPER The visible range in the electromagnetic spectrum is called light. The human eye can detect light in the wavelength range between 400 (blue) and 800 (red) nm (corresponding to frequencies between 790-380 THz) whereas these frequencies do not represent exact threshold values as the sensitivity of the eye decreases gradually. Neighboring spectral ranges (ultraviolet UV 10-400 nm and infrared IR 800-10,000 nm) also generally also called light. The electromagnetic spectrum also covers the short-wavelength gamma and X-ray range as well as the long-wavelength microwave and radio range. The shorter the wavelength, the higher the frequency and, thus, also the energy (E=h*f). 3 Laser Technology Light as electromagnetic wave Gas discharging emits light in a varity of frequencies lens red yellow green blue He gas discharging Dr.-Ing Mahdi AMNE ELAHI, Prof. Dr.-Ing Peter PLAPPER A discharging gas emits photons of the entire spectrum. If the light of these thermal emitters is separated with a prism or a grating spectrograph, a continuous spectrum with all colors can be observed As shown here schematically, the spectrum of a He gas discharge consists of a series of isolated and narrow emission lines. In contrast to black body emitters, the light-emitting atoms, and molecules experience only a very small interaction with the environment in a gas discharge at low pressure and thus reveal their natural quantum mechanical properties. Since the energy of a photon is proportional to its frequency, all photons of the same color (means with the same frequency or identical wavelength) have the same energy. The atoms in the gas under consideration can after their excitation by the gas discharge only release the absorbed energy in sharply defined portions. A diffraction grating works based on the principles of: 1.Diffraction – The bending and spreading of light waves when they pass through small openings or obstacles. 2.Interference – The phenomenon where diffracted light waves overlap and combine to produce constructive or destructive patterns. The key equation governing a diffraction grating is: dsinθ=mλ: d = spacing between adjacent slits (grating constant) θ = angle of the diffracted light m = diffraction order (1st order, 2nd order, etc.) λ = wavelength of light This equation shows that different wavelengths are diffracted at different angles, allowing a grating to separate white light into a spectrum of colors. Seite 4 Light as electromagnetic wave Propagation of a linearly polarized electromagnetic wave Electrical vector field E(z, t) Polarized @ 45o Dr.-Ing Mahdi AMNE ELAHI, Prof. Dr.-Ing Peter PLAPPER Since the wave equations are linear, each superposition of such electromagnetic waves is possible. By adding two orthogonally polarized waves to each other, further polarization states can be generated depending on the phase difference. The in-phase addition of two plane waves polarized in the x and y directions with the same amplitude results in a plane wave with a linear polarization rotated by 45 ° from the x to the y axis. Conversely, any plane wave in space with linear polarization can be broken down into two orthogonal components 6 Light as electromagnetic wave Polarized light Circular polarized Superposition of = /4 2 electric waves Ey and Ex Same frequency, different phase (/4) => Circular polarized Ey Ex Dr.-Ing Mahdi AMNE ELAHI, Prof. Dr.-Ing Peter PLAPPER If there is a phase shift between the two orthogonally polarized components, the field vectors in a snapshot generally form an elliptical spiral (left). If you consider the temporal behavior of the same wave at a fixed location, the tip of the field vector rotates along an ellipse that is perpendicular to the direction of propagation. One speaks here of an elliptically polarized wave. The special case of circularly polarized waves occurs when the phase shift is just a quarter of a wavelength, i.e. = / 2. This polarization state, which is particularly important for material processing, can be generated from a linearly polarized wave using a so-called / 4 plates. It consists of a crystal (e.g. CaCO3, quartz, ZnO, CaSe, BaTiO3) that is parallel and perpendicular has different refractive indices nx and ny to its optical axis. If the optical axis of the crystal is aligned by 45 ° to the direction of polarization of the incident wave, the two components of the wave polarized in the x and y directions experience a different phase delay, which is exactly at / 4 with a suitable thickness of the crystal as shown on right side. The wave generated in this way is then exactly circularly polarized. 7 Light as electromagnetic wave Polarization of light Circular polarization by λ/4 plates Axis of polarization of incoming beam circular polarized optical retardation linear polarized Optical axis of crystal Rotated polarization with λ/2 plates Axis of polarization of incoming beam right circular Changed axis of left circular polarized polarized polarization linear polarized Source: Eichler: Laser, 7.ed, 2010, Springer Berlin Heidelberg Optical axis of crystal Dr.-Ing Mahdi AMNE ELAHI, Prof. Dr.-Ing Peter PLAPPER The stimulated emission phenomenon not only produces long trains of waves but these waves will also have their electric vectors all lined up. The beam is thus polarized. Many of the early lasers (esp. CO2) and some of the more modern ones which do not have a fold in the cavity will produce randomly polarized beams. In this case the plane of polarization of the beam changes with time – and the cut quality may show it! To avoid this it is necessary to introduce into the cavity a fold mirror of some form. Outside the cavity, such a fold would make no noticeable difference. Inside the cavity, it is a different matter since the cavity is an amplifier and hence the least-loss route is the one being amplified in preference to the others – in fact almost to their total exclusion. Polarized beams have a directional effect in certain processes, for example, cutting. This means that the quality of the laser- cut parts is different depending on the polarization of the beam. Hence, CO2 lasers for material processing are usually engineered to give a polarized beam which is then fitted with a circular polarizer. Polarization plays a role in the reflection and scattering of all light. If the electric vector is all aligned in one direction, then the beam is “linearly polarized”. If it has two vector directions at right angles to each other of equal intensity, it is said to be “circularly polarized” – if the field rotates clockwise to an observer looking into the beam then it is said to be “right-circular polarized” as opposed to “left- circular polarized”. If one vector is larger