Interventions - Response to Exercise, Pain, & Range of Motion
Document Details
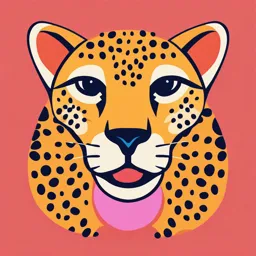
Uploaded by DivineSagacity2178
Tags
Summary
This document provides information about youth exercise, responses to exercise, pain, and ROM. The document covers topics such as: physiological adaptation, resistance training, injury risks, and strength gains. The document also discusses different types of ROM techniques, their therapeutic goals, and the factors that need to be considered for an individual's health. Further information is given on pain mechanisms, pain assessment, and questionnaires.
Full Transcript
2.1 Youth Exercise The lecture covered the physiological, developmental, and practical considerations of exercise in children and adolescents. It emphasized that youth are physiologically adaptive to aerobic, resistance, and bone-loading exercises, highlighting benefits such as improved strength, c...
2.1 Youth Exercise The lecture covered the physiological, developmental, and practical considerations of exercise in children and adolescents. It emphasized that youth are physiologically adaptive to aerobic, resistance, and bone-loading exercises, highlighting benefits such as improved strength, cardiometabolic health, psychosocial well-being, and reduced injury risks. It also addressed the influence of growth, maturation, and puberty on exercise outcomes and offered guidelines for safe and effective youth resistance training programs. Key Points 1. Physiological Adaptation and Benefits ○ Children and adolescents can adapt to aerobic, resistance, and bone-loading exercises. ○ Prepubescent children can achieve strength gains comparable to adolescents through resistance training. ○ Exercise benefits include: Improved cardiometabolic risk factors and weight control. Enhanced bone strength and psychosocial well-being. Potential to prevent sports-related injuries. ○ Physical activity positively impacts cognition and academic achievement. 2. Resistance Training in Youth ○ Increases muscular strength, power, endurance, and motor skills. ○ Reduces sports and recreational injuries. ○ Improves psychosocial and anatomical parameters without stunting growth. ○ Preseason conditioning with resistance training reduces injury risks. 3. Growth and Maturation ○ Children grow at variable rates; chronological age doesn’t accurately reflect maturation. ○ Biological age (e.g., skeletal or sexual maturation) better represents development. ○ Puberty onset: Girls: 8–13 years, typically 2 years before boys. Boys: 9–15 years. ○ Peak height velocity: Girls: ~12 years. Boys: ~14 years. ○ Hormonal changes during puberty: Boys: Increased muscle mass and strength. Girls: Increased fat deposition and widened hips. 4. Injury Risks and Skeletal Development ○ Growth cartilage (epiphyseal plate, joint surface, apophyseal insertions) is vulnerable to trauma. ○ Overuse or improper exercise can cause growth plate injuries, leading to long-term issues. ○ Peak height velocity increases risk for muscle imbalances and overuse injuries. 5. Muscle Development ○ Muscle mass increases from 25% of body weight at birth to ~40% in adulthood. ○ Puberty accelerates muscle hypertrophy, especially in boys due to hormonal influences. ○ Muscle hypertrophy in girls occurs at a slower rate. 6. Exercise Guidelines for Youth ○ Resistance training is safe with proper supervision, instruction, and progression. ○ Recommendations: Begin with low-to-moderate intensity (6–15 reps). Gradual load increases (5–10%) as technique improves. 1–4 sets per exercise, 2–3 non-consecutive days per week. Include multi-modality exercises (free weights, machines, etc.). ○ Emphasize intrinsic goals: skill improvement, personal success, and enjoyment. 7. Safety Considerations ○ Avoid sustained heavy exercise in hot environments; ensure hydration. ○ Untrained youth should start with moderate activity and gradually increase intensity. ○ Warm-up and cool-down exercises are essential. ○ Use appropriately sized equipment in a safe environment. 8. Strength Gains and Trainability ○ Strength gains in pre-adolescents are mainly due to neural adaptations (e.g., motor unit recruitment). ○ Muscle hypertrophy becomes prominent during and after puberty. ○ Gains are impermanent and require continued progressive training. 9. Youth-Specific Needs ○ Younger children’s immature skeletons require moderate exercise loads. ○ Myelination of motor neurons is incomplete until sexual maturation, impacting skill performance. ○ Structured and unstructured physical activity (e.g., active play) is important for young children. 10.Evidence and Best Practices ○ Research supports resistance training as safe and effective for children as young as six when supervised. ○ One-rep max testing is safe with proper guidelines. ○ Systematic periodization and variation in training programs ensure continued development. 11.Professional Recommendations ○ National Strength and Conditioning Association endorses youth resistance training. ○ Focus on technical proficiency, safe progression, and tailored programs. ○ Encourage fun, age-appropriate activities to sustain participation. 2.2 Older Adults Exercise The lecture highlights age-related differences and exercise implications for older adults, emphasizing that health and functional status, rather than chronological age, are better indicators of physical activity capacity. Aging leads to loss of bone density, muscle mass, and strength, increasing the risk of falls and disability. Resistance training can mitigate these effects by improving strength, muscle mass, and bone health, though it should be supplemented with balance and flexibility training to reduce fall risk. Older adults benefit from resistance training tailored to their needs, starting with low intensity and gradually increasing to higher intensity and advanced exercises like free weights and balance training. Proper nutrition, adequate protein intake, and careful progression are critical. High-velocity power training shows promise for enhancing functional abilities, and training frequency of twice weekly is recommended initially to allow for recovery. The principles of exercise prescription for older adults align with those for younger individuals, with considerations for pre-existing conditions and recovery needs. Key Points 1. Definition of Older Adults ○ Individuals 65 years or older. ○ Individuals 50–64 years with conditions or limitations affecting movement or fitness. ○ Aging effects vary across individuals, with health and functional status better indicators of exercise capacity than age. 2. Age-Related Physiological Changes ○ Bone and Muscle Changes: Loss of bone mineral content increases fragility and fracture risk. Loss of muscle mass and strength (sarcopenia) begins after age 30. Declines include: Muscle density. Tendon compliance. Increased intramuscular fat. 3. Factors Influencing Fall Risk: ○ Decreased muscle strength and power. ○ Impaired balance and postural stability. 