Laboratory Instrumentation and Techniques PDF
Document Details
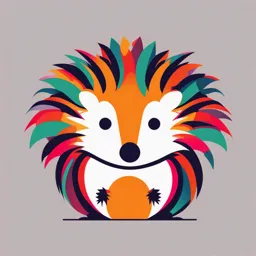
Uploaded by MajesticPlatinum3151
Achievers University
Dr. Mathew Folaranmi OLANIYAN
Tags
Related
- MLS1004SEF Clinical Chemistry & Immunonology Lecture 1 PDF
- MLS 1004SEF Clinical Chemistry & Immunology Lecture 2 2024 PDF
- Clinical Chemistry 1 Instrumentation OL FU PDF
- Instrumentation In Medical Diagnostic, Laboratory And Blood Banking BIO62004 PDF
- Instrumentation Prelim Week 2 PDF
- General Introduction and Ordinary Microscope PDF
Summary
This book details laboratory instrumentation and techniques, covering various instruments like autoclaves and centrifuges. It's aimed at undergraduate and postgraduate students in medical laboratory science and related fields.
Full Transcript
LABORATORY INSTRUMENTATION AND TECHNIQUES Dr. Mathew Folaranmi OLANIYAN Associate Professor Department of Medical Laboratory Science Achievers University, Owo-Nigeria i DEDICATION This book is dedicated to Almig...
LABORATORY INSTRUMENTATION AND TECHNIQUES Dr. Mathew Folaranmi OLANIYAN Associate Professor Department of Medical Laboratory Science Achievers University, Owo-Nigeria i DEDICATION This book is dedicated to Almighty God and my children(Olamide, Ajibola and Oluwatobi) ii PREFACE This book is written out of the author’s several years of professional and academic experience in Medical Laboratory Science. The textbook is well-planned to extensively cover the working principle and uses of laboratory instruments. Common Laboratory techniques (including principle and applications) are also discussed. Descriptive diagrams/schematics for better understanding are included. Teachers and students pursuing courses in different areas of Laboratory Science, Basic and medical/health sciences at undergraduate and postgraduate levels will find the book useful. Researchers and interested readers will also find the book educative and interesting. iii TABLE OF CONTENTS TITLE PAGE TITLE PAGE………………………………………………………………………………… i DEDICATION………………………………………………………………………………. ii PREFACE…………………………………………………………………………………… iii TABLE OF CONTENT…………………………………………………………………….. iv CHAPTER ONE BASIC CONCEPTS……………………………………………………………………….. 1 CHAPTER TWO AUTOCLAVE……………………………………………………………………………….. 3 CHAPTER THREE CENTRIFUGES……………………………………………………………………………. 10 CHAPTER FOUR WEIGHING BALANCE…………………………………………………………………... 14 CHAPTER FIVE LABORATORY WATERBATHS……………………………………………………… 20 CHAPTER SIX ANAEROBIC JARS………………………………………………………………………… 23 CHAPTER SEVEN MICROSCOPE……………………………………………………………………………… 28 CHAPTER EIGHT SPECTROPHOTOMETER AND COLORIMETER………………………………. 39 CHAPTER NINE FLAME PHOTOMETERS………………………………………………………………... 53 iv CHAPTER TEN ION SELECTIVE ELECTRODES AND POTENTIOMETRY…………………. 58 CHAPTER ELEVEN HOT AIR/BOX OVEN…………………………………………………………………… 79 CHAPTER TWELVE ELIZA READER…………………………………………………………………………… 83 CHAPTER THIRTEEN REFRIGERATOR…………………………………………………………………………… 88 CHAPTER FOURTEEN LABORATORY MIXER…………………………………………………….…………… 100 CHAPTER FIFTEEN POLYMERASE CHAIN REACTION (PCR ) MACHINE………………..………. 101 CHAPTER SIXTEEN LABORATORY INCUBATOR…………………………………………………………. 105 CHAPTER SEVENTEEN MICROTOMES…………………………………………………………………………. ….. 112 CHAPTER EIGHTEEN ENZYME-LINKED IMMUNOSORBENT ASSAYS (ELISAs) TECHNIQUE. 120 CHAPTER NINETEEN MICROSCOPY TECHNIQUE………………………………………………………….. 123 CHAPTER TWENTY HISTOLOGICAL TECHNIQUES – MICROTOMY………………………………. 138 CHAPTER TWENTY ONE SPECTROPHOTOMETRY AND COLORIMETRY………………………………. 164 CHAPTER TWENTY TWO ELECTROPHORESIS……………………………………………………………………. 174 CHAPTER TWENTY THREE POLYMERASE CHAIN REACTION (PCR)………………………………………. 182 CHAPTER TWENTY FOUR FLOROMETRY /SPECTROFLOROMETRY……………………………………… 187 CHAPTER TWENTY FIVE LYOPHILISATION (FREEZE-DRYING)……………………………………….. … 201 CHAPTER TWENTY SIX OSMOMETRY……………………………………………………………………………… 205 CHAPTER TWENTY SEVEN TURBIDIMETRY AND NEPHELOMETRY…………………………………………. 208 CHAPTER TWENTY EIGHT CONDUCTOMETRY, POLAROGRAPHY AND POLAROGRAPHY…………. 210 CHAPTER TWENTY NINE RADIOIMMUNOASSAY (RIA)………………………………………………………………. 215 CHAPTER THIRTY AUTOANALYZERS/ AUTOMATED ANALYSER………………………………. 218 CHAPTER THIRTY ONE SOLVENT EXTRACTION…………………………………………………………………….. 225 CHAPTER THIRTY TWO CHROMATOGRAPHY………………………………………………………………………….. 228 CHAPTER THIRTY THREE FLOW CYTOMETRY……………………………………………………………………………. 240 LIST OF REFERENCES…………………………………………………………………………. 244 iv v CHAPTER ONE BASIC CONCEPTS Laboratory instrumentation is the use or application of instruments for observation, measurement, or control. It involves the use of or operation with instruments; especially: the use of one or more instruments in carrying out laboratory tests. Instrumentation is the development or use of measuring instruments for observation, monitoring or control. The use of UV spectrophotometry (to measure light intensity) and gas chromatography. Laboratory instrumentation is a collection of laboratory test equipment. Such a collection of equipment might be used to automate testing procedure. It could also include: "The design, construction, and provision of instruments for measurement, control, etc; the state of being equipped with or controlled by such instruments collectively." Laboratory Instrument is any implement, tool, or utensil used for laboratory test. An instrument is a device that measures a physical quantity, such as flow, concentration, temperature, level, distance, angle, or pressure. Instruments may be as simple as direct reading hand-held thermometers or as complex as multi-variable process analyzers. Medical instrument is a device used to diagnose or treat diseases. A tool or device used for a particular purpose; especially: a tool or device designed to do careful and exact work. A device that measures something. Laboratory equipment, the measuring tools used in a scientific laboratory, often electronic in nature. Laboratory equipment refers to the various tools and equipment used by scientists working in a laboratory. Laboratory equipment is generally used to either perform an experiment or to take measurements and gather data. Larger or more sophisticated equipment is generally called a scientific instrument. Both laboratory equipment and scientific instruments are increasingly being designed and shared using open hardware principles.The classical equipment includes tools such as Bunsen burners and microscopes as well as specialty equipment such as operant conditioning chambers, spectrophotometers and calorimeters. Laboratory techniques are the sum of procedures used on pure and applied sciences in order to conduct an experiment, all of them follow scientific method; while some of them involves the use of complex laboratory equipment from laboratory glassware to electrical devices others require such specific or expensive supplies. 1 Laboratory apparatus is a set of equipment or tools or a machine that is used for a particular purpose. Laboratory apparatus is the individual instruments or pieces of equipment, or the entire set of equipment to conduct projects and experiments. The most common utensils and appliances that you need while performing hands on activities in a laboratory. The laboratory apparatus depends upon the type of laboratory you are in and the experiment you are going to perform. Laboratory tool is any physical item that can be used to achieve a goal, especially if the item is not consumed in the process. Tools that are used in particular fields or activities may have different designations such as "instrument", "utensil", "implement", "machine", "device," or "apparatus". The set of tools needed to achieve a goal is "equipment". The knowledge of constructing, obtaining and using tools is technology. 2 CHAPTER TWO AUTOCLAVE An autoclave is essentially just a large steel vessel through which steam or another gas is circulated to sterilize things, perform scientific experiments, or carry out industrial processes. Typically the chambers in autoclaves are cylindrical, because cylinders are better able to withstand extreme pressures than boxes, whose edges become points of weakness that can break. The high-pressure makes them self-sealing (the words "auto" and "clave" mean automatic locking), though for safety reasons most are also sealed manually from outside. Just like on a pressure cooker, a safety valve ensures that the steam pressure cannot build up to a dangerous level. A medical autoclave is a device that uses steam to sterilize equipment and other objects. This means that all bacteria, viruses, fungi, and spores are inactivated. However, prions, such as those associated with Creutzfeldt- Jakob disease, may not be destroyed by autoclaving at the typical 134 °C for three minutes or 121 °C for 15 minutes[citation needed]. Although that a wide range species of archaea, including Geogemma barosii, can survive at temperatures above 121 °C, no archaea are known to be infectious or pose a health risk to humans; in fact their biochemistry is so vastly different from our own and their multiplication rate is far too slow for microbiologists to worry about them. Autoclaves are found in many medical settings, laboratories, and other places that need to ensure the sterility of an object. Many procedures today employ single-use items rather than sterilizable, reusable items. This first happened with hypodermic needles, but today many surgical instruments (such as forceps, needle holders, and scalpel handles) are commonly single- use rather than reusable items (see waste autoclave). Autoclaves are of particular importance in poorer countries due to the much greater amount of equipment that is re-used. Providing stove-top or solar autoclaves to rural medical centres has been the subject of several proposed medical aid missions. 3 Because damp heat is used, heat-labile products (such as some plastics) cannot be sterilized this way or they will melt. Paper and other products that may be damaged by steam must also be sterilized another way. In all autoclaves, items should always be separated to allow the steam to penetrate the load evenly. Autoclaving is often used to sterilize medical waste prior to disposal in the standard municipal solid waste stream. This application has become more common as an alternative to incineration due to environmental and health concerns raised because of the combustion by-products emitted by incinerators, especially from the small units which were commonly operated at individual hospitals. Incineration or a similar thermal oxidation process is still generally mandated for pathological waste and other very toxic and/or infectious medical waste. In dentistry, autoclaves provide sterilization of dental instruments according to health technical memorandum 01-05 (HTM01-05). According to HTM01-05, instruments can be kept, once sterilized using a vacuum autoclave for up to 12 months using sealed pouches Working Principle Why is an autoclave such an effective sterilizer? An autoclave is a large pressure cooker; it operates by using steam under pressure as the sterilizing agent. High pressures enable steam to reach high temperatures, thus increasing its heat content and killing power. Most of the heating power of steam comes from its latent heat of vaporization. This is the amount of heat required to convert boiling water to steam. This amount of heat is large compared to that required to make water hot. For example, it takes 80 calories to make 1 liter of water boil, but 540 calories to convert that boiling water to steam. Therefore, steam at 100… C has almost seven times more heat than boiling water. Steam is able to penetrate objects with cooler temperatures because once the steam contacts a cooler surface, it immediately condenses to water, producing a concomitant 1,870 fold decrease in steam volume. This creates negative pressure at the point of condensation and draws more steam to the area. Condensations continue so long as the temperature of the condensing surface is less than that of steam; once temperatures equilibrate, a saturated steam environment is formed. 