Force, Torque and Power Measurements PDF
Document Details
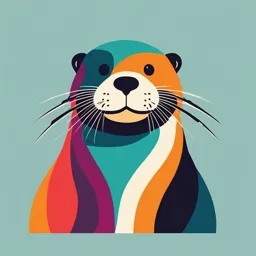
Uploaded by GratifiedAstatine6644
Tags
Summary
This document provides an overview of force, torque, and power measurements. It details various methods for measuring these parameters, including direct and indirect methods. Examples include using physical balances, elastic members, and magnetic forces.
Full Transcript
Force, Torque and Power Measurements 1 Force Force is a vector A force can accelerate/decelerate objects by pulling or ✔ The SI unit of Force is in Newtons (N). 1 Newton N = pushing them....
Force, Torque and Power Measurements 1 Force Force is a vector A force can accelerate/decelerate objects by pulling or ✔ The SI unit of Force is in Newtons (N). 1 Newton N = pushing them. kg x m/s2 The relationship between force, mass, and acceleration is defined by Newton’s second law of motion ✔ The British units of force is the pound-force (lbf) = 4.4482216 N Force = Mass x Acceleration ✔ g = 9.81 m/s2 = 32.2 ft/s2 Weight is the measure of the force of gravity acting on an object, but mass is not affected by gravity. Weight (w) = mass (m) × gravity (g) 2 Force Measurement Methods ❑ Direct Method: This involves a direct comparison with a known gravitational force on a standard mass. Example: Physical Balance ❑ Indirect Method: This involves the measurement of effect of force on a body. e.g. Force causes a certain deflection on an elastic element and the force is calculated from the measured deflection. 3 Force Measurement Methods 1. Balance the unknown force against a known gravitational force caused by a standard mass. Scales and balances are based on this principle 2. Apply the unknown force to an elastic member (spring, beam, cantilever, etc.) and measure the resulting deflection on a calibrated scale 3. Apply the unknown force to a capacitor or a piezo electric transducer and measure the change in capacitance or the resulting charge 4. Translate the unknown force to a fluid pressure and measure the resultant pressure. Hydraulic and pneumatic load cells are based on this principle 5. Apply the unknown force to a known mass and then measure the resulting acceleration. 6. Balance the unknown force against a magnetic force that is developed by interaction of a magnet and current in coil. 4 Balance the unknown force against a known gravitational force https://www.brainkart.com/article/Scale-and-balances-on-Measurement-of-Force_5862/ 5 Equal-arm beam balance scale An equal arm balance can be used to measure the mass or weight of an object. Depends on gravity. When the beam is balanced( horizontal), the force of the known weights equals the weight of the object. Some scales can be calibrated to read in units of force https://www.brainkart.com/article/Scale-and-balances-on-Measurement-of-Force_5862/ (weight) or in units of mass 6 Unequal-arm beam balance scale For null balance: W1b = W2a, hence W2 = W1 (b/a) https://image.invaluable.com/housePhotos/Pacific/94/626694/H0123-L147221929.jpg 7 Sources of errors in balance scales 1. Error in the mass of the reference weights 2. Misaligned mechanical components due to thermal expansion or contraction of components 3. Buoyancy – objects in air develop a buoyancy force that is directly proportional to the volume of air displaced (i.e., proportional to their volumes) 4. Friction in the moving components causes the scale to reach https://www.brainkart.com/article/Scale-and-balances-on-Measurement-of-Force_5862/ equilibrium at a different configuration than with a friction-less equilibrium. 5. Condensation of atmospheric water on cold items/evaporation of water from wet items 6. Chemical reactivity between air and the substance being weighed (or the balance itself, in the form of corrosion) 7. Vibration and air gusts 8 Force Measurements: Force to Displacement Conversion 9 Spring scale Operates based on Hooke’s law Within the elastic range, F = kX, where F is the amount of force applied to the spring, X is the distance travelled, and k is the stiffness of the spring Range can be increased by increasing the stiffness of the spring, but this reduces the resolution. Reads correctly in the frame of reference where the acceleration in the spring is constant (such as on earth, where the acceleration is due to gravity). The reading will not be true in an accelerating/decelerating elevator. F = K. X, where K is the stiffness of spring and x is the deflection 10 Strain-gauge load cell with a Wheatstone-Bridge Circuit A strain gauge load cell is made up of 4 strain gauges in a Wheatstone bridge configuration. The applied force causes deformation the beam 🡪 Length and area of the strain gage changes 🡪 Resistance of the strain gage changes 🡪 Output voltage from the Wheatstone bridge Must operate within the elastic range Requires calibration https://cdn.instrumentationtools.com/wp-content/uploads/2016/05/instrumentationtool s.com_resistive-load-cell-working.png 11 LVDT load cell The force causes a displacement on the elastic diaphragm This displacement is measured using an LVDT The LVDT generates a voltage proportional to the force Must operate within the elastic range Can also measure pressure or acceleration https://www.omega.co.uk/prodinfo/images/LVDT-pressure.jpg 12 Proving Rings It is a ring of known physical dimensions & mechanical properties. An external tensile or compressive force causes a