4. Exercise Prescription Principles for Older Adults ○ Intensity adjusted based on individual fitness using a 10-point perceived exertion scale: Moderate intensity: 5–6. Vigorous intensity: 7+. ○ Resistance training: Improves muscle strength, endurance, power, and reduces body fat. Enhances bone mineral density, reducing fracture risk. 5. Training Considerations ○ Initial Program: Start with 1 set of 8–12 reps at low intensity (40–50% 1RM). Gradually increase to 3 sets at 60–80% 1RM. 6. Advanced Techniques: ○ Incorporate high-velocity power exercises after building a base. ○ Use multi-joint, functional movements like free weights and medicine ball drills. 7. Rest and Recovery: ○ Allow longer recovery between sessions. ○ Recommended frequency: twice per week, especially for beginners. 8. Avoid Risks: ○ Monitor for Valsalva maneuver during lifts (can spike blood pressure). ○ Avoid rapid progression in intensity or technically complex exercises. 9. Nutrition: ○ Adequate protein intake is critical for muscle hypertrophy and recovery. 10.Benefits of Resistance Training in Older Adults ○ Improves functional capabilities: Gait speed, balance, and stair climbing ability. Reduces fall risk when combined with balance and flexibility training. ○ Power-specific training: Enhances explosive force production and neuromuscular activation. More effective than traditional resistance training for power development. ○ Long-term adaptations: Gains in muscle hypertrophy, strength, and metabolic health (increased resting metabolic rate). 11.Programming Tips ○ Vary volume and intensity to avoid overtraining and promote progress. ○ Progress to advanced exercises, e.g., single-leg stance, once basic exercises are mastered. ○ Balance machine and free weight use based on abilities and postural stability. 2.3 Sex Differences Exercise Sex-related differences in exercise stem from variations in body composition, muscle mass, and hormonal influences. Women typically have more body fat, less muscle mass, and lower bone mineral density than men, with relative strength differences narrowing when adjusted for body size or fat-free mass. While absolute upper body strength in women is lower, lower body strength is more comparable to men’s. Women respond similarly to resistance training as men, with comparable relative gains despite smaller absolute increases. Programs should focus on individual needs, with an emphasis on upper body development for women in strength-demanding sports. Women generally exhibit greater flexibility due to hormonal factors like estrogen and relaxin, anatomical differences, and frequent participation in flexibility-centric activities. These distinctions highlight the need for tailored approaches in training to optimize performance and safety. Key Points 1. Body Composition and Strength: ○ Estrogen effects: Increases fat deposition, breast development, and affects body composition in women during puberty. ○ Women typically have: More body fat, less muscle mass, and lower bone mineral density compared to men. Lower absolute strength (⅔ of men’s strength), especially in upper body; lower body strength is closer to men’s values. Similar eccentric strength relative to fat-free mass but lower concentric strength. ○ Strength relative to: Body weight: Women’s lower body strength is comparable to men’s; upper body strength is lower. Fat-free mass and muscle cross-sectional area: Differences disappear, showing muscle quality is not sex-specific. 2. Power and Training Responses: ○ Women’s power output relative to body weight is 63% of men’s. ○ Women can increase strength at the same or faster rates than men in resistance training. Absolute gains are generally greater in men, but relative gains are similar or greater in women. ○ Training programs for men and women should focus on muscle groups relevant to the activity or sport, with differences only in the amount of absolute resistance. 3. Upper Body Strength and Flexibility: ○ Women may benefit from: Additional upper body exercises or extra sets due to lower upper body strength. Emphasis on multi-joint free weight exercises where feasible. ○ Women are generally more flexible than men due to: Hormonal factors: Higher levels of estrogen and relaxin, especially during pregnancy, increase joint laxity and flexibility. Anatomical differences: Wider pelvis and different muscle attachment sites favor greater range of motion. Activity levels: Engagement in flexibility-focused activities like dance and gymnastics. 4. Key Takeaways: ○ Resistance training programs can be similar for both sexes, tailored to individual needs. ○ Women’s training may need adjustments for upper body strength and flexibility considerations. ○ Flexibility advantages in women are attributed to both biological and activity-related factors. 2.4 Endocrine Response to Exercise The endocrine response to exercise is governed by the general adaptation syndrome, where the adrenal gland initially reacts with a decrease in function to stress but eventually adapts, increasing resistance to stress over time. This adaptation, particularly in response to exercise, involves changes in hormone production, receptor content, and the body's tolerance to stress. Acute and chronic changes can occur, with hormones like insulin, glucagon, epinephrine, norepinephrine, growth hormone, cortisol, and testosterone playing key roles in regulating muscle, bone, and connective tissue integrity. High-intensity aerobic exercise stimulates greater hormone secretion, while trained athletes show less hormonal response during submaximal exercise. Aerobic training, especially running, can increase protein breakdown, yet endurance training may still lead to muscle hypertrophy, primarily through mitochondrial rather than contractile proteins. Key Points 1. General Adaptation Syndrome and Training Adaptations ○ General adaptation syndrome: Describes adrenal gland response to stress, starting with an alarm phase (initial function reduction) followed by resistance above baseline. ○ Adaptation: Increased resistance to stress due to exercise is termed a training adaptation. ○ Effective adaptation requires timely removal of stress to allow recovery before progressive overload. 