4 Achieving high and even moisture content in the steam-air environment is important for effective autoclaving. The ability of air to carry heat is directly related to the amount of moisture present in the air. The more moisture present, the more heat can be carried, so steam is one of the most effective carriers of heat. Steam therefore also results in the efficient killing of cells, and the coagulation of proteins. When you cook beef at home, for example, it can become tough when roasted in a covered pan in the oven. But just add a little water in the bottom of the pan, and you will find that the meat will be tender! The temperature is the same and the time of roasting is the same, but the result is different. Now (as in an autoclave) add another parameter, pressure. By putting this same roast in a pressure cooker you can reduce the time it takes to cook this roast by at least three quarters, and you still get just as tender a finished product. How does killing occur? Moist heat is thought to kill microorganisms by causing coagulation of essential proteins. Another way to explain this is that when heat is used as a sterilizing agent, the vibratory motion of every molecule of a microorganism is increased to levels that induce the cleavage of intramolecular hydrogen bonds between proteins. Death is therefore caused by an accumulation of irreversible damage to all metabolic functions of the organism. Death rate is directly proportional to the concentration of microorganisms at any given time. The time required to kill a known population of microorganisms in a specific suspension at a particular temperature is referred to as thermal death time (TDT). All autoclaves operate on a time/temperature relationship; increasing the temperature decreases TDT, and lowering the temperature increases TDT. What is the standard temperature and pressure of an autoclave? Processes conducted at high temperatures for short time periods are preferred over lower temperatures for longer times. Some standard temperatures/pressures employed are 115 ¡C/10 p.s.i., 121¡C/ 15 p.s.i., and 132 ¡C/27 p.s.i. (psi=pounds per square inch). In our university autoclave, autoclaving generally involves heating in saturated steam under a pressure of approximately 15 psi, to achieve a chamber temperature of a least 121¡C (250¡F)but in other applications in industry, for example, other combinations of time and temperature are sometimes used. 5 Please note that after loading and starting the autoclave, the processing time is measured after the autoclave reaches normal operating conditions of 121¡C (250¡F) and 15 psi pressure, NOT simply from the time you push the "on" button. How does the autoclave itself work? Basically, steam enters the chamber jacket, passes through an operating valve and enters the rear of the chamber behind a baffle plate. It flows forward and down through the chamber and the load, exiting at the front bottom. A pressure regulator maintains jacket and chamber pressure at a minimum of 15 psi, the pressure required for steam to reach 121…C (250…F). Overpressure protection is provided by a safety valve. The conditions inside are thermostatically controlled so that heat (more steam) is applied until 121C is achieved, at which time the timer starts, and the temperature is maintained for the selected time. Mode of operation Artwork: How an autoclave works (simplified): (1) Steam flows in through a pipe at the bottom and around a closed jacket that surrounds the main chamber (2), before entering the chamber itself (3). The steam sterilizes whatever has been placed inside (in this case, three blue drums) (4) before exiting through an exhaust pipe at the bottom (5). A tight door lock and gasket seal (6) keeps the steam securely inside. A safety valve (7) similar to the ones on a pressure cooker will pop out if the pressure gets too high. Once the chamber is sealed, all the air is removed from it either by a simple vacuum pump (in a design called pre-vacuum) or by pumping in steam to force the air out of the way (an alternative design called gravity displacement). 6 Next, steam is pumped through the chamber at a higher pressure than normal atmospheric pressure so it reaches a temperature of about 121– 140°C (250–284°F). Once the required temperature is reached, a thermostat kicks in and starts a timer. The steam pumping continues for a minimum of about 3 minutes and a maximum of about 15–20 minutes (higher temperatures mean shorter times)—generally long enough to kill most microorganisms. The exact sterilizing time depends on a variety of factors, including the likely contamination level of the items being autoclaved (dirty items known to be contaminated will take longer to sterilize because they contain more microbes) and how the autoclave is loaded up (if steam can circulate more freely, autoclaving will be quicker and more effective). Autoclaving is a bit like cooking, but as well as keeping an eye on the temperature and the time, the pressure matters too! Safety is all-important. Since you're using high-pressure, high-temperature steam, you have to be especially careful when you open an autoclave that there is no sudden release of pressure that could cause a dangerous steam explosion. To be effective against spore forming bacteria and viruses, autoclaves need to: ◾Have steam in direct contact with the material being sterilized (i.e. loading of items is very important). ◾Create vacuum in order to displace all the air initially present in the autoclave and replacing it with steam. ◾Implement a well designed control scheme for steam evacuation and cooling so that the load does not perish. The efficiency of the sterilization process depends on two major factors. One of them is the thermal death time, i.e. the time microbes must be exposed to at a particular temperature before they are all dead. The second factor is the thermal death point or temperature at which all microbes in a sample are killed.The steam and pressure ensure sufficient heat is transferred into the organism to kill them. A series of negative pressure pulses are used to vacuum all possible air pockets, while steam penetration is maximized by application of a succession of positive pulses 7 Test for the efficacy of an Autoclave(quality assurance) There are physical, chemical, and biological indicators that can be used to ensure that an autoclave reaches the correct temperature for the correct amount of time. If a non-treated or improperly treated item can be confused for a treated item, then there is the risk that they will become mixed up, which, in some areas such as surgery, is critical. Chemical indicators on medical packaging and autoclave tape change color once the correct conditions have been met, indicating that the object inside the package, or under the tape, has been appropriately processed. Autoclave tape is only a marker that steam and heat have activated the dye. The marker on the tape does not indicate complete sterility. A more difficult challenge device, named the Bowie-Dick device after its inventors, is also used to verify a full cycle. This contains a full sheet of chemical indicator placed in the center of a stack of paper. It is designed specifically to prove that the process achieved full temperature and time required for a normal minimum cycle of 274 degrees F for 3.5–4 minutes To prove sterility, biological indicators are used. Biological indicators contain spores of a heat-resistant bacterium, Geobacillus stearothermophilus. If the autoclave does not reach the right temperature, the spores will germinate when incubated and their metabolism will change the color of a pH-sensitive chemical. Some physical indicators consist of an alloy designed to melt only after being subjected to a given temperature for the relevant holding time. If the alloy melts, the change will be visible Some computer-controlled autoclaves use an F0 (F-nought) value to control the sterilization cycle. F0 values are set for the number of minutes of sterilization equivalent to 121 °C (250 °F) at 100 kPa (15 psi) above atmospheric pressure for 15 minutes. Since exact temperature control is difficult, the temperature is monitored, and the sterilization time adjusted accordingly. Application of autoclave Sterilization autoclaves are widely used in microbiology, medicine, podiatry, tattooing, body piercing, veterinary science, mycology, funeral homes, dentistry, and prosthetics fabrication. They vary in size and function depending on the media to be sterilized. 8 Typical loads include laboratory glassware, other equipment and waste, surgical instruments, and medical waste.A notable recent and increasingly popular application of autoclaves is the pre-disposal treatment and sterilization of waste material, such as pathogenic hospital waste. Machines in this category largely operate under the same principles as conventional autoclaves in that they are able to neutralize potentially infectious agents by utilizing pressurized steam and superheated water. A new generation of waste converters is capable of achieving the same effect without a pressure vessel to sterilize culture media, rubber material, gowns, dressing, gloves, etc. It is particularly useful for materials which cannot withstand the higher temperature of a hot air oven. Autoclaves are also widely used to cure composites and in the vulcanization of rubber. The high heat and pressure that autoclaves allow help to ensure that the best possible physical properties are repeatably attainable. The aerospace industry and sparmakers (for sailboats in particular) have autoclaves well over 50 feet (15 m) long, some over 10 feet (3.0 m) wide. Other types of autoclave are used to grow crystals under high temperatures and pressures. Synthetic quartz crystals used in the electronic industry are grown in autoclaves. Packing of parachutes for specialist applications may be performed under vacuum in an autoclave which allows the parachute to be warmed and inserted into the minimum volume. 