than the other, the beam is “elliptically polarized”. The “extinction ratio” is the ratio between the maximum and minimum intensities of the beam after passing through a polarization filter. Other modern lasers like diode, disc or fiber lasers provide non-polarized laser beams. Source: Steen, Mazumder: Laser Material Processing, 4th ed., 2010, Springer London 8 Manufacturing Technology Basic Principles of Laser Operation Lasers operate based on the principles of quantum mechanics and electromagnetic radiation. The fundamental processes involved in laser action are: - Energy Absorption (Excitation): Atoms or molecules in a material (called the gain medium) absorb external energy (from an electrical current, another light source, or chemical reaction) and move to an excited state. - Spontaneous Emission: After some time, the excited atoms naturally return to their original lower energy state by emitting photons (particles of light). This emission is random in direction and phase. - Stimulated Emission (Key to Laser Action): If a photon of specific energy interacts with an excited atom, it can trigger the release of another photon of identical energy, phase, and direction. This process leads to a chain reaction, amplifying the light. - Optical Cavity & Light Amplification: The photons bounce between two mirrors placed at the ends of the gain medium, stimulating further emissions and amplifying the light. One mirror is partially transparent, allowing a focused beam of coherent light to exit as the laser output. Seite 9 Laser Technology Atoms and light Laser light emission 1. Absorption of energy (Pumping) 2. Spontaneous emission of photon 1. 2. 3. 3. Spontaneous emission, in preference direction h.f21 4. Stimulated emission of light, amplified E0 E1 E2 h.f21 2.h.f21 3.h.f21 E2: Upper energy level (instable) E1: intermediate Energy level (instable) E0: lower level (stabile) 4. h.Fxy: Energy difference for transition between energy levels Energetic status of electrons before optical transition Energetic status of electron after optical transition preferred direction Modified from : F. Klocke Dr.-Ing Mahdi AMNE ELAHI, Prof. Dr.-Ing Peter PLAPPER Through Einstein’s analysis of radiation from hot objects, he postulated that there must be a radiant term based on a photon of radiation striking an excited species and causing it to release the energy of excitation. This theory has since then been shown to be true. The stimulated photons are found to be in phase (=coherent) and travelling in the same direction (=parallel) as the stimulating photons. A photon originates from the energy change between an excited state and a lower state. It is thus usually spectrally pure (=monochromatic) if the change is between electronic or vibrational quantum states, but may have a range of wavelengths if the change is from an energy well to a lower state (as with Excimer lasers, Diode lasers, Titanium sapphire lasers and others). Atomic systems (atoms, ions or molecules) can exist in certain states which are characterized by definite excitation energy levels. Each orbit occupies such an energy level. A transition between energy states (E1 to E2) results in the absorption or emission of a photon with its characteristic frequency ν12 which is given by h*ν12 =│E1 - E2│ Where h is Planck‘s constant. A system in the lower (ground) state may absorb photons of the appropriate frequency to rise to a higher state. Einstein identified three major processes by which the atoms can interact with an electromagnetic field: 1. spontaneous emission; 2. absorption; 3. stimulated emission. These three processes are illustrated for a two-level system separated by h*ν energy. Many materials can be made to show this stimulated emission phenomenon, but only a few have significant power capability, since a further condition is that a population inversion is necessary, whereby there are more atoms or molecules in the excited state than in the lower-energy state, so as to allow amplification as opposed to absorption. To achieve this, the lifetime of the excited species has to be longer than that of the lower-energy state. The main laser materials are carbon dioxide (CO2), carbon monoxide (CO), neodymium-doped yttrium aluminum garnet (YAG; Nd:YAG), Neodymium glass (Nd:glass), Ytterbium-doped YAG (Yb:YAG), Erbium-doped YAG (Er:YAG), Excimer (KrF, ArF, XeCl) and Diode (GaAs, GaAlAs, InGaAs, GaN and others being developed) lasers. From: Steen, Mazumder: Laser Material Processing, 4th ed., 2010, Springer 10 Laser Technology Atoms and light Spontaneous laser emission Creation of inversion and pumping of energy Two energy levels Three energy levels Four energy levels (Laser) (Laser) I= irradiated energy intensity E3 pumped energy level Fast, transition w/o radiation transition w/o radiation (fast) Absorption = stimulated emission With two energy levels no laser beam can be stimulated At least three levels (Ruby and Excimer Laser) or 4-levels (Nd:YAG Laser) are required Dr.-Ing Mahdi AMNE ELAHI, Prof. Dr.-Ing Peter PLAPPER Population inversion is a necessary condition for stimulated emission. Without population inversion, there will be net absorption of emission instead of stimulated emission. Population inversion corresponds to a non-equilibrium distribution of electrons such that the higher energy states have a larger number of electrons than the lower energy states. The process of achieving the population inversion by exciting the electrons to the higher energy states is referred to as pumping. The population inversion explained here for the two-level energy systems is only for the introduction of the concept. In actual practice, it is impossible to achieve the population inversion in two-level energy systems. Population inversion in most of the lasers generally involves three- or even better four-level energy levels. For a three-level energy system, electrons are first pumped from energy level E0 to E2 by the absorption of radiation (of frequency, ν = (E2 − E0)/h) from an energy pumping source. The lifetime of the electrons in the higher energy level E2 is generally very short and the electrons from the energy level E2 rapidly decay into metastable energy level E1 without any radiation (radiationless decay). Thus, the net population inversion is achieved between the energy level E1 and E0, which is responsible for the subsequent emission of laser radiation. Similar mechanisms cause the population inversion between the energy levels E2 and E1 in four-level energy laser. Example of 4-level-lasery-system is a CO2 Laser and Nd:YAG Laser three level is an Excimer laser. Dahotre, Harimka: Laser Fabrication and Machining of Materials, 2008, Springer Science+Business Media 11 Laser Technology Atoms and light “monochromatic” emission Bands of energy cause a range of frequency of laser beam Radiated E2 Frequency- E2 spectrum Energy levels E [J] Intensity I [Wcm²] 21 E1 E1 20 f 10 E0 E0 f10f f10 f10f Frequency of radiation f [Hz] range of energy energy level Eo + E1 f > Eo initial level of electrons (stable) h E2 Metastable intermediate level of energy E1 level of inversion (unstable) Dr.