2. Exercise Adaptations ○ Most research focuses on early to intermediate training stages (4-24 weeks). ○ Adaptations relate to target organs and exercise stress tolerance. ○ Endocrine adaptations include: i. Acute changes during/after exercise. ii. Chronic changes in acute responses. iii. Chronic changes in resting hormone concentrations. iv. Changes in hormone receptor content. 3. Hormone Adaptation Insights ○ Chronic changes in resting hormone levels (e.g., testosterone, growth hormone, cortisol) are unlikely; responses depend on training and nutrition. ○ Hormonal changes include: i. Insulin and glucagon: Blood glucose control. ii. Epinephrine and norepinephrine: Fight-or-flight response. iii. Growth hormone, cortisol, testosterone, thyroid hormones, endorphins. 4. Resistance and Aerobic Training Responses ○ Resistance training: Hormones (testosterone, IGF-I, growth hormone) support muscle, bone, connective tissue integrity, and metabolism. ○ Aerobic training: i. Increases hormonal circulation and receptor-level changes. ii. Higher intensity training increases absolute hormone secretion during maximal exercise. iii. Trained athletes have reduced hormonal responses to submaximal exercise but maintain similar concentrations at comparable intensities. 5. High-Intensity and Short-Duration Exercise ○ 5-10 seconds of very high-intensity exercise elicits fight-or-flight hormone responses (epinephrine/norepinephrine increase). 6. Aerobic Training and Protein Dynamics ○ Aerobic exercise (e.g., running) increases net protein breakdown due to cortisol but is offset by anabolic hormones like testosterone and IGF-I. ○ Endurance training may lead to muscle hypertrophy through increased mitochondrial proteins rather than contractile proteins. 7. Key Points Summary: ○ General adaptation syndrome underpins exercise-induced stress responses and adaptations. ○ Timely recovery and progressive overload drive effective training adaptations. ○ Exercise-induced endocrine changes include acute, chronic, and receptor-level adjustments. ○ Hormonal responses depend on exercise type, intensity, and training status. ○ Resistance training improves muscle and tissue integrity, while aerobic training enhances endurance and mitochondrial protein synthesis. 2.5 Neuromuscular Response to Exercise The neuromuscular response to exercise involves several neural adaptations that improve muscle performance. These include increased motor unit recruitment, better synchronization and firing rates of motor neurons, enhanced neuromuscular junction efficiency, reduced co-contraction of antagonist muscles, and improved intermuscular coordination. These changes help enhance strength, endurance, and reaction times. Anabolic training induces long-term adaptations in the neuromuscular system, increasing neural drive, which is essential for maximizing strength and power. Neural adaptations, such as selective recruitment of motor units and changes in firing rates, occur before muscle structural changes. Training also influences fast-twitch fiber recruitment, firing rates, and muscle hypertrophy. In advanced lifters, the central nervous system adapts to recruit larger motor units first for power and speed. Cross-education and the bilateral deficit phenomena further illustrate neural adaptations, with strength gains in untrained limbs and lower force production during bilateral contractions. Additionally, antagonist muscle co-contraction serves to stabilize joints but can hinder maximal force production. Aerobic training also leads to improved efficiency, delaying fatigue and reducing energy expenditure. Key Points 1. Neural Adaptations to Training: ○ Increased motor unit recruitment and synchronization for better force production. ○ Improved firing rates of motor neurons for greater endurance and force. ○ Increased efficiency of neuromuscular junctions for better signal transmission. ○ Reduced co-contraction of antagonist muscles, easing movement. ○ Enhanced activation of agonist muscles and intermuscular coordination. ○ Improved reflex responses and reaction times. ○ Changes in the central nervous system (e.g., increased cortical activity). 2. Anabolic Training Effects: ○ Long-term adaptations throughout the neuromuscular system, from brain centers to individual muscle fibers. ○ Neural adaptations precede structural changes in muscle tissue. ○ Increased neural drive enhances muscular strength and power. ○ Training reduces inhibitory mechanisms and improves muscle recruitment. 3. Motor Unit Recruitment and Synchronization: ○ Fast-twitch motor fiber recruitment increases during training for high force expression. ○ Untrained individuals have limited ability to recruit motor units, particularly fast-twitch fibers. ○ The size principle governs recruitment order, but athletes can selectively recruit higher threshold units. ○ Plyometrics and Olympic lifting favor fast-twitch fiber recruitment for power and speed. 4. Motor Unit Adaptations: ○ Muscle hypertrophy reduces the need for high neural activation to lift the same load. ○ High firing rates enhance force production, especially in smaller muscles. ○ Larger muscles depend more on motor unit recruitment than firing rates. 5. Cross-Education and Bilateral Deficit: ○ Resistance training of one limb can increase strength in the opposite, untrained limb (cross-education). ○ Strength in untrained limbs can increase by up to 22% with corresponding EMG activity. ○ Bilateral deficit: force produced by both limbs together is lower than the sum of individual forces due to lower EMG activity during bilateral contractions. 6. Antagonist Muscle Co-Contraction: ○ Co-contraction increases joint stability but can reduce maximal force production if excessive. 7. Neurological Role in Aerobic Endurance: ○ Early stages of endurance training involve improved efficiency and delayed fatigue. ○ Neural activity rotation between synergists and motor units increases efficiency and reduces energy expenditure. 2.6 NMS Response to Aerobic Training Aerobic exercise training leads to several neuromuscular adaptations, primarily an increase in aerobic capacity, allowing athletes to perform more efficiently at higher relative intensities. Key adaptations include glycogen sparing and enhanced fat utilization, which prolong performance. Blood lactate accumulation occurs at higher intensities, and muscle fiber adaptations reduce lactic acid production. Chronic physiological changes include improved muscular endurance, increased aerobic power, and no significant changes in anaerobic power or sprint speed. Type 1 muscle fibers show selective hypertrophy, with cellular changes such as increased mitochondria and myoglobin, enhancing oxygen utilization. Bone and connective tissues respond to increased loading, with high-intensity exercise promoting bone growth and cartilage thickening. Aerobic exercise also leads to reduced body fat and no change in fat-free mass. Studies on cartilage and joint health show moderate running may enhance cartilage thickness, although excessive running may have detrimental effects. Key Points 1. Fundamental Adaptation: ○ Increased aerobic capacity of trained muscles allows athletes to perform at higher relative intensities. ○ Glycogen sparing and increased fat utilization prolong performance at the same intensity. ○ Blood lactate accumulation occurs at a higher percentage of aerobic capacity in trained athletes. 