9 CHAPTER THREE CENTRIFUGES A laboratory centrifuge is a piece of laboratory equipment, driven by a motor, which spins liquid samples at high speed. There are various types of centrifuges, depending on the size and the sample capacity. Like all other centrifuges, laboratory centrifuges work by the sedimentation principle, where the centripetal acceleration is used to separate substances of greater and lesser density. A centrifuge is a device for separating two or more substances from each other by using centrifugal force. Centrifugal force is the tendency of an object traveling around a central point to continue in a linear motion and fly away from that central point. Centrifugation can be used to separate substances from each other because materials with different masses experience different centrifugal forces when traveling at the same velocity and at the same distance from the common center. For example, if two balls of different mass are attached to strings and swung around a common point at the same velocity, the ball with the greater mass will experience a greater centrifugal force. If the two strings are cut simultaneously, the heavier ball will tend to fly farther from the common center HBJthan will the lighter ball. Centrifuges can be considered devices for increasing the effects of the earth's gravitational pull. For example, if a spoonful of clay is mixed vigorously with a cup of water and then allowed to sit for a period of time, the clay will eventually settle out because it experiences a greater gravitational pull than does the water. If the same clay-water mixture is centrifuged, however, the separation will take place much more quickly. There are different types of laboratory centrifuges: Microcentrifuges (devices for small tubes from 0.2 ml to 2.0 ml (micro tubes), up to 96 well-plates, compact design, small footprint; up to 30,000 g) Clinical centrifuges (moderate-speed devices used for clinical applications like blood collection tubes) 10 Multipurpose high-speed centrifuges (devices for a broad range of tube sizes, high variability, big footprint) Ultracentrifuges (analytical and preparative models) Because of the heat generated by air friction (even in ultracentrifuges, where the rotor operates in a good vacuum), and the frequent necessity of maintaining samples at a given temperature, many types of laboratory centrifuges are refrigerated and temperature regulated. Centrifugation A centrifuge is used to separate particles or macromolecules: -Cells -Sub- cellular components -Proteins -Nucleic acids Basis of separation: -Size - Shape -Density Methodology: -Utilizes density difference between the particles/macromolecules and the medium in which these are dispersed - Dispersed systems are subjected to artificially induced gravitational fields Principles of centrifugation A centrifuge is a device for separating particles from a solution according to their size, shape, density, viscosity of the medium and rotor speed. In a solution, particles whose density is higher than that of the solvent sink (sediment),and particles that are lighter than it float to the top. The greater the difference in density, the faster they move. If there is no difference in density (isopyknic conditions), the particles stay steady. To take advantage of even tiny differences in density to separate various particles in a solution, gravity can be replaced with the much more powerful “centrifugal force” provided by a centrifuge. Centrifuge Rotors Fixed Angle Rotor :Sedimenting particles have only short distance to travel before pelleting. Shorter run time. The most widely used rotor type. Swinging Bucket Rotor : Longer distance of travel may allow better separation, such as in density gradient centrifugation. Easier to withdraw supernatant without disturbing pellet. 11 Care and Maintenance of centrifuges Mechanical stress Always ensure that loads are evenly balanced before a run. „Always observe the manufacturers maximum speed and sample density ratings. „Always observe speed reductions when running high density solutions, plastic adapters, or stainless steel tubes. Many rotors are made from titanium or aluminum alloy, chosen for their advantageous mechanical properties. While titanium alloys are quite corrosion-resistant, aluminum alloys are not. When corrosion occurs, the metal is weakened and less able to bear the stress from the centrifugal force exerted during operation. The combination of stress and corrosion causes the rotor to fail more quickly and at lower stress levels than an uncorroded rotor. 12 1- A tabletop micro laboratory centrifuge 2- Laboratory macro/bench centrifuge 4- An Eppendorf laboratory centrifuge 13 CHAPTER FOUR WEIGHING BALANCE Balances are designed to meet the specific weighing requirement in the laboratory working environment. These balances come in precision designs and operating characteristics that allows making quick and accurate measurements. Further, these balances can also be tubes to transfer data to computer for further analysis as well as can have piece count functions and hopper functions. With end usage of these scales in precision weighing applications in laboratories, these also offer excellent value of money invested. Here, our expertise also lies in making these available in both standard and custom tuned specifications. The range offered includes Analytical Balances, General Purpose Electronic Balance, Laboratory Balances and Precision Weighing Balances. The history of balances and scales dates back to Ancient Egypt. A simplistic equal-arm balance on a fulcrum that compared two masses was the standard. Today, scales are much more complicated and have a multitude of uses. Applications range from laboratory weighing of chemicals to weighing of packages for shipping purposes. To fully understand how balances and scales operate, there must be an understanding of the difference between mass and weight. Mass is a constant unit of the amount of matter an object possesses. It stays the same no matter where the measurement is taken. The most common units for mass are the kilogram and gram. Weight is the heaviness of an item. It is dependent on the gravity on the item multiplied by the mass, which is constant. The weight of an object on the top of a mountain will be less than the weight of the same object at the bottom due to gravity variations. A unit of measurement for weight is the newton. A newton takes into account the mass of an object and the relative gravity and gives the total force, which is weight. Although mass and weight are two different entities, the process of determining both weight and mass is called weighing. 14 Balance and Scale Terms Accuracy The ability of a scale to provide a result that is as close as possible to the actual value. The best modern balances have an accuracy of better than one part in 100 million when one-kilogram masses are compared. Calibration The comparison between the output of a scale or balance against a standard value. Usually done with a standard known weight and adjusted so the instrument gives a reading in agreement. Capacity The heaviest load that can be measured on the instrument. Precision Amount of agreement between repeated measurements of the same quantity; also known as repeatability. Note: A scale can be extremely precise but not necessarily be accurate. Readability This is the smallest division at which the scale can be read. It can vary as much as 0.1g to 0.0000001g. Readability designates the number of places after the decimal point that the scale can be read. Tare The act of removing a known weight of an object, usually the weighing container, to zero a scale. This means that the final reading will be of the material to be weighed and will not reflect the weight of the container. Most balances allow taring to 100% of capacity. Balance and Scale Types Analytical Balance These are most often found in a laboratory or places where extreme sensitivity is needed for the weighing of items. Analytical balances measure mass. Chemical analysis is always based upon mass so the results are not based on gravity at a specific location, which would affect the weight. Generally capacity for an analytical balance ranges from 1 g to a few kilograms with precision and accuracy often exceeding one part in 106 at full capacity. There are several important parts to an analytical balance. A beam arrest is a mechanical device that prevents damage to the delicate internal devices when objects are being placed or removed from the pan. The pan is the area on a balance where an object is placed to be weighed. Leveling feet are adjustable legs that allow the balance to be brought to the reference position. The reference position is determined by the spirit level, leveling bubble, or plumb bob that is an integral part of the balance. Analytical balances are so sensitive that even air currents can affect the measurement. 15 To protect against this they must be covered by a draft shield. This is a plastic or glass enclosure with doors that allows access to the pan. Analytical Balances come with highest accuracy for meeting the demands of analytical weighing processes. These balances come equipped with provision for eliminating interfering ambient effects as well as in delivering repeatable weighing results with a fast response. These are recommended for use in analytical applications requiring precision performance and durability. Here, the presence of latest automatic internal calibration mechanism also helps in keeping balance calibrated at all times, thus providing for optimum weighing accuracy. These scales and balances also automatically calibrate itself at startup, at preset time intervals or whenever as required by temperature changes. Equal Arm Balance/Trip Balance This is the modern version of the ancient Egyptian scales. This scale incorporates two pans on opposite sides of a lever. It can be used in two different ways. The object to be weighed can be placed on one side and standard weights are added to the other pan until the pans are balanced. The sum of the standard weights equals the mass of the object. Another application for the scale is to place two items on each scale and adjust one side until both pans are leveled. This is convenient in applications such as balancing tubes or centrifugation where two objects must be the exact same weight. Platform Scale This type of scale uses a system of multiplying levers. It allows a heavy object to be placed on a load bearing platform. The weight is then transmitted to a beam that can be balanced by moving a counterpoise, which is an element of the scale that counterbalances the weight on the platform. This form of scale is used for applications such as the weighing of drums or even the weighing of animals in a veterinary office. Spring Balance This balance utilizes Hooke's Law which states that the stress in the spring is proportional to the strain. Spring balances consist of a highly elastic helical spring of hard steel suspended from a fixed point. The weighing pan is attached at the lowest point of the spring. An indicator shows the weight measurement and no manual adjustment of weights is necessary. An example of this type of balance would be the scale used in a grocery store to weigh produce. Top-Loading Balance This is another balance used primarily in a laboratory setting. They usually can measure objects weighing around 150– 5000 g. They offer less readability than an analytical balance, but allow 16 measurements to be made quickly thus making it a more convenient choice when exact measurements are not needed. Top-loaders are also more economical than analytical balances. Modern top-loading balances are electric and give a digital readout in seconds. Torsion Balance Measurements are based on the amount of twisting of a wire or fiber. Many microbalances and ultra-microbalances, that weigh fractional gram values, are torsion balances. A common fiber type is quartz crystal. Triple-Beam Balance This type of balance is less sensitive than a top- loading balance. They are often used in a classroom situation because of ease of use, durability and cost. They are called triple-beam balances because they have three decades of weights that slide along individually calibrated scales. The three decades are usually in graduations of 100g, 10g and 1g. These scales offer much less readability but are adequate for many weighing applications. Precision Weighing Balances are laboratory standard high precision balances that are based on latest process technology and features best displayed increment of 0.001g (1mg) with maximum capacity available. These perfectly match up the applications demanding more than a standard balance and assist in simplifying complex laboratory measurements including in determining difference between initial & residual weights. Here, the calculation of the density of solids & liquids also eliminates need for time consuming manual calculation and data logging. The standard features include protective in-use cover and security bracket, working capacities from 0.1 mg to 230 gm, pan size of 90 mm, ACC of 0.1 mg, internal calibration, display using LCD with back light, standard RS-232 C interface and hanger for below balance weighing. Balance and Scale Care and Use A balance has special use and care procedures just like other measuring equipment. Items to be measured should be at room temperature before weighing. A hot item will give a reading less than the actual weight due to convection currents that make the item more buoyant. And, if your balance is enclosed, warm air in the case weighs less than air of the same volume at room temperature. 