-Ing Mahdi AMNE ELAHI, Prof. Dr.-Ing Peter PLAPPER In the active medium, which is the core of any laser, the beam is created. The name of the laser beam source usually is derived from the active medium. The active media are commonly used in gaseous, solid and liquid state, as listed before. The active media have a meta stable energy level, where the excited electrons can remain quite long (up to one second) before falling to the base level of energy. The difference of energy emitted at the transition between the two levels is specific to the active material. A metastable level E1 can only be reached indirectly through higher levels of energy. In real live the levels of energy are not discrete, but are more like bands of energy, as they come with some bandwidth. This leads to a difference in the amount of emitted energy, when the electrons transition from any level to another. Thus, as a consequence the energy has a variation, which means that the laser beam frequency also has a variation. This is relevant for laser beam source development, but due to its minimal extend this effect is usually neglected by the engineers. Seite 12 Laser Technology Atoms, waves and light – Coherence Temporal coherence constant Coherence = const. random Coherence = var. f => Dr.-Ing Mahdi AMNE ELAHI, Prof. Dr.-Ing Peter PLAPPER Two electromagnetic waves, or two parts of a wave field, are called coherent if they have a defined phase or path difference. If, on the other hand, the phases of the fields change in a random manner relative to one another, the waves are incoherent (left). The coherence shown here is called temporal coherence. The stimulated emission phenomenon means that the radiation is generating itself and in consequence a continuous waveform is possible with low-order mode beams. The length of the continuous wave train may be many meters long. The comparison of laser light with standard random light is illustrated on the left side. This long coherence length allows some extraordinary interference effects with laser light such as length gauging, speckle interferometry, holography and Doppler velocity measurement. This property has not yet been used in material processing. In years to come it may be that someone will be able to use it as a penetration meter or to carry out subtle experiments with interference-banded heat sources. Seite 13 Laser Technology Atoms, waves and light Stimulated emission in Laser Source of pumping energy Non amplified radiation usable laser beam Amplified radiation Complete reflective Laser active material Partially mirror transmissible resonator mirror Dr.-Ing Mahdi AMNE ELAHI, Prof. Dr.-Ing Peter PLAPPER The positioning of the laser active material between two parallel mirrors, the so- called resonator, provides the radiation with a preferred directions. Every photon, which is reflected orthogonal to the mirrors, is reflected into the laser active material, where it can stimulation the emission of more photons. This leads to an amplification of the radiation in the axis of the resonator. To ensure the light of the laser beam is in the same phase, the distance of the two mirrors must be multiples of an integer of the wavelength. Thus, the phase and wavelength of the radiation are identical, which are two of the characteristics of a coherent and monochromatic light beam. To use the light, a part of the beam is coupled out by a partially reflective mirror, leaving appropriate number of photons in the resonator to keep the stimulations of the electrons in balance. Seite 14 Laser Technology Atoms, waves and light Longitudinal laser modes Requirements for an operative Laser device Laser active medium required for light amplification Inversion must be continuously maintained via pumping Part of the amplified light wave must be fed back into the active medium in the correct phase Dr.-Ing Mahdi AMNE ELAHI, Prof. Dr.-Ing Peter PLAPPER In the laser medium, the radiation is stimulated by the supply of energy. A lamp or a diode or high-frequency voltage is usually used as the pump source. It pumps the required energy into the laser medium. The laser medium (or laser material) is either gas (e.g. CO2) or liquid or a solid (e.g. a doped YAG crystal). By introducing energy into the active medium, a population inversion is generated and maintained there (the pumping). By supplying energy to the pump source, the laser medium is stimulated to emit radiation. In the resonator, which is usually formed by two mirrors, this radiation is now reflected several times and thus passed through the active laser medium. Due to the multiple and long path between the mirrors, the parallel part of the light emerges from the laser as a parallel, coherent laser beam of one wavelength. Coherence in a laser beam refers to the property that allows the light waves to be in phase with each other over a certain distance or time. This coherence can be divided into two types: 1.Temporal Coherence: This describes how consistent the phase of the wave is over time. It is related to the monochromaticity (single wavelength) of the laser light. A laser with high temporal coherence can produce a very narrow spectral linewidth. 2.Spatial Coherence: This describes how consistent the phase of the wave is across different points in space. It allows the laser beam to stay focused over long distances and produce sharp interference patterns. Together, these properties make laser light highly directional and capable of maintaining its intensity over long distances, which is why lasers are used in applications like holography, optical communication, and precision measurements. 15 Laser Technology Atoms waves and light – Laser beam blind 𝜆 𝑠𝑖𝑛 𝜃 = 𝑘 𝑑 k= ±1,±2,±3, … Dr.-Ing Mahdi AMNE ELAHI, Prof. Dr.