2. Chronic Physiological Adaptations: ○ Increased muscular endurance for low-power output. ○ Increased aerobic power with no change in anaerobic power. Stored ATP, stored creatine phosphate, stored glycogen and stored triglycerides increase with chronic physiological adaptations to aerobic exercise ○ No change or decrease in maximum rate of force production, vertical jump ability, and sprint speed. 3. Muscle Fiber Adaptations: ○ Type 1 fibers have higher pre-existing aerobic capacity and increase in aerobic potential with training. ○ Type 1 fibers experience selective hypertrophy due to increased recruitment, though not as much as Type 2 fibers from resistance training. 4. Cellular Adaptations: ○ Increase in the size and number of mitochondria and myoglobin content, which enhances oxygen delivery and ATP production. ○ Increase in enzymes related to aerobic metabolism and stores of glycogen and triglycerides. 5. Bone Adaptations: ○ Intense aerobic exercise like running stimulates bone growth. ○ To stimulate bone formation, intensity must exceed daily activity levels and increase to continuously overload the bone. ○ High-intensity interval training can enhance osteogenic stimulus. 6. Connective Tissue Adaptations: ○ Tendons, ligaments, and cartilage strengthen in proportion to exercise intensity, especially with weight-bearing exercises. ○ Weight-bearing exercises increase cartilage thickness, particularly in joints exposed to load. ○ Full range of motion during weight-bearing is essential for maintaining tissue viability. 7. Body Composition: ○ Aerobic training leads to a decrease in body fat percentage with no change in fat-free mass. 8. Research on Cartilage: ○ Strenuous running (e.g., 12.5 miles/session) may decrease cartilage thickness, but moderate running (e.g., 1 hour/day, 5 days/week) increases cartilage thickness and stimulates bone tissue remodeling. 2.7 NMS Response to Resistance Training This lecture discusses the neuromuscular responses and physiological adaptations to resistance training. It outlines how muscular strength can increase by 40% in untrained individuals, with lesser gains in trained individuals. The effects of resistance training on muscle fiber types, power output, muscular endurance, oxidative capacity, and flexibility are covered. It highlights the impact of hypertrophy, including muscle fiber enlargement, and the debated concept of hyperplasia. Additionally, the lecture addresses how resistance training alters myofibrillar volume, mitochondrial density, capillary density, pennation angle, and fascicle length, contributing to improved muscle strength. The role of resistance training in bone and connective tissue adaptation is also discussed, emphasizing the importance of progressive overload, joint force vectors, and structural exercises. Finally, it touches on body composition changes, such as fat loss and lean mass gain, and the potential increase in metabolic rate due to resistance training. Key Points 1. Muscular Strength Gains: ○ Strength can increase by 40% in untrained individuals, 20% in moderately trained, and 16% in trained individuals over periods of 4 weeks to 2 years. 2. Muscle Fiber Adaptations: ○ Resistance training shifts muscle fiber types, increasing the recruitment of higher-order motor units. ○ Type II fibers increase more in size than Type I fibers with resistance training. 3. Hypertrophy Mechanisms: ○ Hypertrophy involves increased synthesis and decreased degradation of actin and myosin in muscle fibers. ○ Myofibrils increase in number and size, leading to muscle enlargement. ○ Hyperplasia (increase in muscle fiber number) is debated in humans, though it occurs in animals. 4. Muscle Fiber Type and Hypertrophy: ○ Athletes with a higher proportion of Type II fibers may have greater potential for muscle mass increase. ○ Muscle fiber transformations (Type I to Type II or vice versa) are unlikely. 5. Muscle Structural Changes: ○ Resistance training increases myofibrillar volume, cytoplasmic density, sarcoplasmic reticulum and T tubule density, and sodium-potassium ATPase activity, which facilitates hypertrophy. ○ Mitochondrial and capillary density decreases relative to muscle volume with hypertrophy. 6. Pennation Angle and Fascicle Length: ○ Resistance training increases pennation angle, which aids in force production and cross-sectional area. ○ Strength-trained athletes show increased fascicle length, which improves force transmission to tendons and bones. 7. Energy Stores and Glycogen: ○ ATP and creatine phosphate concentrations increase with training (28% and 18%, respectively). ○ Bodybuilding-style training enhances glycogen content by up to 112%. 8. Bone Adaptations: ○ Resistance training above a certain threshold stimulates new bone formation (osteogenesis), especially with heavy loads and multi-joint exercises. ○ Bone adaptations take approximately 6 months to show, beginning within the first few workouts. 9. Connective Tissue Adaptations: ○ Tendons, ligaments, and fascia increase in strength and load-bearing capacity due to resistance training. ○ Structural changes include increased collagen fibril diameter, cross-links, number of fibrils, and packing density. 10.Cartilage and Joint Health: ○ Cartilage may undergo atrophy without loading, but appropriate progressive loading does not seem to cause degenerative joint disease. 11.Body Composition: ○ Resistance training can reduce body fat by up to 9% and increase lean muscle mass, daily metabolic rate, and energy expenditure during exercise. 