17 Another important part of using a balance is cleaning. Scales are exposed to many chemicals that can react with the metal in the pan and corrode the surface. This will affect the accuracy of the scale. Also, keep in mind that a potentially dangerous situation could occur if a dusting of chemicals is left on the balance pan. In many lab and classroom situations, more than one person uses a single scale for weighing. It would be impossible for each person to know what everyone else has been weighing. There is a chance that incompatible chemicals could be brought into contact if left standing or that someone could be exposed to a dangerous chemical that has not been cleaned from the balance. To avoid damaging the scale or putting others in danger, the balance should be kept extremely clean. A camel's hair brush can be used to remove any dust that can spill over during weighing. Calibration is another care issue when it comes to scales. A scale cannot be accurate indefinitely; they must be rechecked for accuracy. There are weight sets available that allow users to calibrate the scale themselves or the scales can be calibrated by hiring a professional to calibrate them on site. The correct weight set needs to be chosen when calibrating a scale. The classes of weight sets start from a Class One which provides the greatest precision, then to Class Two, Three, Four and F and finally go down to a Class M, which is for weights of average precision. Weight sets have class tolerance factors, and as a general rule, the tolerance factor should be greater than the readability of the scale. A scale should be calibrated at least once a year or per manufacturer’s guidelines. It can be done using calibration weight sets or can be calibrated by a professional. The readability of the scale will determine which weight set will be appropriate for calibrating the scale 18 What is the difference between accuracy and precision? Accuracy tells how close a scale gets to the real value. An inaccurate scale is giving a reading not close to the real value. Precision and accuracy are unrelated terms. A precise scale will give the same reading multiple times after weighing the same item. A precise scale can be inaccurate by repeatedly giving values that are far away from the actual value. For instance a scale that reads 5.2g three times in a row for the same item is very precise but if the item actually weighs 6.0g the scale is not accurate. 19 CHAPTER FIVE LABORATORY WATERBATHS Most general laboratory water baths go from ambient up to 80oC or 99oC. Boiling baths will boil water at 100oC (under normal conditions). Any baths that work above 100oC will need a liquid in them such as oil. Any baths that work below ambient will need an internal or external cooling system. If they work below the freezing point of water they will need to be filled with a suitable anti-freeze solution. If you require very accurate control you require the laboratory stirred baths with accurate thermostat / circulator. Water baths are used in industrial clinical laboratories, academic facilities, government research laboratories environmental applications as well as food technology and wastewater plants. Because water retains heat so well, using water baths was one of the very first means of incubation. Applications include sample thawing, bacteriological examinations, warming reagents, coliform determinations and microbiological assays. Different types of water baths may be required depending on the application. Below is a list of the different types of commerially available water baths and their general specifications. Types of Laboratory Water Baths: Unstirred water baths are the cheapest laboratory baths and have the least accurate temperature control because the water is only circulated by convection and so is not uniformly heated. Stirred water baths have more accurate temperature control. They can either have an in-built pump/circulator or a removable immersion thermostat / circulator (some of which can pump the bath liquid externally into an instrument and back into the bath). Circulating Water Baths Circulating water baths (also called stirrers ) are ideal for applications when temperature uniformity and consistency are critical, such as enzymatic and serologic experiments. Water is thoroughly circulated throughout the bath resulting in a more uniform temperature. 20 Non-Circulating Water Baths This type of water bath relies primarily on convection instead of water being uniformly heated. Therefore, it is less accurate in terms of temperature control. In addition, there are add-ons that provide stirring to non-circulating water baths to create more uniform heat transfer Shaking water baths have a speed controlled shaking platform tray (usually reciprocal motion i.e. back and forwards, although orbital motion is available with some brands) to which adaptors can be added to hold different vessels. Cooled water baths are available as either an integrated system with the cooling system (compressor, condenser, etc.) built into the laboratory water baths or using a standard water bath as above using an immersion thermostat / circulator with a separate cooling system such as an immersion coil or liquid circulated from a circulating cooler. The immersion thermostat used must be capable of controlling at the below ambient temperature you require Laboratory water baths working below 4oC should be filled with a liquid which does not freeze. Immersion thermostats which state they control bath temperatures below room ambient temperature need a cooling system as mentioned above to reach these temperatures. The immersion thermostat will then heat when required to maintain the set below ambient temperature. Boiling water baths are usually designed with an anlogue control to control water temperature from simmering up to boiling and have a water level device so that the bath does not run dry and an over temperature cut-out thermostat fitted to or near the heating element. The flat lids usually have a number of holes (up to 150mm. diameter for instance) with eccentric rings which can be removed to accommodate different size flasks. Specify the number of holes you require (usually a choice of 4, 6 or 12). Cooling circulators vary in size, cooling capacity / heat removal, temperature accuracy, flow rate, etc. and are used to cool laboratory water baths or remove heat from another piece of equipment by circulating the cooled water / liquid through it and back to the circulator 21 Construction and Dimensions: Laboratory water baths usually have stainless steel interiors and either chemically resistant plastic or epoxy coated steel exteriors. Controllers are either analogue or digital. Bath dimensions can be a bit misleading when litre capacity is quoted because it depends how high you measure (water baths are never filled to the top). To compare different bath volumes it is best to compare the internal tank dimensions. Laboratory Water Bath Accessories: Lift-off or hinged plastic (depending on bath temperature) or stainless steel lids are available as well as different racks to hold tubes, etc. Lids with holes with concentric rings are available for boiling water baths to hold different size flasks. Care and maintenance It is not recommended to use water bath with moisture sensitive or pyrophoric reactions. Do not heat a bath fluid above its flash point. Water level should be regularly monitored, and filled with distilled water only. This is required to prevent salts from depositing on the heater. Disinfectants can be added to prevent growth of organisms. Raise the temperature to 90 °C or higher to once a week for half an hour for the purpose of decontamination. Markers tend to come off easily in water baths. Use water resistant ones. If application involves liquids that give off fumes, it is recommended to operate water bath in fume hood or in a well-ventilated area. The cover is closed to prevent evaporation and to help reaching high temperatures. Set up on a steady surface away from flammable materials. 22 CHAPTER SIX ANAEROBIC JARS 23 Method of use 1a.The culture: The culture media are placed inside the jar, stacked up one on the other, and 24 1b..Indicator system: Pseudomonas aeruginosa, inoculated on to a nutrient agar plate is kept inside the jar along with the other plates. This bacteria need oxygen to grow (aerobic). A growth free culture plate at the end of the process indicates a successful anaerobiosis. However, P. aeruginosa possesses a denitrification pathway. If nitrate is present in the media, P. aeruginosa may still grow under anaerobic conditions. 2. 6/7ths of the air inside is pumped out and replaced with either unmixed Hydrogen or as a 10%CO2+90%H2 mixture. The catalyst (Palladium) acts and the oxygen is used up in forming water with the hydrogen. The manometer registers this as a fall in the internal pressure of the jar. 3. Hydrogen is pumped in to fill up the jar so that the pressure inside equals atmospheric pressure. The jar is now incubated at desired temperature settings. DESCRIPTION The jar (McIntosh and Filde's anaerobic jar), about 20″×12.5″ is made up of a metal. Its parts are as follows: 1.The body made up of metal (airtight) 2.The lid, also metal can be placed in an airtight fashion 3.A screw going through a curved metal strip to secure and hold the lid in place 4.A thermometer to measuring the internal temperature 5.A pressure gauge to measuring the internal pressure (or a side tube is attached to a manometer) 6.Another side tube for evacuation and introduction of gases (to a gas cylinder or a vacuum pump) 7.A wire cage hanging from the lid to hold a catalyst that makes hydrogen react to oxygen without the need of any ignition source 25 Gas-pak Gas-pak is a method used in the production of an anaerobic environment. It is used to culture bacteria which die or fail to grow in presence of oxygen (anaerobes).These are commercially available, disposable sachets containing a dry powder or pellets, which, when mixed with water and kept in an appropriately sized airtight jar, produce an atmosphere free of elemental oxygen gas (O2). They are used to produce an anaerobic culture in microbiology. It is a much simpler technique than the McIntosh and Filde's anaerobic jar where one needs to pump gases in and out. Constituents of gas-pak sachets 1.Sodium borohydride - NaBH4 2.Sodium bicarbonate - NaHCO3 3.Citric acid - C3H5O(COOH)3 4.Cobalt chloride - CoCl2 (catalyst) The addition of a Dicot Catalyst maybe required to initiate the reaction. Reactions NaBH4 + 2 H2O = NaBO2 + 4 H2↑ C3H5O(COOH)3 + 3 NaHCO3 + [CoCl2] = C3H5O(COONa)3 + 3 CO2 + 3 H2 + [CoCl2] 2 H2 + O2 + [Catalyst] = 2 H2O + [Catalyst] Consumption of oxygen These chemicals react with water to produce hydrogen and carbon dioxide along with sodium citrate and water (C3H5O(COONa)3) as byproducts. Again, hydrogen and oxygen reacting on a catalyst like Palladiumised alumina (supplied separately) combine to form water. Culture method The nedium, the gas-pak sachet (opened and with water added) and an indicator are placed in an air-tight gas jar which is incubated at the desired temperature. The indicator tells whether the environment was indeed oxygen free or not. 26 The chemical indicator generally used for this purpose is "chemical methylene blue solution" that since synthesis has never been exposed to elemental oxygen. It is colored deep blue on oxidation in presence of atmospheric oxygen in the jar, but will become colorless when oxygen is gone, and anaerobic conditions are achieved. 