-Ing Peter PLAPPER A decent model for a laser beam, leaving the laser device through the blend of the partially transmissive mirror, shown on the last slide, can be obtained by looking at a plane wave that is laterally limited by a blind with a hole as depicted here. This creates a beam with constant intensity over the cross section. Experiments show that such a rectangular intensity profile is not retained behind the diaphragm. At large distances (z> d2 / λ) a broadened rounded profile with secondary maxima is created. The change in the intensity profile of a light wave behind the hole of the blind is diffraction. According to the Huygens principle, this is explained by the fact that every point of the aperture emits spherical waves. The spherical waves add up in the right half-space behind the cover to a resulting field distribution. When adding, the respective path difference or the phase of the spherical waves must be considered. If the path difference is zero, e.g. on the axis of symmetry, the amplitudes of the waves add up and an intensity maximum arises. If the path difference is λ / 2, the waves eliminate each other because the amplitudes are directed in opposite directions. This is in the directions with the angle θ. This is called spatial coherence. The most important feature of coherent rays is their interference. Incoherent rays show no interference. Seite 16 Laser Technology Laser Resonator Impact of losses at mirrors due to non-perfect reflectivity (Ri0 Quelle: Donat, Uni Paderborn Dr.-Ing Mahdi AMNE ELAHI, Prof. Dr.-Ing Peter PLAPPER The left small figure shows how a plane electromagnetic wave with the initial intensity S0 coming from the vacuum. It hits an absorbing media with the absorption coefficient γ2. When passing through the length x in the medium, the intensity drops exponentially and when leaving the medium it has the intensity S (x), which no longer changes in the vacuum and remains constant. Now to the the laser cavity, on the right side : Whenever a beam of intensity P transfers a laser cavity of the length L with absorption , the intensity of the beam is reduced exponentially with and L. The reflectivity of the Mirror Ri weakens the beam at each reflection. After multiple reflections, only the portion (1-Ri) leaves the laser cavity. Seite 18 Laser Technology Atoms waves and light - Transversal modes Amplitude distribution of the Hermite- Alternative explanation of transversal modes: Gaussian modes for first transversal orders Classic light beam makes several rounds in laser cavity until it returns to starting point Applications: TEM₀₀: Used in applications requiring high beam quality, such as precision cutting, medical procedures, and scientific research. Higher-Order Modes: Sometimes used in applications where beam shaping is required, but generally less desirable due to lower beam quality. Dr.-Ing Mahdi AMNE ELAHI, Prof. Dr.-Ing Peter PLAPPER In addition to the longitudinal mode structure, resonators also have a transverse mode structure. The individual modes differ in the number of knot lines (see left half of the picture). The right part of the picture shows an alternative way of looking at things: with higher modes, the light as a classic beam has to make several revolutions inside the resonator until it is back at the starting point. TEM stands for Transverse Electromagnetic Mode, which describes the pattern of the electromagnetic field in the cross-section of a laser beam. The most common and fundamental mode is TEM₀₀. TEM₀₀ Mode: Description: The TEM₀₀ mode is the lowest-order transverse mode and has a Gaussian intensity distribution. This means the beam has a single, central peak of intensity that decreases smoothly and symmetrically in all directions from the center. Characteristics: It has the highest beam quality and is often referred to as a Gaussian beam. It is ideal for applications requiring precise focusing and minimal beam divergence. Higher-Order TEM Modes: Higher-order TEM modes are described by two indices, m, and n, which indicate the number of intensity minima in the horizontal and vertical directions, respectively. TEM₁₀ Mode: Description: This mode has one intensity minimum along the horizontal axis, resulting in two peaks of intensity. Appearance: The beam profile looks like two bright spots side by side. TEM₀₁ Mode: Description: This mode has one intensity minimum along the vertical axis, resulting in two peaks of intensity. Appearance: The beam profile looks like two bright spots one above the other. TEM₁₁ Mode: Description: This mode has one intensity minimum along both the horizontal and vertical axes, resulting in four peaks of intensity. Appearance: The beam profile looks like a grid of four bright spots. Higher-Order Modes (e.g., TEM₂₀, TEM₀₂, TEM₂₁): Description: These modes have more complex patterns with multiple intensity minima and peaks. Appearance: The beam profiles become increasingly intricate with more bright spots arranged in various patterns. Seite 19 Laser Technology Atoms waves and light - Transversal modes Gauß-Hermite-Modes Gauß-Laguerre-Modes m p I= Imax TEM 00 TEM 10 TEM 00 TEM 10 n l TEM 01 TEM 11 TEM 01 TEM 11 I= 0 TEM transversal electro-magnetic mode. Quelle: R. Poprawe Dr.-Ing Mahdi AMNE ELAHI, Prof. Dr.-Ing Peter PLAPPER The geometry of the resonator has a great influence on the intensity and power density distribution of the emitted laser beam. Through the Laser-active material, Light is reflected multiple times and forms a stationary electromagnetic wave inside the resonator. Both the shape (round or rectangular) and the dimensions of the resonator (distance, diameter or width, and height as well as the radii of curvature of the mirrors) about the magnitude of the wavelength significantly determine the resulting distributions of the electric field strength, which are called (vibration) modes. The indices rn and n indicate the number of zero points of the vertical field strength distribution, and the index q is the number of field strength maxima along the axis inside the resonator. Since the intensity distribution over the beam cross-section is determined by the first two indices, the abbreviated notation TEMmn is often found. In addition to the indices m and n, for Cartesian symmetric distributions, the indices p and l (TEM pl) are also used if polar symmetric distributions are present. The basic mode TEM00 and the ring mode TEM01 are the most important for CO2 lasers. In this basic mode, which is also referred to as Gaussian mode, the radiation intensity is highest precisely on the beam axis. Higher modes have intensity minima due to interference phenomena, as shown here. The TEM01 is called ring mode and lies in a ring around the beam axis, where it has an intensity minimum exactly on the beam axis. Seite 20 Laser Technology Atoms waves and light - Transversal modes Lateral intensity distribution of CO2 laser beam Beam intensity distribution in Plexiglas: TEM00 Dr.-Ing Mahdi AMNE ELAHI, Prof. Dr.