2.8 CVS Response to Exercise This lecture explores the cardiopulmonary responses to exercise, focusing on both anaerobic and aerobic training. Anaerobic exercise, like resistance training, leads to acute increases in cardiac output, stroke volume, heart rate, oxygen uptake, systolic blood pressure, and blood flow to active muscles. However, chronic resistance training reduces the cardiovascular response to exercise. The lecture also discusses the ventilatory responses during anaerobic exercise, noting that ventilation is elevated during resistance exercise but generally does not limit performance. In contrast, aerobic exercise significantly impacts oxygen uptake, systolic blood pressure, and ventilation, with adaptations in cardiac function, such as increased stroke volume and reduced heart rate. Chronic aerobic training results in enhanced cardiovascular function, improved oxygen delivery, and increased maximal oxygen uptake, which is a key measure of cardiorespiratory fitness. The lecture also highlights specific ventilatory adaptations related to exercise type and the muscles involved, emphasizing the importance of aerobic training for improving endurance and overall cardiovascular Key Points 1. Anaerobic Training: ○ Acute anaerobic exercise (e.g., resistance training) increases: Cardiac output, stroke volume, heart rate, oxygen uptake, systolic blood pressure, blood flow to active muscles. ○ Chronic resistance training reduces cardiovascular response to acute resistance exercise at a given intensity. ○ Short-term studies show resistance training reduces acute increases in heart rate and blood pressure. ○ Oxygen extraction is not significantly improved with heavy, low-volume resistance training but is enhanced with continuous aerobic exercise or high-volume resistance training with short rest periods. 2. Ventilatory Response to Anaerobic Exercise: ○ Ventilation rate does not limit resistance exercise; it is either unaffected or moderately improved by anaerobic training. ○ Ventilation is significantly elevated during each set, especially during the first minute of recovery. ○ Training adaptations include: Increased tidal volume and breathing frequency with maximal exercise. Reduced breathing frequency and increased tidal volume with submaximal exercise. Ventilatory adaptations result from local neural and chemical changes in the muscles trained. 3. Cardiovascular Responses to Aerobic Exercise: ○ Primary Functions: Deliver oxygen and nutrients to muscles, remove waste products. Increases: cardiac output, stroke volume, heart rate, oxygen uptake, systolic blood pressure, blood flow to muscles, and ventilation. Diastolic blood pressure remains stable or decreases slightly. ○ Cardiac Output: Amount of blood pumped per minute (heart rate x stroke volume). Stroke volume increases until oxygen consumption is 40-50% of maximum, then plateaus. ○ Heart Rate: Increases linearly with exercise intensity. Anticipatory increase due to sympathetic nervous system stimulation before exercise. Maximal heart rate depends on age, fitness, and exercise workload. ○ Oxygen Uptake: Increases with exercise intensity; related to muscle mass, metabolic efficiency, and intensity. Maximal oxygen uptake correlates with physical conditioning and is a key measure of cardiorespiratory fitness. ○ Systolic vs. Diastolic Blood Pressure: Systolic pressure increases during exercise, correlating with myocardial oxygen consumption. Diastolic pressure remains stable or decreases due to vasodilation. ○ Ventilation: Minute ventilation increases during aerobic exercise due to deeper, more frequent breaths. 4. Chronic Cardiovascular and Respiratory Responses to Aerobic Training: ○ Cardiovascular Adaptations: Increased maximal cardiac output, stroke volume. Reduced resting and submaximal heart rates. Enhanced muscle fiber capillary density for better oxygen delivery and carbon dioxide removal. ○ Maximal Oxygen Uptake: Improved by increasing central cardiovascular function (cardiac output). Aerobic training reduces the discharge rate of the heart's node, increasing parasympathetic tone and stroke volume. ○ Heart Rate: Resting heart rate slows as stroke volume increases, requiring fewer contractions for the same cardiac output. ○ Ventilatory Adaptations: Do not limit aerobic exercise and are specific to the exercise type (e.g., lower extremity training impacts ventilatory adaptations during lower-body exercises). Increased tidal volume and breathing frequency with maximal exercise. Decreased breathing frequency and increased tidal volume with submaximal exercise. Adaptations are due to local neural and chemical changes in the muscles trained. 2.9 Intro to Pain The lecture on pain highlights its complexity as both a sensory and emotional experience unique to each individual. It emphasizes the challenge of defining and treating pain due to its subjective nature and varying causes, such as tissue damage or even in the absence of it. Chronic pain, affecting a significant portion of the population, is a leading cause for medical visits, and often results in limitations in daily activities. Pain is influenced by biological, psychological, and social factors, and its experience involves sensory perception, emotional responses, and cognitive interpretations. The lecture distinguishes between acute and chronic pain, with the latter becoming maladaptive and requiring interdisciplinary treatment. It also covers various types of pain, including nociceptive, neuropathic, and nociplastic pain, each with different underlying mechanisms and treatment responses. The lecture concludes by explaining how pain can impact the motor system, leading to muscle dysfunction and disability, and stresses the importance of understanding pain mechanisms for effective treatment. Key Points 1. Pain as a Complex Experience: ○ Pain is unique to each individual and difficult to define and treat. ○ Can result from tissue damage or occur without damage. 2. Prevalence of Pain: ○ Pain is the leading cause for seeking medical attention. ○ 100 million adults in the U.S. suffer from chronic pain, greater than diabetes, cancer, and heart disease combined. ○ 30% of the U.S. population has chronic pain lasting over 6 months. ○ 30-60% of those with acute pain have significant limitations in daily activities. 3. Types and Incidence of Pain: ○ Most common: Low back pain (28%), neck pain, migraines, peripheral joint pain. ○ Women experience higher incidence, especially musculoskeletal pain and widespread pain. ○ Pain incidence increases with age. 4. Pain and Nociception: ○ Pain and nociception are not the same. Pain cannot be inferred solely from nociceptor activity. ○ Pain is influenced by biological, psychological, and social factors. ○ Patient reports of pain should be respected, even if they cannot communicate effectively. 5. International Definition of Pain: ○ Pain is an unpleasant sensory or emotional experience associated with potential or actual tissue damage. 6. Dimensions of Pain: ○ Sensory Discriminative: Includes location, quality (sharp, dull, etc.), intensity, and duration. ○ Motivational Affective: Refers to how bothersome the pain is (e.g., nausea or discomfort). ○ Cognitive Evaluative: Includes past experiences and beliefs, influencing pain perception. 