27 CHAPTER SEVEN MICROSCOPE 28 29 Working Principle and Parts of a Compound Microscope (with Diagrams) The most commonly used microscope for general purposes is the standard compound microscope. It magnifies the size of the object by a complex system of lens arrangement.It has a series of two lenses; (i) the objective lens close to the object to be observed and (ii) the ocular lens or eyepiece, through which the image is viewed by eye. Light from a light source (mirror or electric lamp) passes through a thin transparent object. The objective lens produces a magnified ‘real image’ first image) of the object. This image is again magnified by the ocular lens (eyepiece) to obtain a magnified ‘virtual image’ (final image), which can be seen by eye through the eyepiece. As light passes directly from the source to the eye through the two lenses, the field of vision is brightly illuminated. That is why; it is a bright-field microscope. Parts of a Compound Microscope: The parts of a compound microscope are of two categories as given below: (i) Mechanical Parts: These are the parts, which support the optical parts and help in their adjustment for focusing the object The components of mechanical parts are as follows: 1. Base or Metal Stand: The whole microscope rests on this base. Mirror, if present, is fitted to it. 2. Pillars: It is a pair of elevations on the base, by which the body of the microscope is held to the base 3. Inclination joint: It is a movable joint, through which the body of the microscope is held to the base by the pillars. The body can be bent at this joint into any inclined position, as desired by the observer, for easier observation. In new models, the body is permanently fixed to the base in an inclined position, thus needing no pillar or joint. 30 4. Curved Arm: It is a curved structure held by the pillars. It holds the stage, body tube, fine adjustment and coarse adjustment. 5. Body Tube: It is usually a vertical tube holding the eyepiece at the top and the revolving nosepiece with the objectives at the bottom. The length of the draw tube is called ‘mechanical tube length’ and is usually 140-180 mm (mostly 160 mm). 6. Draw Tube: It is the upper part of the body tube, slightly narrower, into which the eyepiece is slipped during observation. 7. Coarse Adjustment: It is a knob with rack and pinion mechanism to move the body tube up and down for focusing the object in the visible field. As rotation of the knob through a small angle moves the body tube through a long distance relative to the object, it can perform coarse adjustment. In modern microscopes, it moves the stage up and down and the body tube is fixed to the arm. 8. Fine Adjustment: It is a relatively smaller knob. Its rotation through a large angle can move the body tube only through a small vertical distance. It is used for fine adjustment to get the final clear image. In modern microscopes, fine adjustment is done by moving the stage up and down by the fine adjustment. 9. Stage: It is a horizontal platform projecting from the curved arm. It has a hole at the center, upon which the object to be viewed is placed on a slide. Light from the light source below the stage passes through the object into the objective. 31 10. Mechanical Stage (Slide Mover): Mechanical stage consists of two knobs with rack and pinion mechanism. The slide containing the object is clipped to it and moved on the stage in two dimensions by rotating the knobs, so as to focus the required portion of the object. 11. Revolving Nosepiece: It is a rotatable disc at the bottom of the body tube with three or four objectives screwed to it. The objectives have different magnifying powers. Based on the required magnification, the nosepiece is rotated, so that only the objective specified for the required magnification remains in line with the light path. (ii) Optical Parts: These parts are involved in passing the light through the object and magnifying its size. The components of optical parts include the following: 1. Light Source: Modern microscopes have in-built electric light source in the base. The source is connected to the mains through a regulator, which controls the brightness of the field. But in old models, a mirror is used as the light source. It is fixed to the base by a binnacle, through which it can be rotated, so as to converge light on the object. The mirror is plane on one side and concave on the other. It should be used in the following manner: (a) Condenser Present: Only plane side of the mirror should be used, as the condenser converges the light rays. (b) Condenser Absent: (i) Daylight: 32 Plane or concave (plane is easier) (ii) Small artificial light: High power objective: Plane side Low power objective: Concave side 2. Diaphragm: If light coming from the light source is brilliant and all the light is allowed to pass to the object through the condenser, the object gets brilliantly illuminated and cannot be visualized properly. Therefore, an iris diaphragm is fixed below the condenser to control the amount of light entering into the condenser. 3. Condenser: The condenser or sub-stage condenser is located between the light source and the stage. It has a series of lenses to converge on the object, light rays coming from the light source. After passing through the object, the light rays enter into the objective. The ‘light condensing’, ‘light converging’ or ‘light gathering’ capacity of a condenser is called ‘numerical aperture of the condenser’. Similarly, the ‘light gathering’ capacity of an objective is called ‘numerical aperture of the objective’. If the condenser converges light in a wide angle, its numerical aperture is greater and vice versa. If the condenser has such numerical aperture that it sends light through the object with an angle sufficiently large to fill the aperture back lens of the objective, the objective shows its highest numerical aperture. Most common condensers have numerical aperture. If the numerical aperture of the condenser is smaller than that of the objective, the peripheral portion of the back lens of the objective is not illuminated and the image has poor visibility. On the other hand, if the numerical aperture of condenser is greater than that of the objective, the back lens may receive too much light resulting in a decrease in contrast. 33 There are three types of condensers as follows: (a) Abbe condenser (Numerical aperture=1.25): It is extensively used. (b) Variable focus condenser (Numerical aperture =1.25) (c) Achromatic condenser (Numerical aperture =1.40): It has been corrected for both spherical and chromatic aberration and is used in research microscopes and photomicrographs. 4. Objective: It is the most important lens in a microscope. Usually three objectives with different magnifying powers are screwed to the revolving nosepiece. The objectives are: (a) Low power objective (X 10): It produces ten times magnification of the object. (b) High dry objective (X 40): It gives a magnification of forty times. (c) Oil-immersion objective (X100): It gives a magnification of hundred times, when immersion oil fills the space between the object and the objective The scanning objective (X4) is optional. The primary magnification (X4, X10, X40 or X100) provided by each objective is engraved on its barrel. The oil-immersion objective has a ring engraved on it towards the tip of the barrel. Resolving Power of Objective: It is the ability of the objective to resolve each point on the minute object into widely spaced points, so that the points in the image can be seen as distinct and separate from one another, so as to get a clear un-blurred image. 34 It may appear that very high magnification can be obtained by using more number of high power lenses. Though possible, the highly magnified image obtained in this way is a blurred, one. That means, each point in the object cannot be found as widely spaced distinct and separate point on the image. Mere increase in size (greater magnification) without the ability to distinguish structural details (greater resolution) is of little value. Therefore, the basic limitation in light microscopes is one not of magnification, but of resolving power, the ability to distinguish two adjacent points as distinct and separate, i.e. to resolve small components in the object into finer details on the image. Resolving power is a function of two factors as given below: (a) Numerical aperture (N.A.) (b) Wavelength of the light (λ) (a) Numerical aperture: Numerical aperture is a numerical value concerned with the diameter of the objective lens in relation to its focal length. Thus, it is related to the size of the lower aperture of the objective, through which light enters into it. In a microscope, light is focused on the object as a narrow pencil of light, from where it enters into the objective as a diverging pencil. The angle 9 subtended by the optical axis (the line joining the centers of all the lenses) and the outermost ray still covered by the objective is a measure of the aperture called ‘half aperture angle’. A wide pencil of light passing through the object ‘resolves’ the points in the object into widely spaced points on the lens, so that the lens can produce these points as distinct and separate on the image. Here, the lens gathers more light. On the other hand, a narrow pencil of light cannot ‘resolve’ the points in the object into widely spaced points on the lens, so that the lens produces a blurred image. Here, the lens gathers less light. Thus, the greater is the width of the pencil of light entering into the objective, the higher is its ‘resolving power’. 35 The numerical aperture of an objective is its light gathering capacity, which depends on the site of the angle 8 and the refractive index of the medium existing between the object and the objective. Numerical aperture (n.a.) = n sin θ Where, n = Refractive index of the medium between the object and the objective and θ = Half aperture angle For air, the value of ‘n’ is 1.00. When the space between the lower tip of the objective and the slide carrying the object is air, the rays emerging through the glass slide into this air are bent or refracted, so that some portion of it do not pass into the objective. Thus, loss of some light rays reduces numerical aperture and decreases the resolving power. However, when this space is filled with an immersion oil, which has greater refractive index (n=1.56) than that of air (n=1.00), light rays are refracted or bent more towards the objective. Thus, more light rays enter into the objective and greater resolution is obtained. In oil immersion objective, which provides the highest magnification, the size of the aperture is very small. Therefore, it needs bending of more rays into the aperture, so that the object can be distinctly resolved. That is why, immersion oils, such as cedar wood oil and liquid paraffin are used to fill the gap between the object and the objective, while using oil-immersion objective. (b) Wavelength of light (λ): The smaller is the wavelength of light (λ), the greater is its ability to resolve the points on the object into distinctly visible finer details in the image. Thus, the smaller is the wavelength of light, the greater is its resolving power. Limit of resolution of objective (d): 36 The limit of resolution of an objective (d) is the distance between any two closest points on the microscopic object, which can be resolved into two separate and distinct points on the enlarged image. Points with their in-between distance less than ‘d’ or objects smaller than ‘d’ cannot be resolved into separate points on the image. If the resolving power is high, points very close to each other can be seen as clear and distinct. Thus, the limit of resolution (the distance between the two resolvable points) is smaller. Therefore, smaller objects or finer details can be seen, when’d’ is smaller. Smaller ‘d’ is obtained by increasing the resolving power, which in turn is obtained by using shorter wavelength of light (λ) and greater numerical aperture. Limit of resolution = d = λ/2 n.a. Where, λ = Wave length of light and n.a. = Numerical aperture of the objective. If λ green = 0.55 p and n.a. = 1.30, then d = λ/2 n.a. = 0.55/2 X 1.30 = 0.21 µ. Therefore, the smallest details that can be seen by a typical light microscope is having the dimension of approximately 0.2 µ. Smaller objects or finer details than this cannot be resolved in a compound microscope. 