-Ing Peter PLAPPER The laser beam has a characteristic intensity distribution (energy density per cm²) in cross section and longitudinal section to the beam axis. This intensity distribution in the cross section of the laser beam is called mode and can be made visible in the mode shot. The unfocused beam is directed onto the surface of a plexiglass, which it burns. This creates a characteristic depression, which corresponds to the intensity distribution over the cross-section of the laser beam. Of main importance is the basic or Gaussian mode with TEM00, which has a continuous intensity distribution in the form of a Gaussian distribution. The intensity decreases evenly with the distance from the beam axis and corresponds to a Gaussian normal distribution (see picture). The beam intensity distribution of real laser sources rarely corresponds to an ideal Gaussian distribution. High-power lasers in particular emit radiation with many modes of different orders. Seite 21 Laser Technology Atoms, waves and light - Laser device Shape of the mirrors Concave mirror Laser active material Concave mirror resonator Dr.-Ing Mahdi AMNE ELAHI, Prof. Dr.-Ing Peter PLAPPER The Mirrors are concave to collect the laser beam rays. The exact geometry is parabolic, the radius of the mirrors very small vs. length of resonator. Reference to front of waves in 3 slides. Seite 22 Atoms, waves and light –Caustic of laser light laser beam lens In the focus is a finite energy density Dr.-Ing Mahdi AMNE ELAHI, Prof. Dr.-Ing Peter PLAPPER The light irradiates linearly. The superposition of all rays forms the beam caustic. The focus diameter is not zero but has an infinite radius wo. Far away from the lens, the caustic diverges to a hyperbole with a constant opening angle This angle correlates to the first minimum behind the blind of the semi-transparent mirror, discussed 2 slides before. There are two reasons why a lens will not focus to a theoretical point; one is the diffraction- limited problem and the other is the fact that a spherical lens does not have a perfect shape. Most lenses are made with a spherical shape since this can be accurately manufactured without too much cost and the alignment of the beam is not so critical as with a perfect aspherical shape. The net result is that the outer ray entering the lens is brought to a shorter axial focal point than the rays nearer the center of the lens. This leaves a blur in the focal point location. The plane of best geometric focus (the minimum spot size) is a little short of the plane of the planar wave front – the paraxial point. 23 Atoms, waves and light –Caustic of laser light laser beam Not to scale lens Beam radius at position z Rayleigh length zR w 𝑧 = 2𝑤 Beam parameter BPP product Source: Eichler: Laser, 7.ed, 2010, Springer Berlin Heidelberg Dr.-Ing Mahdi AMNE ELAHI, Prof. Dr.-Ing Peter PLAPPER A raw laser beam diverges by diffraction from its initial waist diameter of D0 at a constant angle and reaches a maximum diameter only at infinity. This maximum value is the far-field divergence angle Θ0∞. If a lens focuses the beam, it forms a new waist, w1. The beam converges towards and diverges away from this new waist with a far-field divergence of Θ1∞ where w0Θ0∞ = w1Θ1∞ = constant. This constancy of wΘ values through the system with error-free optics allows the calculation of beam radius, depth of focus, Rayleigh length and curvature of phase fronts of the beam. The product of beam waist radius and divergence angle is also called beam parameter product (BPP, German: Strahlparameterprodukt SPP). The usual units are mm mrad (millimeters multiplied with milliradians). The BPP is the key parameter to specify the quality of a laser beam: a smaller beam parameter product correlates with a better beam quality. The BPP can also be defined for non-Gaussian beams. In that case, second moments should be used for the definitions of beam radius and divergence. The smallest possible beam parameter product is then achieved with a diffraction-limited Gaussian beam; it is λ/π. For example, the minimum beam parameter product of a 1064-nm beam is ≈ 0.339 mm mrad. Steen, Mazumder: Laser Material Processing, 4th ed., 2010, Springer London 24 Laser Technology Atoms, waves and light –Caustic of laser light w(z) zR w 𝑤 Θ ≅ tan(Θ) = Beam waist radius w 𝑧 Beam divergence angle in far field Beam Parameter Product BPP= o. w Dr.-Ing Mahdi AMNE ELAHI, Prof. Dr.-Ing Peter PLAPPER Laser beams are directed, but the rays of the light are not perfectly parallel. The laser beam has a beam waist and then widens slightly. The diameter of the beam waist is the narrowest beam cross-section which, together with the opening angle, and the divergence, defines the propagation behavior of the laser light. Rays with a small waist and little divergence can be focused on a smaller spot and at the same time have a greater depth of field. The working distance between the lens and the workpiece can therefore be relatively large, which is the aim when used in production. Smaller opening angles mean higher beam quality. The lowest possible can only be achieved by laser beams with fundamental mode TEMoo and is a function of the wavelength. The beam parameter product (BPP) or the M² value (diffraction index) indicates the quality of the beam in accordance with ISO 11145. With the beam parameter product, the two most important and easily measurable beam parameters are multiplied by each other: the radius of the waist and half the opening angle (divergence angle). Note: The smaller the beam parameter product, the higher the beam quality. Seite 25 Laser Technology Atoms, waves and light –Caustic of laser light radius r [mm] w(z) w(2.zR)= 5. w0 Beam radius w(z) w0 w(zR)= 2. w0 Distance from beam waist z [mm] Beam waist radius w o z=0 z = zR z=2.zR Far field divergence o Lateral intensity distribution of gaussian beam Intensität I [Wcm²] l(0,0)= Imax Rayleigh length zR l(r,0) 2.w0 w(zR)= 2. wo l(0,zR)= 0,5.Imax l(r,zR) l(0,2.zR)= 0,25.Imax 2. 2. w0 l(r,2.zR) Beam parameter product 2. 5.w0 BPP= o. wo 0 radius r [mm] Definition of beam radius intensity I [Wcm²] BPPideal = SPP Gauß = l(0,z) 8 l(r,z)TEM00 86,5% l(r,z)dr l(r,z)TEM10 2.w(z) 8 TEM00 1. l(0,z) 2.w(z)TEM10 e² 0 radius r [mm] Dr.-Ing Mahdi AMNE ELAHI, Prof. Dr.