7. Acute vs. Chronic Pain: ○ Acute Pain: Result of tissue damage, protective in nature. Often treated with pharmacological and non-pharmacological methods. ○ Chronic Pain: Lasts beyond normal tissue healing time, does not serve a biological purpose, and is more difficult to treat. ○ Chronic pain may become centralized, resulting in a lower pain threshold (allodynia, hyperalgesia). 8. Types of Pain: ○ Nociceptive Pain: Arises from tissue injury (e.g., ankle sprain). ○ Neuropathic Pain: Caused by nerve damage (e.g., carpal tunnel, diabetes). ○ Nocioplastic Pain: Results from central nervous system alterations (e.g., fibromyalgia). 9. Sensitization in Pain: ○ Peripheral Sensitization: Increased nociceptor activity leading to pain. ○ Central Sensitization: Lowered pain threshold due to central nervous system changes, leading to allodynia or hyperalgesia. ○ Referred Pain: Pain felt in areas distant from the injury, often following dermatome or spinal segment patterns (e.g., heart attack pain radiating to the arm). 10.Other Pain Terminology: ○ Hyperalgesia: Increased sensitivity to stimuli. Primary hyperalgesia: At the site of injury. Secondary hyperalgesia: Outside the injury site. ○ Hypoalgesia: Decreased sensitivity to stimuli. ○ Allodynia: Pain from non-painful stimuli (e.g., sunburn pain from brushing the skin). 11.Treatment Considerations: ○ Understanding the underlying pain mechanisms (nociceptive, neuropathic, nocioplastic) guides treatment. ○ Sensitization may require different treatment approaches for peripheral versus central mechanisms. 12.Impact of Pain on Movement: ○ Pain can lead to muscle contraction, inhibition, and fear avoidance behaviors, contributing to disability. 13.Conclusion: ○ Pain is a multifaceted condition that varies across individuals and requires a tailored approach for effective management. 2.10 Intro to Pain Mechanisms and Theories This lecture provides an overview of pain mechanisms and theories. It outlines three primary pain mechanisms: nociceptive pain (due to nociceptor activation), nocioplastic pain (resulting from central pain disturbances), and neuropathic pain (linked to somatosensory system issues). Theories of pain include the specificity theory, which fails to explain phenomena like phantom limb pain, and the gate control theory, which integrates physiological and psychological components, suggesting pain modulation occurs in the spinal cord. Despite criticisms, the theory influenced treatments like TENS. The neuromatrix theory, evolving from the gate control theory, proposes a network of brain structures that generates a personalized pain response based on sensory inputs and cognitive processes. Pain is processed at the cortical level but modified by nociceptors, brain structures, and systemic factors such as hormones and immune cells. The body self neuromatrix interacts with various systems to influence pain perception and response, explaining pain persistence even in the absence of noxious stimuli. Key Points 1. Main Pain Mechanisms: ○ Nociceptive Pain: Caused by activation of nociceptors. ○ Nocioplastic Pain: Due to disturbance in central pain processing (central sensitization). ○ Neuropathic Pain: Results from problems with the somatosensory system. ○ Psychosocial Pain: Involves social or psychological factors contributing to pain. 2. Early Theories of Pain: ○ Specificity Theory: Assumes separate nerve endings for different sensations (cold, touch, warmth, pain). Pain receptors are activated only by painful stimuli. Limitations: Cannot explain phenomena like phantom limb pain or allodynia (pain from non-painful stimuli). Central lesions do not eliminate pain. ○ Gate Control Theory (Melzack & Wall, 1965): Integrated Model: Combines physiological and psychological aspects of pain. Nociceptors and large diameter afferents converge in the spinal cord's dorsal horn (substantia gelatinosa, SG). Key Concepts: SG neurons inhibit T cells that initiate pain responses (motor, sensory, autonomic). Pain occurs when nociceptors inhibit SG neurons, allowing T cells to fire. Increased input from large diameter fibers can close the gate and reduce pain. The brain can modulate this pain process through central control. ○ Applications of Gate Control Theory: Explains phenomena like: Pain persistence after nociceptor removal (e.g., phantom limb pain). Increasing pain with repetitive noxious stimuli. Use of TENS to increase large diameter input and inhibit pain. ○ Criticisms of Gate Control Theory: Nociceptors: Not tonically active; they respond to noxious stimuli. SG Interaction: Nociceptors are excitatory, not inhibitory to SG. Neurons: Some SG neurons only respond to noxious stimuli and don't receive input from large fibers. Presynaptic Inhibition: The theory overemphasized presynaptic inhibition, but postsynaptic inhibition also plays a role. Central Modulation: CNS both facilitates and inhibits pain at the spinal cord level. ○ Clinical Use of Gate Control Theory: Influenced treatments like TENS, designed to modulate pain through spinal cord input. 3. Evolution of Pain Theories: ○ Neuromatrix Theory (Melzack, 1991): Proposes a widespread network of neurons integrating the thalamus, cortex, and limbic system. The "neurosignature" is a unique pattern of output that includes pain awareness and motor output. The neurosignature is plastic, changing based on sensory inputs and cognitive events. ○ Pain Processing: Pain is processed in the brain at the cortical level, where awareness occurs. Activation of nociceptors can maintain pain, influenced by spinal and subcortical brain structures. Other systems like immune cells, hormones (e.g., cortisol, estradiol), and muscle factors (ATP, lactate) also influence pain. ○ Body Self Neuromatrix: Pain perception is influenced by: Direct input from nociceptors. Cognitive and evaluative processes in the cortex. Systemic factors (immune cells, hormones). Outputs include pain perception, stress responses, and actions in response to pain. 4. Conclusion: ○ Pain results from a complex network of interactions between sensory inputs, brain structures, and systemic factors. ○ Pain perception and response can persist even without noxious stimuli due to the plasticity of the neuromatrix and the brain's role in modulating pain. 2.11 Intro to Pain Assessment The lecture on pain assessment emphasizes the importance of accurately evaluating pain to guide treatment decisions. It stresses that pain assessment should be done regularly, alongside vital signs, and that it provides critical data for diagnosis, therapy, and progress evaluation. Pain assessments should include details on location, intensity, duration, and frequency, with a focus on understanding the patient's personal experience. The lecture highlights various pain assessment tools, including unidimensional (focusing on pain intensity) and multidimensional scales (assessing multiple constructs), and emphasizes the value of self-reporting for pain measurement, especially in adults and older children. For individuals who cannot self-report, behavioral and physiological indicators are used, though these are less reliable. Common tools include numerical rating scales, visual analogue scales, and face scales, which help gauge pain intensity and its impact on daily function. The lecture also discusses the significance of pain location and how widespread pain can correlate with greater disability. Key Points 1. Objectives of Pain Assessment: ○ Provide accurate data for determining appropriate treatment. ○ First step in effective pain management. ○ Includes assessing nature of pain, physiologic, behavioral, emotional responses, and previous pain experiences. 