5. Eyepiece: The eyepiece is a drum, which fits loosely into the draw tube. It magnifies the magnified real image formed by the objective to a still greatly magnified virtual image to be seen by the eye. Usually, each microscope is provided with two types of eyepieces with different magnifying powers (X10 and X25). Depending upon the required magnification, one of the two eyepieces is inserted into the draw tube before viewing. Three varieties of eyepieces are usually available. 37 They are the Huygenian, the hyper plane and the compensating. Among them, the Huygenian is very widely used and efficient for low magnification. In this eyepiece, two simple Plano-convex lenses are fixed, one above and the other below the image plane of the real image formed by the objective. The convex surfaces of both the lenses face downward. The lens towards the objective is called ‘field lens’ and that towards eye, ‘eye lens’. The rays after passing through the eye lens come out through a small circular area known as Rams-den disc or eye point, where the image is viewed by the eye. Total magnification: The total magnification obtained in a compound microscope is the product of objective magnification and ocular magnification. Mt = Mob X Moc Where, Mt = Total magnification, Mob = Objective magnification and Moc = Ocular magnification If the magnification obtained by the objective (Mob) is 100 and that by the ocular (Moc) is 10, then total magnification (Mt) = Mob X Moc =100 X 10 =1000. Thus, an object of lq will appear as 1000 µ. Useful magnification: It is the magnification that makes visible the smallest resolvable particle. The useful magnification in a light microscope is between X1000 and X2000. Any magnification beyond X2000 makes the image blurred. 38 CHAPTER EIGHT SPECTROPHOTOMETER AND COLORIMETER A spectrophotometer is an instrument which is used to measure the intensity of electromagnetic radiation at different wavelengths. Important features of spectrophotometers are spectral bandwidth and the range of absorption or reflectance measurement. Spectrophotometers are generally used for the measurement of transmittance or reflectance of solutions, transparent or opaque solids such as polished gases or glass. They can also be designed to measure the diffusivity on any of the listed light ranges in electromagnetic radiation spectrum that usually covers around 200 nm- 2500 nm using different controls and calibrations. Spectrophotometry is a quantitative measurement of the reflection or transmission properties of a material as a function of wavelength. It is more specific than the common term electromagnetic spectroscopy which deals with visible light near ultraviolet and near infra-red. It does not cover the time resolved spectroscopic techniques which means that "anything that allows to measure temporal dynamics and kinetics of photo physical processes". Spectrophotometry involves the use of spectrophotometer. The basic measurement principle used by a spectrophotometer is relatively simple and easy to understand. 39 What are the different types of Spectrophotometers? There are 2 major classifications of spectrophotometer. They are single beam and double beam. A double beam spectrophotometer compares the light intensity between 2 light paths, one path containing the reference sample and the other the test sample. A single beam spectrophotometer measures the relative light intensity of the beam before and after the test sample is introduced. Even though, double beam instruments are easier and more stable for comparison measurements, single beam instruments can have a large dynamic range and is also simple to handle and more compact. How does a spectrophotometer work? Light source, diffraction grating, filter, photo detector, signal processor and display are the various parts of the spectrophotometer. The light source provides all the wavelengths of visible light while also providing wavelengths in ultraviolet and infra red range. The filters and diffraction grating separate the light into its component wavelengths so that very small range of wavelength can be directed through the sample. The sample compartment permits the entry of no stray light while at the same time without blocking any light from the source. The photo detector converts the amount of light which it had received into a current which is then sent to the signal processor which is the soul of the machine. The signal processor converts the simple current it receives into absorbance, transmittance and concentration values which are then sent to the display. How to use a spectrophotometer? Spectrophotometer is used to determine absorbency at certain wavelengths of a solution. This can be used to determine the concentration of a solution or to find an unknown substance. First, clean out the cuvette (a small rectangular transparent tube that holds the solution) in the machine making sure that throughout the experiment, we face it the same way into the machine each time. Any fingerprints or dirt on the cuvette can affect the results of the experiment. So, it is always essential to wear gloves. 40 Then, add the solute which would be the blank for the experiment. It is important not to add water to the blank so that it won’t affect the accuracy of the results. As a next step, the spectrophotometer is set to the desired wavelength. Insert the blank cuvette, making sure that the arrow is aligned each time. Then press the “set zero” to calibrate the spectrophotometer for that wave length. Now, introduce the solution in order to calculate its absorbency. Each time we change the wavelength, it is important to change blank for that wavelength. 1.The Measurement Principle Used by a Spectrophotometer The basic measurement principle used by a spectrophotometer is relatively simple and easy to understand.. (1) Solid Samples first the intensity of the measurement light beam, I0, is measured without the sample set. Then the sample is set in the path of the measurement light beam, and the intensity of the light beam after it passes through the sample, It, is measured. (2) Solution Samples a cell containing solvent is set in the path of the measurement light beam, and the intensity of the light beam after it passes through the cell, I0, is measured. Next, a cell containing a solution produced by dissolving the sample in the solvent is set in the path of the measurement light beam, and the intensity of the light beam after it passes through the cell, It, is measured. The transmittance, T, is given by equation (1), but with solution samples, it is more common to use the absorbance, Abs, which is given by equation (2). Equation (3), which expresses the relationship between the absorbance, Abs, and the sample concentration, C, is called the “Lambert-Beer law”. 41 There is a proportional relationship between the absorbance and concentration, and this forms the basis of quantitative analysis. Here, ε is the sample’s absorption coefficient and L is the cell’s optical path length. The measurement method shown in eliminates the influence of reflection from the cell surface and absorption by the solvent, and ensures that only the absorption due to the sample is measured. Monochromatic light is light that consists of a single wavelength. To be precise, it has a spectral bandwidth (slit width). For example, monochromatic light with a wavelength of 500 nm and a spectral bandwidth of 2 nm is light that covers a wavelength interval (full width at half maximum) spanning 499 and 501 nm. 2.The Configuration of a Spectrophotometer The indispensable elements of a spectrophotometer consist, as shown in of a light source, a spectrometer, a sample compartment, and a detector. Although I said in the previous section that the sample is exposed to monochromatic light, there are instruments in which white light is passed through the sample before being passed into the spectrometer. This method is employed in high-speed photometry instruments that use array detectors. 3.Light Source The desirable properties of a light source are as follows: a) Brightness across a wide wavelength range b) Stability over time c) A long service life d) Low cost Although there are no light sources that have all of these properties, the most commonly used light sources at the moment are the halogen lamps used for the visible and near-infrared regions and the deuterium lamps used for the ultraviolet region. Apart from these, xenon flash lamps are sometimes used. (1) Halogen Lamp 42 Emission Intensity Distribution of Halogen Lamp (3,000K) The principle for light emission is the same as that for a standard incandescent bulb. Electric current is supplied to a filament, the filament becomes hot, and light is emitted. The bulb in a halogen lamp is filled with inert gas and a small amount of a halogen. While the tungsten used as the filament evaporates due to the high temperature, the halide causes the tungsten to return to the filament. This helps create a bright light source with a long service life. The emission intensity distribution of a halogen lamp can be approximated using Planck’s law of radiation. It has relatively high levels of each of the properties a) to d) mentioned above. (2)Deuterium Lamp Emission Intensity Distribution of Deuterium Lamp1) A deuterium lamp is a discharge light source in which the bulb is filled with deuterium (D2) at a pressure of several hundred pascals. Although 400 nm is, in general, an approximate usage limit at the long wavelength end, because the degree of attenuation at this end is quite low, light of wavelengths greater than 400 nm is used. In the region beyond 400 nm, there are also large numbers of bright line spectra. Among these, the bright line spectra at 486.0 nm and 656.1 nm are particularly intense, and can be used for the wavelength calibration of spectrophotometers. 43 The usage limit at the short wavelength end is determined by the transmittance of the window material. 4.Monochrometer Cross Section of Diffraction Grating Spectroscopy is the technique of splitting light that consists of various wavelengths into components that correspond to those wavelengths. The element that splits this light is called a dispersive element. Prisms and diffraction gratings are typical dispersive elements. Prisms used to be commonly used as the dispersive elements in spectrometers, but recently, diffraction gratings have become the most commonly used type of dispersive element. The diffraction gratings used in spectrophotometers have from several hundred to approximately 2,000 parallel grooves per millimeter cut into them at equal intervals. If this diffraction grating is exposed to white light, because of interference, the white light is dispersed in a direction perpendicular to the grooves, and light components of specific wavelengths are reflected only in specific directions. λ1 to λ3 represent wavelengths. The wavelengths change continuously and so if a diffraction grating is exposed towhite light, it appears iridescent. The way that the clear side of a CD appearsto glitter with iridescence when it is exposed to light is based on the same mechanism as the spectroscopy performed with a diffraction grating. 