-Ing Peter PLAPPER The geometry of a laser beam is described as a function of the position on the beam axis by the outer boundary of the beam perpendicular to the beam axis. The term beam caustic describes the area surrounding the laser beam. Even the geometry of real laser beams can be easily described by a few easily determinable metrics. Shown is the beam caustic of an initially parallel, laterally limited, and freely propagating laser beam. As a result of the diffraction of light, the freely propagating laser beam is widened. The widening (divergence) approaches a limit value, which is referred to as the far field divergence o and corresponds to half the opening angle of the resulting beam cone. The beam waist radius is the smallest beam radius where the beam is straight parallel to the beam axis and the cross-sec onal area of the beam is minimal. The distance to the beam waist is described by the axis coordinate z. The beam radius w (z) (beam caustic) diverging along the beam axis describes the enveloping beam geometry as a function of the distance to the beam waist. Due to the asymptotic decrease in intensity in the radial direction, a suitable definition is needed to determine the beam radius. In practice, the following definition applies: The beam radius is as large as a pinhole, which lets 86.5% of the emitted laser power through. For the ideal Gaussian beam, this practical parameter agrees with the mathematically precise definition. The Rayleigh length zR indicates the axial distance z in which the cross-sec onal area (!) of the beam has doubled. Two times the Rayleigh length is called the focal length or depth of focus field. At the focal point of focusing optics, the depth of field is a measure of the area with approximately parallel beam propagation. The beam waist radius w0 and the far field divergence o are of particular importance in practice, since the product of the two metrices, the so-called beam parameter product SPP (BPP for Beam Parameter Product) is a constant and characteristic parameter for the quality of the radiation emitted by a laser. The beam parameter product of the ideal Gaussian beam depends only on the wavelength of the laser beam and is proportional to it. High-frequency radiation thus achieves a higher beam quality than low-frequency radiation SPP = Seite 26 Laser Technology Atoms, waves and light –Caustic of laser light laser beam lens Phase front of the electromagnetic waves Dr.-Ing Mahdi AMNE ELAHI, Prof. Dr.-Ing Peter PLAPPER Seite 27 Laser Technology Laser beam diagnostics Quantitative Measuring of laser beam energy: A part of the laser beam is directed onto energy detector Absorption of the beam Heating can be calculated to energy of beam Principle of calorimetric beam dump Dr.-Ing Mahdi AMNE ELAHI, Prof. Dr.-Ing Peter PLAPPER Seite 28 Laser Technology Laser beam diagnostics Quantitative Dr.-Ing Mahdi AMNE ELAHI, Prof. Dr.-Ing Peter PLAPPER Measurement of the beam intensity profile A very small part of the laser beam is directed onto the detector. The power distribution is recorded directly by the CCD array or by scanning. Types Camera-based detectors (CCD, pyroelectric) Scanning detectors (aperture, slot, knife-edge) 29 Laser Technology Laser beam diagnostics Quantitative Measurement of beam profiles with CCD- sensor Top right: TEM00 – Mode of HeNe-Laser Bottom: Lasermode of Nd:YAG Laser 120 W Dr.-Ing Mahdi AMNE ELAHI, Prof. Dr.-Ing Peter PLAPPER 30 Laser Technology Laser light Learning control Light is an electromagnetic wave Polarization of electromagnetic waves Generation of stimulated laser emission (pumping, inversion, stimulation, emission) Longitudinal and transversal modes Characteristics of laser light vs. „normal“ light What is coherence? Why is laser light monochromatic? (at least 2 reasons / impacting factors) Why is laser beam parallel? Explain beam caustic, focus diameter, beam opening angle Explanation, definition and formula of beam parameter product (BPP) What is Raleigh length and why is it relevant for use of laser light in manufacturing? Dr.-Ing Mahdi AMNE ELAHI, Prof. Dr.-Ing Peter PLAPPER Seite 31 Manufacturing Technology TEM 00 Laserstrahl Source: Pedrotti, Springer Dr.-Ing Mahdi AMNE ELAHI, Prof. Dr.-Ing Peter PLAPPER Seite 32 Laser Technology Content 2. Atoms and light Characteristics of light (visible spectrum, polarized light, magnetic and electrical field,..) Creation of laser light Caustic of laser beam and how to describe it (Quality of beam, Rayleigh length, …). Difference between “normal” light and laser light 3. Laser units for production Components of a laser system Laser beam sources –Gas Laser –Solid state lasers Ruby laser Nd:YAG Fiber laser Disc Laser Diode laser VCSEL Dr.-Ing Mahdi AMNE ELAHI, Prof. Dr.-Ing Peter PLAPPER Laser units for production – Laser beam sources / active material + energy pump Source: ISO 11145:2006 Dr.-Ing Mahdi AMNE ELAHI, Prof. Dr.-Ing Peter PLAPPER laser unit one or more laser assemblies together with handling, measurement and control laser assembly laser device complemented with beam handling and forming components (usually optical, mechanical or electrical components) laser device laser where the radiation is generated, together with essential additional facilities (e.g. cooling, power and gas supply) that are necessary to operate the laser laser Active laser medium generating coherent radiation by means of stimulated emission Source: ISO 11145:2006 2 Laser units for production – Laser beam sources Optical resonator partially transmitting mirror Cooling unit Quelle: Eichler, Laser, 7.ed, 2010, Springer Berlin Heidelberg Dr.-Ing Mahdi AMNE ELAHI, Prof. Dr.-Ing Peter PLAPPER A laser device generally consists of four main components: - Laser active material, which amplifies the stimulated radiation - Optical resonator, composed of the active material and two mirrors - Pumping source, providing energy to the electrons of the active material - Cooling unit, to avoid overheating due to pumping of energy into the active material The optical resonator generally consists of a 100% reflective mirror and a partially transmitting mirror where the coherent laser beam is coupled into the beam guidance. The pumping source can either of be chemical, thermal, optical or electrical nature. The cooling can be air/air, water/air, water/water, either internally or externally. For industrial use cooling is generally done by an external water/water device. 3 Laser Technology Laser beam sources: Ruby laser Historic laser Flash pumped Al2O3 with substitute of 1% of Al3+ by Cr3+ 3 Niveau laser ruby bar flash lamp electrode electrode Dr.-Ing Mahdi AMNE ELAHI, Prof. Dr.-Ing Peter PLAPPER T. H. Maiman achieved the first experimental realization of a laser in 1960 with a flashlight-pumped ruby. Today, however, this laser is only of historical importance and is mentioned here for this reason. The optical excitation of the ruby laser takes place via the two absorption bands in the green and blue spectral range. The absorption bands are wide enough to enable excitation using flash lamps. The excitation of the first ruby laser took place with a flashlight, which was arranged in a spiral around the ruby rod, as shown here. The two polished end faces of the ruby stick of Maiman were partially reflective and thus formed the optical laser resonator. 