2. Pain as a Vital Sign: ○ Recognized as a fifth vital sign by the American Pain Society and the Joint Commission. ○ Pain should be assessed with every vital sign check. 3. Importance of Pain Assessment: ○ Crucial for diagnosis, guiding therapy, and evaluating treatment progress. ○ Requires age-appropriate tools and scales for accurate assessment. 4. Routine Monitoring: ○ Pain should be monitored, assessed, reassessed, and documented. ○ Helps facilitate treatment and communication among healthcare providers. ○ Valid and reliable pain measurement needed for identifying patients requiring intervention and evaluating therapy effectiveness. 5. Pain Characteristics to Assess: ○ Location: Multiple locations or a single area? ○ Description: Terms used by the patient (e.g., ache, sharpness, etc.). ○ Intensity: How severe is the pain? ○ Duration and Frequency: Duration of pain and frequency of occurrences. ○ Pain Fluctuations: Questions about worst, average, and least pain over a certain period. 6. Pain Recall: ○ Short-term recall of pain intensity is generally accurate, especially for the average pain experienced in the last 24 hours or week. ○ Long-term memory of pain intensity is less accurate, while recall of pain-reduced activities and pain location is generally good. ○ People often overestimate pain intensity compared to actual measurements. 7. Pain Intensity Influencers: ○ Memory of pain is influenced by factors such as the intensity of painful procedures, emotions, expectations, and peak pain intensity. 8. Pain Measurement Tools: ○ Should have well-established reliability and validity. ○ Assessment tools vary depending on whether the pain is acute or chronic and the therapist's practice setting. ○ Two main types: unidimensional (measures one construct like pain intensity) and multidimensional (measures various constructs). 9. Pain Measurement Classifications: ○ Self-report: Gold standard, preferred method. ○ Behavioral or Observational: Used when the patient cannot self-report. ○ Physiological: Used to measure physiological responses (e.g., heart rate, blood pressure). 10.Self-Report Tools: ○ Verbal: Structured interviews, questionnaires, and self-rating scales. ○ Nonverbal: Includes facial expression scales, visual analog scales (VAS), and drawings. ○ Pain Intensity Rating: Numerical rating scale (0–10), VAS, and face scales are commonly used. 11.Behavioral Observation: ○ Used for individuals who cannot self-report, such as infants, young children, or those with cognitive impairments. ○ Behavioral checklists and rating scales assess vocal, facial, postural, and motor behaviors. 12.Physiological Parameters: ○ Includes heart rate, blood pressure, pupil dilation, nausea, oxygen saturation, etc. ○ Less reliable for long-term pain but useful in acute pain stages. ○ Should be used as complementary tools alongside behavioral observations. 13.Pain Intensity Scales: ○ Numerical Rating Scale (NRS): Simple, quick, and reliable; rates from 0 (no pain) to 10 (worst pain). ○ Visual Analog Scale (VAS): A 10 cm line where patients mark their pain intensity. ○ Global Rating Scale: Provides a rating of pain intensity. ○ Body Diagram: Patients draw the location of their pain, useful for understanding pain distribution. 14.Pain Area and Disability: ○ Understanding the widespread nature of pain is essential in pain management. ○ More areas of pain are linked to higher disability and greater difficulty with physical functions, social activities, and mood. 15.Psychometric Properties of Pain Tools: ○ Pain measurement scales should be reliable, easy to use, and responsive to both pharmacological and non-pharmacological interventions. 2.12 Intro to Pain Questionnaires The lecture focuses on the use of various pain assessment questionnaires, highlighting their role in understanding and managing pain. It introduces three key psychological dimensions of pain: sensory-discriminative, affective-motivational, and evaluative-cognitive, which form the basis for tools like the McGill Pain Questionnaire. This questionnaire assesses the sensory, emotional, and evaluative aspects of pain through a body diagram, pain descriptors, and intensity ratings. The lecture also covers the Brief Pain Inventory, which measures pain severity and interference with daily activities, and the PainDetect Questionnaire, designed to identify neuropathic pain. It discusses the psychological factors that influence pain, such as depression, anxiety, and fear-avoidance, and the importance of recognizing these factors to improve treatment outcomes. The Fear-Avoidance Beliefs Questionnaire (FABQ) and the Pain Catastrophizing Scale are presented as tools to evaluate psychological responses to pain. Lastly, self-efficacy scales like the Chronic Pain Self-Efficacy Scale are discussed for understanding how pain affects patients' confidence in managing daily activities. Key Points Pain Assessment Questionnaires: Key Points 1. Psychological Dimensions of Pain: ○ Sensory-Discriminative: Identifies the location, intensity, and quality of pain. ○ Affective-Motivational: Relates to the emotional response to pain. ○ Evaluative-Cognitive: Involves the individual's assessment and interpretation of pain. 2. McGill Pain Questionnaire (MPQ): ○ Developed to assess the multidimensional aspects of pain experience. ○ Structure: Body Diagram: Patients mark the location of their pain. Pain Descriptors: 78 words across 20 subclasses, allowing selection of words that best describe their pain. Pain Intensity: A single measure ranging from 1 to 5. ○ Scoring: Pain Rating Index (PRI): Sum of rank values of chosen words. Present Pain Intensity (PPI): Single intensity score. ○ Validity: Demonstrated to be a valid, objective, and reliable instrument. 