5.Sample Compartment Two light beams pass through the compartment, and that this is therefore the sample compartment of a “double-beam spectrophotometer”. The monochromatic light that leaves the spectrometer is split into two beams before it enters the sample compartment. A spectrophotometer in which only one beam passes through the sample compartment is called a “single- beam spectrophotometer”. 44 In a standard configuration, the sample compartment contains cell holders that, hold square cells with optical path lengths of 10 mm. The various accessories are attached by replacing these cell holder units or by replacing the entire sample compartment. Among spectrophotometers of medium or higher grade that use photomultipliers, which will be described later, as detectors, there are models for which large sample compartments are made available in order to allow the analysis of large samples or the attachment of large accessories. 6.Detector The light beams that pass through the sample compartment enter the detector, which is the last element in the spectrophotometer. Photomultipliers and silicon photodiodes are typical detectors used with spectrophotometers for the ultraviolet and visible regions. For the near- infrared region, PbS photoconductive elements have always been used in the past, but recently, instruments incorporating InGaAs photodiodes have been sold. Silicon photodiode array detectors are used, in combination with the back spectroscopy method, for high-speed photometry instruments. Photomultipliers and silicon photodiodes are described below. (1) Photomultiplier 45 Spectral Sensitivity Characteristics of a Photomultiplier2) A photomultiplier is a detector that uses the fact that photoelectrons are discharged from a photoelectric surface when it is subjected to light (i.e., the external photoelectric effect). The photoelectrons emitted from the photoelectric surface repeatedly cause secondary electron emission in sequentially arranged dynodes, ultimately producing a large output for a relatively small light intensity. The most important feature of a photomultiplier is that it achieves a significantly high level of sensitivity that cannot be obtained with other optical sensors. If there is sufficient light intensity, this feature is not particularly relevant, but as the light intensity decreases, this feature becomes increasingly useful. For this reason, photomultipliers are used in high-grade instruments. The spectral sensitivity characteristics of a photomultiplier are mainly determined by the material of the photoelectric surface. (2) Silicon Photodiode 46 Spectral Sensitivity Characteristics of a Silicon COLORIMETER A colorimeter is a device used for measuring colours, or colorimetry. It measures the absorbance of different wavelengths of light in a solution. It can be used to measure the concentration of a known solute. A colorimeter is an instrument that compares the amount of light getting through a solution with the amount that can get through a sample of pure solvent. A colorimeter contains a photocell is able to detect the amount of light which passes through the solution under investigation. A colorimeter is a light- sensitive instrument that measures how much color is absorbed by an object or substance. It determines color based on the red, blue, and green components of light absorbed by the object or sample, much as the human eye does. When light passes through a medium, part of the light is absorbed, and as a result, there is a decrease in how much of the light reflected by the medium. 47 A colorimeter measures that change so users can analyze the concentration of a particular substance in that medium. The device works on the basis of Beer-Lambert's law, which states that the absorption of light transmitted through a medium is directly proportional to the concentration of the medium. Types of colorimeters There are many different types of colorimeters, including the color densitometer, which measures the density of primary colors, and the color photometer, which measures the reflection and transmission of color. Styles include digital, also called laboratory, and portable. Digital versions are most often used in a lab setting for sampling or in the classroom for educational purposes. Portable versions can be carried anywhere, regardless of environmental conditions, to test things like water and soil samples on site. The spectrophotometer, a type of photometer that measures light intensity, is often grouped together with colorimeters, but it is technically a different device. Both rely on Beer-Lambert's law to calculate the concentration of a substance in a solution, but they do so in different ways. A colorimeter measures only red, green, and blue colors of light, while a spectrophotometer can measure the intensity of any wavelength of visible light. In general, spectrophotometers are more complicated and less rugged than most colorimeters; they should be handled with utmost care and require regular recalibration. A colorimeter is a light-sensitive device used for measuring the transmittance and absorbance of light passing through a liquid sample. The device measures the intensity or concentration of the color that develops upon introducing a specific reagent into a solution. There are two types of colorimeters — color densitometers, which measure the density of primary colors, and color photometers, which measure the color reflection and transmission. Design of Colorimeter The three main components of a colorimeter are a light source, a cuvette containing the sample solution, and a photocell for detecting the light passed through the solution. The instrument is also equipped with either colored filters or specific LEDs to generate color. The output from a colorimeter may be displayed by an analog or digital meter in terms of transmittance or absorbance. 48 In addition, a colorimeter may contain a voltage regulator for protecting the instrument from fluctuations in mains voltage. Some colorimeters are portable and useful for on-site tests, while others are larger, bench-top instruments useful for laboratory testing. Working Principle The colorimeter is based on Beer-Lambert's law, according to which the absorption of light transmitted through the medium is directly proportional to the medium concentration. In a colorimeter, a beam of light with a specific wavelength is passed through a solution via a series of lenses, which navigate the colored light to the measuring device. This analyses the color compared to an existing standard. A microprocessor then calculates the absorbance or percent transmittance. If the concentration of the solution is greater, more light will be absorbed, which can be identified by measuring the difference between the amount of light at its origin and that after passing the solution. In order to determine the concentration of an unknown sample, several sample solutions of a known concentration are first prepared and tested. The concentrations are then plotted on a graph against absorbance, thereby generating a calibration curve. The results of the unknown sample are compared to that of the known sample on the curve to measure the concentration. Applications Colorimeters are widely used to monitor the growth of a bacterial or yeast culture. They provide reliable and highly accurate results when used for the assessment of color in bird plumage. They are used to measure and monitor the color in various foods and beverages, including vegetable products and sugar. Certain colorimeters can measure the colors that are used in copy machines, fax machines and printers. 49 Besides being used for basic research in chemistry laboratories, colorimeters have many practical applications such as testing water quality by screening chemicals such as chlorine, fluoride, cyanide, dissolved oxygen, iron, molybdenum, zinc and hydrazine. They are also used to determine the concentrations of plant nutrients such as ammonia, nitrate and phosphorus in soil or hemoglobin in blood. How a Colorimeter Works At its most basic, a colorimeter works by passing a specific wavelength of light through a solution, and then measuring the light that comes through on the other side. In most cases, the more concentrated the solution is, the more light will be absorbed, which can be seen in the difference between the light at its origin and after it has passed through the solution. To find the concentration of an unknown sample, several samples of the solution in which the concentration is known are first prepared and tested. These are then plotted on a graph with the concentration at one axis and the absorbance on the other to create a calibration curve; when the unknown sample is tested, the result is compared to the known samples on the curve to determine the concentration. Some types of colorimeters will automatically create a calibration curve based on an initial calibration. How to use a colorimeter There are several different types of instrument in Science Laboratories. The procedures are similar, but make sure you know which type of instrument you are using and always use the same machine for your measurements. In a colorimeter you are looking to see the change in colour as a reaction takes place. This is often a change from clear to a coloured solution, although it could be from one colour to another, or from a coloured solution to a clear one. Some colours are of more importance to a given reaction than others. In a particular reaction the wavelength of a given colour that is most affected by the reaction is referred to by the Greek letter lambda (for wavelength) and subscripted max (i.e. λmax to denote maximum absorption/transmission. The wavelength or colour can be selected by various means (e.g. LASER, LED, prismatic dispersion, etc.) but a cheap method is to use an optical slide as a filter. This allows a "band" of light frequencies to be used that contain the peak wavelength desired. 50 The filters are used to isolate a part of the visible light spectrum that is absorbed maximally by the sample. Different colourimeters use different sets of filters but typical wavelengths passed are red filter: 630-750nm, green filter: 510-570nm and blue filter: 360-480nm. Although you will normally be told which filter to use you should consider and understand the reason for this choice. Operating instructions for a typical colorimeter Switch on the instrument at least 5 minutes before use to allow it to stabilize. Select the most appropriate filter for the analysis and insert it in the light path (or dial it in with the selector) Place the reagent blank solution (or water) in the cuvette and zero the instrument (consult your manufacturers instruction about how to do this.) Make sure the clear faces of the cuvette are in the light path Place the sample in the colorimeter and read the absorbance of the solution. If the absorbance is "over range" (usually > 2.0) then the sample must be diluted to yield a value within the limits of the instrument. At intervals, recheck the reagent blank to ensure that there is no drift in the zero value. Notes Colour choice. Why red on a blue solution: (an example of why a red filter is used on a blue solution but can be developed for any other colour). The blue solution appears blue as all other colours have been preferentially absorbed. The Red is the most strongly absorbed. Remember: that if you want to see how the changes in the solution are progressing you need to study a colour that changes. IF the blue is always passing unhindered then red must be used. This is very counter intuitive. However if you want to convince yourself: shine a bright white light through a tank of water with milk. It appears blue. If you go to the end and look at the light source it will be reddish in tinge. Your machine must be re-zeroed (step 3) if a new filter is chosen. The sample cuvettes must be at least two-thirds full. Uses A colorimeter can be used in a wide variety of industries and settings. Small, portable devices can be used to analyze the color contrast and brightness on a television or computer screen, allowing the user to then adjust the settings to obtain the best quality picture. 