4 Laser beam sources: CO2 laser Excitation modes of CO2 Gas molecules Source: Hügel, Graf Springer Dr.-Ing Mahdi AMNE ELAHI, Prof. Dr.-Ing Peter PLAPPER The CO2 laser is mainly used for material processing, as it can generate beams from a few 10 W to several kW with an almost diffraction-limited beam quality. CO2 lasers emit radiation at a wavelength of 10.6 μm. This is enabled via transition between two vibronical states of the linear CO2 molecule. A special feature of the CO2 laser is that the laser transition does not take place between energy states of the electrons, like discussed earlier in this class, but between vibratory energy levels. As shown here, the CO2 molecule, which is linear, can have three different vibrations modes. In the symmetrical stretching vibration v1, the two oxygen atoms swing back and forth symmetrically to the carbon atom. The flexural vibration v2, in which the molecule bends in a V-shape, is degenerate twice due to the two degrees of freedom of direction. In the asymmetrical stretching vibration v3, one oxygen atom and the carbon atom always move alternately towards each other, while the other oxygen atom shifts outwards. Like the energy of the electrons, the energies of these molecular vibrations are also quantized and can only assume discrete energy values. Since with these oscillations charges such a molecule acts like a microscopic antenna and can absorb or emit electromagnetic radiation (DC or HF, like shown on next page). 5 Laser beam sources: CO2 laser DC & HF / RF excitation Direction of flow outcoupling electrode electrode mirror mirror Longitudinal flow Transversal flow Source: Hügel, Graf Springer Dr.-Ing Mahdi AMNE ELAHI, Prof. Dr.-Ing Peter PLAPPER For CO2 lasers the electron excitation takes place either in a direct current discharge (DC) or in a high frequency discharge (HF or RF for radio frequency), whose frequency is usually 13.6 or 27.3 MHz. Today, most of the CO2 lasers are RF-excited because this type of discharge comes with a number of advantages. The capacitive coupling of energy through dielectric materials (glass, ceramics) allows the electrodes to be arranged outside the discharge space, whereas with DC discharges they are always in contact with the gas. They contaminate the CO2 gas through their burn-up (due to the high voltage drop) and therefore require an increased exchange with fresh gas. Further advantages of HF excitation are the significantly higher achievable power densities as well as a more homogeneous and more stable form of discharge compared to DC excitation. 6 Laser Technology Laser beam sources: CO2 laser Requirements for a Laser Laser active medium required for light amplification Inversion must be continuously maintained via pumping Part of the amplified light wave must be fed back into the active medium in the correct phase Laser beam Reflective mirror Coupling mirror Pump source Dr.-Ing Mahdi AMNE ELAHI, Prof. Dr.-Ing Peter PLAPPER In the laser medium, the radiation is stimulated by the supply of energy. A lamp or a diode or high-frequency voltage is usually used as the pump source. It pumps the required energy into the laser medium. The laser medium (or laser material) is either gas (e.g. CO2) or liquid or a solid (e.g. a doped YAG crystal). By introducing energy into the active medium, a population inversion is generated and maintained there (the pumping). By supplying energy to the pump source, the laser medium is stimulated to emit radiation. In the resonator, which is usually formed by two mirrors, this radiation is now reflected several times and thus passed through the active laser medium. Due to the multiple and long path between the mirrors, the parallel part of the light emerges from the laser as a parallel, coherent laser beam of one wavelength. 7 Laser Technology Laser beam sources: CO2 laser Typical stucture of a CO2 Laser Window (transparent glass) Electrodes Laser gas Resonator Glass tube Resonator Mirror Mirror Wavelength: 10.6 µm Continous high output power with very good beam quality Dr.-Ing Mahdi AMNE ELAHI, Prof. Dr.-Ing Peter PLAPPER Principle set up of a CO2 laser: The high voltage generates plasma in the laser gas, which emits photons. This light is fed back through the concave resonator mirrors. One mirror is opaque, the other has a low transparency, so that the laser light can exit on the right. The laser gas flows in at the top right and out at the bottom left 8 Laser beam sources: CO2 laser “internal” Laser beam mirror mirror CO2 Gas flow cavity “external” laserbeam cavity pump End mirror Quelle: Poprawe: Tailored Light 2, 2011, Springer Berlin Heidelberg Dr.-Ing Mahdi AMNE ELAHI, Prof. Dr.-Ing Peter PLAPPER Longitudinal flow CO2 lasers have good beam quality. Shown are here 4 cavities, which are connected via 2 copper mirrors. The black arrow show flow of CO2 gas. 9 Laser Technology Laser beam sources: CO2 laser Structure of a CO2 Laser Resonator 1x folded 4 discharge zones 2000 W beam power Hot CO2 Laser gas source: comp. Trumpf Dr.-Ing Mahdi AMNE ELAHI, Prof. Dr.-Ing Peter PLAPPER Seite 10 Laser Technology Laser beam sources: CO2 laser Axiale flow CO2 Laser with multiple folded resonator End-of-resonator Turbo Mirror ventilator Resonator- mirror Source: Trumpf Dr.-Ing Mahdi AMNE ELAHI, Prof. Dr.-Ing Peter PLAPPER Seite 11 Laser beam sources: CO2 laser Gas flow in CO2 Laser system Source: Steen, Mazumder: Laser Material Processing, 4th ed., 2010, Springer London Dr.-Ing Mahdi AMNE ELAHI, Prof. Dr.-Ing Peter PLAPPER Different types of CO2 lasers can be categorized in flowing and stationary gas mixtures. The electrical excitation happens by alternating or direct current. This is the principle of a longitudinal or an axial-flow CO2 laser. Diffusion-cooled multi-kW lasers with large electrodes (which also serve for cooling) are shown on next page. 12 Laser beam sources: CO2 laser (Slab laser) Slab laser: HF excited with large electrodes Quelle: Poprawe: Tailored Light 2, 2011, Springer Berlin Heidelberg Dr.-Ing Mahdi AMNE ELAHI, Prof. Dr.-Ing Peter PLAPPER Excitation in the upper laser level takes place via collision with an electron or, much more likely, via collision with a vibronically excited nitrogen molecule. Thus, nitrogen is added to the laser gas to enhance this pumping process. In addition helium is added for efficient cooling and depletion of the lower laser level via collisions. The pressure of the gas mixture between the laser mirrors is usually below normal conditions (100–250 hPa) in order to achieve a homogeneous discharge. Commercially available CO2 lasers achieve electro-optic efficiencies of 5–15%. Poprawe: Tailored Light 2, 2011, Springer Berlin Heidelberg 13 Laser beam sources: Solid state laser Nd:YAG rod laser Stimulation from flash lamps reflector Light: 1.06 µm Nd:YAG laser rod conduction via glasfiber flash lamp Laser beam mirror Outcoupling mirror cooling Pump pump lamp light Quelle: Trumpf & Poprawe: Tailored Light 2, 2011, Springer Berlin Heidelberg Dr.