3. Brief Pain Inventory (BPI): ○ Assesses the functional impact of pain. ○ Structure: Pain Severity: Four visual analog scales (VAS) for pain severity. Pain Interference: Measures interference with general activity, mood, walking, work, relationships, sleep, and enjoyment of life. ○ Scoring: Pain Intensity Score: Sum of severity items. Pain Interference Score: Sum of interference items. 4. PainDetect Questionnaire: ○ Detects neuropathic pain components in low back pain patients. ○ Structure: Seven questions assessing pain quality, course, and radiation. ○ Scoring: Total score ranges from -1 to 35. Scores ≤12: Unlikely neuropathic component. Scores ≥19: Probable neuropathic component. 5. Psychological Factors Influencing Pain Perception: ○ Depression and Anxiety: Associated with increased pain perception. ○ Fear-Avoidance Beliefs: Fear of pain leading to activity avoidance. ○ Pain Catastrophizing: Negative cognitive response to pain. ○ Low Self-Efficacy: Reduced confidence in managing pain. 6. Fear-Avoidance Beliefs Questionnaire (FABQ): ○ Assesses beliefs about how physical activity and work affect current pain. ○ Structure: 16 items focusing on physical activity and work. ○ Scoring: Higher scores indicate greater fear-avoidance beliefs. 7. Pain Catastrophizing Scale (PCS): ○ Measures negative cognitive and emotional responses to pain. ○ Structure: 13 items rated on a 5-point scale. ○ Scoring: Total score reflects overall catastrophizing tendency. 8. Chronic Pain Self-Efficacy Scale (CPSS): ○ Evaluates confidence in managing chronic pain. ○ Structure: 22 items across three domains: pain management, physical functioning, and coping with symptoms. ○ Scoring: Higher scores indicate greater self-efficacy. 9. Pain Self-Efficacy Questionnaire (PSEQ): ○ Assesses confidence in performing activities despite pain. ○ Structure: 10 items rated on a 7-point scale. ○ Scoring: Total score reflects overall self-efficacy. 10.Clinical Application: ○ Identifying psychological factors can guide treatment plans. ○ Incorporating psychological assessments aids in comprehensive pain management 2.13 ROM The lecture focused on the concept of range of motion (ROM) as a fundamental technique in physical therapy for assessing and improving movement. ROM activities are categorized into three types: passive (PROM), active (AROM), and active assistive (AAROM). PROM involves external forces moving a joint, while AROM is initiated by muscle contraction, and AAROM combines both. ROM helps maintain joint and soft tissue mobility, prevents contractures, and promotes healing, but it must be carefully managed based on the patient’s condition, including any contraindications. The therapist needs to assess the patient's ability, choose the appropriate ROM type, and monitor progress, using tools like CPM machines and mechanical aids to assist when necessary. Key Points 1. Range of Motion (ROM) Overview: ○ ROM Definition: Range of motion (ROM) is a technique used to examine and initiate movement as a therapeutic intervention. It involves muscles or external forces moving bones through various patterns or ranges of motion. ○ Control of Motion: The central nervous system controls muscle activity to produce movement. ○ Affected Structures: ROM affects muscles, joints, synovial fluid, joint capsules, ligaments, fascia, vessels, and nerves. ○ Joint vs. Muscle ROM: ROM is typically described in terms of joint range (e.g., flexion, extension, abduction) and muscle range, measured with a goniometer. 2. Types of ROM: ○ Passive Range of Motion (PROM): External force moves the segment within its unrestricted range. No voluntary muscle contraction by the patient (force could be from gravity, machines, or another person). Indications: Acute tissue inflammation, inability to move a segment (e.g., coma, paralysis), post-surgical situations where active motion would be harmful (e.g., after muscle repair). Primary Goals: Decrease complications from immobilization, maintain joint mobility, enhance circulation, assist with healing, prevent contractures. Limitations: Doesn't prevent muscle atrophy, doesn't improve strength/endurance, doesn't assist circulation as well as active muscle contractions. ○ Active Range of Motion (AROM): Movement produced by the active contraction of muscles crossing a joint. Indications: When the patient can actively move a segment, aerobic conditioning, or preventing circulatory issues in immobilized body parts. Benefits: Maintains muscle elasticity, improves circulation, enhances motor learning, prevents thrombus formation. Limitations: Doesn't build strength in strong muscles, doesn't develop coordination unless specific to the movement pattern. ○ Active Assistive Range of Motion (AAROM): A combination of active contraction and external assistance (manual or mechanical). Indications: When a patient needs help to move a joint through a range due to weak muscles. 3. Therapeutic Goals for ROM: ○ Maintain joint and connective tissue mobility. ○ Minimize contracture formation. ○ Maintain muscle elasticity and assist in circulation. ○ Enhance synovial movement for cartilage nutrition. ○ Decrease pain, assist in the healing process, and improve awareness of movement. 4. Contraindications & Considerations: ○ ROM may be contraindicated in situations where movement could disrupt healing, cause excessive pain, or is life-threatening. ○ Precautions: Always monitor patient responses during ROM exercises (e.g., pain, inflammation, vitals). ○ Communication: It is essential to communicate with physicians, especially regarding the appropriateness of ROM techniques. 5. ROM Application Techniques: ○ Preparation: Communicate with the patient, ensure comfort, remove restrictions (clothing, splints), align the patient correctly, and apply good body mechanics. ○ Control of Movement: Gently grasp extremities, avoid forcing motions (especially in painful joints), and move smoothly through the pain-free range. ○ Repetitions: Typically 5-10 repetitions, depending on goals. ○ ROM Equipment: ○ Self-Assistive ROM: Patients can use unaffected extremities or assistive devices (e.g., skateboards, powder boards, towel slides) for movement. ○ Other Equipment: Wands, finger ladders, pulleys, and continuous passive motion (CPM) machines. CPM machines can be used post-surgery to move a joint, but there is debate over their effectiveness, especially after knee replacement surgeries. 6. Key Considerations: ○ Tailor ROM techniques based on the patient’s condition, impairments, and recovery stage. ○ ROM can be performed in different planes of motion (frontal, sagittal, transverse) and can include combined or functional patterns. ○ Always monitor and adjust based on the patient's progress and responses during ROM activities.