51 In the printing industry, a colorimeter is a basic element in a color management system. Other printing industry applications include checking the electronic components and quality of pulp paper and measuring the quality of printing ink. Diamond merchants use colorimeters to measure the optical properties of precious stones. In cosmetology, the device is used to measure the sun protection factor of products applied to the skin. Colorimeters can analyze skin tones and tooth color to help diagnose certain diseases, and hospitals even use some types of this device to test the concentration of hemoglobin in blood. 52 CHAPTER NINE FLAME PHOTOMETERS FLAME PHOTOMETERS In flame photometry, a branch of atomic spectroscopy also called “flame atomic emission spectrometry,” atoms are examined in the spectrometer. This technique is suited to the quantitative and qualitative determination of a variety of cations—particularly for alkali and alkaline earth metals— since they are excited to higher levels of energy at low flame temperatures 53 Flame photometry is a process where in emission of radiation by neutral atoms is measured. The neutral atoms are obtained by introduction of sample into flame. Hence the name flame photometry. Since radiation is emitted it is also called as flame emission spectroscopy. Flame photometer working principle: When a solution of metallic salt is sprayed as fine droplets into a flame. Due to heat of the flame, the droplets dry leaving a fine residue of salt. This fine residue converts into neutral atoms. Due to the thermal energy of the flame, the atoms get excited and there after return to ground state. In this process of return to ground state, exited atoms emit radiation of specific wavelength. This wavelength of radiation emitted is specific for every element. This specificity of wavelength of light emitted makes it a qualitative aspect. While the intensity of radiation, depends on the concentration of element. This makes it a quantitative aspect. The process seems to be simple and applicable to all elements. But in practice only a few elements of Group IA and group IIA (like Li, Na, k & Ca, Mg) are only analyzed. The radiation emitted in the process is of specific wavelength. Like for Sodium (Na) 589nm yellow radiation, Potassium 767nm range radiation. Flame photometer instrumentation: 1. Burner 2. Monochromators 3. Detectors 54 4. Recorder and display. Burner: This is a part which produces excited atoms. Here the sample solution is sprayed into fuel and oxidant combination. A homogenous flame of stable intensity is produced. Total Consumption burner There are different types of burners like Total consumption burner, Laminar flow and Mecker burner. Fuel and oxidants: Fuel and oxidant are required to produce flame such that the sample converts to neutral atoms and get excited by heat energy. The temperature of flame should be stable and also ideal. If the temperature is high, the elements in sample convert into ions instead of neutral atoms. If it is too low, atoms may not go to exited state. So a combination of fuel and oxidants is used such that there is desired temperature. The following combination of fuel and oxidants are commonly used. Fuel Oxidant Temperature of Flame Propane + Air 2100 Degree C Propane + Oxygen 2800 Degree C Hydrogen + Air 1900 Degree C Hydrogen + Oxygen 2800 Degree C Acetylene + Air 2200 Degree C Acetylene + Oxygen 3000 Degree C 55 Monochromators: Filters and monochromators are needed to isolate the light of specific wavelength from remaining light of the flame. For this simple filters are sufficient as we study only few elements like Ca, Na, K and Li. So a filter wheel with filter for each element is taken. When a particular element is analyzed, the particular filter is used so that it filters all other wavelengths. Detector: Flame photometric detector is similar to that used in spectrophotometry. The emitted radiation is in the visible region i.e. 400nm to 700nm. Further the radiation is specific for each element so simple detectors are sufficient for the purpose like photo voltaic cells, photo tubes etc. Recorders and display: These are the devices to read out the recording from detectors. Flame photometer uses/ Applications 1. For qualitative analysis of samples by comparison of spectrum emission wavelengths with that of standards. 2. For quantitative analysis to determine the concentration of group IA and IIA elements. For example a) Concentration of calcium in hard water. b) Concentration of Sodium, potassium in Urine c) Concentration of calcium and other elements in bio-glass and ceramic materials. Flame photometry limitations: Unlike other spectroscopy methods, flame photometry finds little use in research and analysis. This is due to 1. Limited number of elements that can be analyzed. 56 2. The sample requires to be introduced as solution into fine droplets. Many metallic salts, soil, plant and other compounds are insoluble in common solvents. Hence, they can’t be analyzed by this method. 3. Since sample is volatilized, if small amount of sample is present, it is tough to analyze by this method. As some of it gets wasted by vaporization. 4. Further during solubilisation with solvents, other impurities might mix up with sample and may lead to errors in the spectra observed. How Flame Photometers Work These instruments are fairly simple, consisting of four basic components: a flame or “burner,” a nebulizer (or aspirator) and mixing chamber, color filters, and a photo detector. Flame photometers are also very cost effective and easy to use. In a flame photometer, the solution is aspirated through a nebulizer (or aspirator) into the flame. After the sample matrix evaporates, the sample is atomized. Atoms then reach an excited state by absorbing heat from the flame. When these excited atoms return to their lowest-energy state, they give off radiation in certain wavelengths, leading to the creation of a line spectrum. A filter pre-selected based on the atom being analyzed is used in flame photometry. The emission line’s intensity is then practically measured and is related to the solution’s original concentration. 57 CHAPTER TEN ION SELECTIVE ELECTRODES AND POTENTIOMETRY The pictures below show all the equipment needed for an Ion Analyser measurement: One Ion-Selective electrode and one Reference electrode are inserted into a dual electrode head. The head, one temperature sensor and one pH electrode are connected to a 2-channel electrode-computer interface. The interface is connected to a serial or USB port of a computer running the 2-channel measurement software. The computer screen shows the calibration graph for a potentiometric ammonium measurement, with sample results plotted on it. 58 Simple laboratory set-up for Ion Selective Electrode calibration and measurement Ion Selective Electrode, Reference Electrode, Electrode Head, pH electrode, Temperature Sensor, Electrode-Computer Interface, Ion Analyser Software, Comprehensive Instructions. a) Applications 59 Ion-selective electrodes are used in a wide variety of applications for determining the concentrations of various ions in aqueous solutions. The following is a list of some of the main areas in which ISEs have been used. Pollution Monitoring: CN, F, S, Cl, NO3 etc., in effluents, and natural waters. Agriculture: NO3, Cl, NH4, K, Ca, I, CN in soils, plant material, fertilisers and feedstuffs. Food Processing: NO3, NO2 in meat preservatives. Salt content of meat, fish, dairy products, fruit juices, brewing solutions. F in drinking water and other drinks. Ca in dairy products and beer. K in fruit juices and wine making. Corrosive effect of NO3 in canned foods. Detergent Manufacture: Ca, Ba, F for studying effects on water quality. Paper Manufacture: S and Cl in pulping and recovery-cycle liquors. Explosives: F, Cl, NO3 in explosive materials and combustion products. Electroplating: F and Cl in etching baths; S in anodising baths. Biomedical Laboratories: Ca, K, Cl in body fluids (blood, plasma, serum, sweat). F in skeletal and dental studies. 60 Education and Research: Wide range of applications. b) Advantages. 1) When compared to many other analytical techniques, Ion-Selective Electrodes are relatively inexpensive and simple to use and have an extremely wide range of applications and wide concentration range. 2) The most recent plastic-bodied all-solid-state or gel-filled models are very robust and durable and ideal for use in either field or laboratory environments. 3) Under the most favourable conditions, when measuring ions in relatively dilute aqueous solutions and where interfering ions are not a problem, they can be used very rapidly and easily (e.g. simply dipping in lakes or rivers, dangling from a bridge or dragging behind a boat). 4) They are particularly useful in applications where only an order of magnitude concentration is required, or it is only necessary to know that a particular ion is below a certain concentration level. 5) They are invaluable for the continuous monitoring of changes in concentration: e.g. in potentiometric titrations or monitoring the uptake of nutrients, or the consumption of reagents. 6) They are particularly useful in biological/medical applications because they measure the activity of the ion directly, rather than the concentration. 7) In applications where interfering ions, pH levels, or high concentrations are a problem, then many manufacturers can supply a library of specialised experimental methods and special reagents to overcome many of these difficulties. 8) With careful use, frequent calibration, and an awareness of the limitations, they can achieve accuracy and precision levels of ± 2 or 3% for some ions and thus compare favourably with analytical techniques which require far more complex and expensive instrumentation. 61 9) ISEs are one of the few techniques which can measure both positive and negative ions. 10) They are unaffected by sample colour or turbidity. 11) ISEs can be used in aqueous solutions over a wide temperature range. Crystal membranes can operate in the range 0°C to 80°C and plastic membranes from 0°C to 50°C. Ion-Selective Electrodes are part of a group of relatively simple and inexpensive analytical tools which are commonly referred to as Sensors. The pH electrode is the most well-known and simplest member of this group and can be used to illustrate the basic principles of ISEs. a) The pH Electrode This is a device for measuring the concentration of hydrogen ions and hence the degree of acidity of a solution - since pH is defined as the negative logarithm of the hydrogen ion concentration; i.e. pH=7 means a concentration of 1x10-7 moles per litre. (To be more precise, the term ‘concentration’ should really be replaced by ‘activity’ or ‘effective concentration’. This is an important factor in ISE measurements. The difference between activity and concentration is explained in more detail later, but it may be noted here that in dilute solutions they are essentially the same. The most essential component of a pH electrode is a special, sensitive glass membrane which permits the passage of hydrogen ions, but no other ionic species. When the electrode is immersed in a test solution containing hydrogen ions the external ions diffuse through the membrane until an equilibrium is reached between the external and internal concentrations. Thus there is a build up of charge on the inside of the membr