-Ing Mahdi AMNE ELAHI, Prof. Dr.-Ing Peter PLAPPER Solid-state lasers have a laser-active medium which consists of a host material with ions as dopants, mostly Nd or Yb. Within these ions the laser process of excitation and stimulated emission takes place. Depending on the geometry of the medium the most common host materials are fused silica (fiber geometry) or a crystal called yttrium aluminum garnet (YAG; disk/rod/slab geometry). Excitation of the ions is facilitated by absorption of light. The electronic transition from the upper to the lower laser level of an excited ion takes place under emission of light of 1,064 nm wavelength for neodymium and 1,030 nm for ytterbium ions. These emission wavelengths around 1 μ m can be transported by flexible glass fibers. Solid-state lasers can be distinguished in the way they are optically excited (lamps, diode lasers) and the geometry of the active medium (rod, disk, fiber, slab). They can be operated in continuous wave (cw) and pulsed mode. Pulsed operation can be achieved by gain switching, quality switching, or mode locking. Gain switching is facilitated by modulation of the pumping source. Technically this is mostly done with flash lamps with the laser pulse duration following the pump pulse duration which are typically in the range of 50–2000 μsec with repetition rates up to 4 kHz. Modulation of diode lasers is also common. Quality switching enables the generation of shorter laser pulses with typical pulse durations from 10 to 500 ns with repetition rates of 100 kHz. The quality of the optical resonator is reduced with an optical switch (mechanical shutter, acousto-optic modulator, electro-optic modulator) during pumping, i.e., the resonator is virtually blocked. Opening the resonator again releases the stored energy in a short light pulse. Mode locking occurs when the axial modes of a resonator are coupled in phase, i.e., they start oscillating at the same time. In this case they interfere in time to a very short pulse with pulse durations from several picoseconds down to several femtoseconds if the spectral bandwidth of the active medium supports enough axial modes. Technically, locking of the modes is realized by modulating the resonator losses with the resonator roundtrip time and caring for dispersion effects. Typical repetition rates of an oscillator are in the range of 100 MHz. Lamp-pumped systems usually have solid- state media in rod geometry. The rods have typical radii of 1–4 mm and lengths of 20–200 mm and are often situated in the focal line of an elliptical reflector. In the remaining focal line(s) is the lamp. Laser rod and lamp are imbedded in a flow tube and are cooled by circumfluent water. Poprawe: Tailored Light 2, 2011, Springer Berlin Heidelberg 14 Laser Technology Laser beam sources: Power balance in pumped solid state laser flash lamp pumped laser vs. diode pumped laser 100% electrical 100% electrical power of flash lamp power of flash lamp 50 % thermal looses 50 % thermal looses 50% Light 50% Light 30% thermal losses in pump cavity 10% thermal losses in pump cavity 20% 40% absorbed absorbed power power 15% thermal losses in laser 15% thermal losses in laser 5% beam power 25% beam power Dr.-Ing Mahdi AMNE ELAHI, Prof. Dr.-Ing Peter PLAPPER Comparing different means of excitation of solid-state lasers, the use of lamps has a number of disadvantages. The emission spectrum of the lamps is usually very broad, which is usually much wider than the absorption lines the laser-active material. As a consequence, only a small proportion of the available pump power is absorbed and used in the laser medium. In contrast, diode lasers have a much narrower emission spectrum, which can be specifically tailored to the strongest absorption lines of the laser medium. Nd: YAG lasers are excited with diodes at a wavelength of around 808 nm, these usually having a spectral bandwidth of less than 5 nm and thus being covered very well by the absorption band of the Nd:YAG. Typical data are shown here. Diode-pumped solid-state lasers have a significantly higher overall efficiency because of the more efficient yield of the pump radiation. The use of diode lasers leads to slightly higher investment costs compared to lamps. Because of the more favorable ratio of beam power and heat loss in the laser medium, diode-pumped lasers offer significantly better beam properties with the same output power, in the sense of a lower beam parameter product. 15 Laser beam sources: Diode pumped solid state laser Longitudinally pumped rod laser Transversally pumped rod laser Quelle: Poprawe: Tailored Light 2, 2011, Springer Berlin Heidelberg Dr.-Ing Mahdi AMNE ELAHI, Prof. Dr.-Ing Peter PLAPPER The optical axis of the resonator is perpendicular on the polished circular facets of the rod. This is called a transversal pumping scheme. Lamp-pumped cw lasers are commercially available with powers from 50 to 800 W per laser rod and beam propagation parameters M2 from 15 to 150. Power scaling is possible by a serial arrangement of several pumped laser rods and is available up to 4 kW with beam propagation parameter M 2 = 70. The flash lamps used for gain switching have typical average powers from 20 to 500 W with pulse peak powers from 5 to 20 kW. Gas discharge lamps have a broad spectral emission compared to the absorption spectrum of doped neodymium or ytterbium ions. This leads to a poor pumping efficiency. The emission wavelength of AlGaAs diode lasers can be adjusted to the absorption spectrum of the laser-active ion. This enhances pumping efficiency significantly and in addition offers the advantage that the intensity distribution of this pump source can be tailored to the geometry of the active medium. The practically achievable electro-optic efficiency is between 10 and 25% which is an order of magnitude higher compared to the several percent of lamp-pumped laser systems. Poprawe: Tailored Light 2, 2011, Springer Berlin Heidelberg 16 Laser beam sources: Types of solid-state lasers Rod laser Disc laser Fiber laser Diode laser (Nd: YAG) Laser Emission Cooling along the entire sleeve Pump light front T T r r Pump light cooling -+ Transfer of cooling and pumping Cooling from the rear Even cooling surface Direct conversion of energy along the sleeve => Planar temperature profile => Planar temperature profile current to light => Limited beam quality High beam quality => High beam quality Dr.-Ing Mahdi AMNE ELAHI, Prof. Dr.-