proportional distortion. A displacement sensor measures the deflection and converts the reading into force Must operate within the elastic range Used as a calibration standard for large tensile-testing machines. https://www.indiamart.com/proddetail/proving-rings-4059389488.html 13 Force Measurements: Force to Capacitance Conversion 14 Capacitive Load Cells Capacitive load cells work on the principle of capacitance, which is the ability of a system to store a charge. The load cell is made up of two flat plates parallel to each other. The plates will have a current applied to them and once the charge is stable it gets stored between the plates. https://realpars.com/load-cell/ The amount of charge stored, the capacitance, depends on how large of a gap between the plates. When a load is placed on the plate the gap shrinks giving us a change in the capacitance which can be calculated into a weight. 15 Force Measurements: Force to Charge Conversion 16 Force Measurements: Piezoelectric force transducer ❑ A piezoelectric material produces an electrical charge between the opposite faces of a crystal when the crystal is deformed by a force that makes them suitable for use as a force sensor. This signal is proportional to the force acting on the material. ❑ Many crystals exhibit the piezoelectric effect. Some common crystals are: - Quartz - Rochelle salt - Lithium Sulphate - Tourmaline 17 Force to Fluid Pressure Conversion 18 Pneumatic/Hydraulic Load Cells When a load or force is placed on the piston or diaphragm, it generates a pressure to the fluid inside it. The increase in the fluid pressure is proportional to the applied force. Must operate within the elastic range. Calibration relates the applied force to the pressure on F = PA the gage. The generated pressure can be translated into an electric signal. When no electric components are used, the cell is not affected by transient voltages as lightning. Measure forces in the range 0 to 2.5 MN Accuracy 0.1% of full scale https://realpars.com/load-cell/ 19 Accuracy of Different Load Cells https://realpars.com/load-cell/ 20 Force Measurements Example #3: Example #1: When a container is placed on a spring balance with What force is required to accelerate a mass of 27 kg at 18 an elongation constant of (11.6 N/cm), the spring m/s2? stretches (8.1 cm). What is the weight of the container? Force = 27 × 18 N = 486 N Weight = 11.6 N/cm × 8.1 cm = 93.96 N Example #2: What is the mass of a block of metal that weighs 130 N? Example #4: What is the force acting on a 35.6-cm diameter piston, if the Mass = 130/9.81 = 13.25 kg pressure gage reads 152 kPa? 21 Torque Measurements 22 Torque Measurements Torque is the tendency of a force to rotate the body on which it acts Torque is a moment vector Torque is the cross product between the radius (r) vector and the force vector (F) and: T = R X F Strain gauges are used to measure the force and then to estimate the torque T = FR 23 Torque versus Brake Power Brake Power = Torque * 2π N/60, where N = Speed in RPM and T is the torque in N.m Brake power is a measure of power (not work) Brake power can only be calculated if torque and RPM are known If there is no motion, no power is done. Because of this, the engine must be rotating to create brake power. Torque exists even if there is no rotation (RPM = 0) Brake power is zero if the RPM is zero 24 Torque versus Brake Power Brake power is a VECTOR, meaning that it has both magnitude and direction. Direction in this case is rotary. If there is no motion, no power is done. Electric motors can produce torque at zero rpm. Internal combustion engines can’t because they won’t run at low rpm. Torque measurement is usually associated with the determination of brake power, either power required to operate a machine or to find out the power developed by the machine. In ICEs, the torque varies with the RPM (N) Example of the output curves 25 Torque Meters Measures the torque on a rotating system, such as an engine, a crank-shaft, or a gear-box There are two main categories of Torque Meters: rotary torque sensor and reaction torque sensors. Reaction torque meters measure stationary torque (static or non-rotational) Rotary torque meters measures rotational torque (dynamic torque sensor). For a given application, a reaction sensor (aka moment sensor) is often less complex and, therefore less expensive than a rotary sensor Static torque is relatively easier to measure than to dynamic torque Dynamic torque requires transfer of some effect (electric, hydraulic or magnetic) from the shaft being measured to a static system. 26 Reaction Torque Meters A reaction torque meter has two mounting flanges (flange-to-flange sensor). One face is fixed to a rigid structural member and the other to the rotating shaft or rotary element. Rotation generates shear forces between the flanges, which is captured by the foil strain gauges bonded to the sensor beams and transduced into voltage by the Wheatstone bridge. Using a cylindrical elastic member, either the deflection or the strain measurement may be used to determine the applied torque. Reactionary torque transducers are frequently used as a torque calibration tool or torque wrench calibration tool. https://www.futek.com/torque-meter Reaction torque sensor https://www.sensel-measurement.fr/en/static-torque-sensor/1218-smrx -reaction-torque-sensor-with-flanges-50-to-5000-nm.html https://www.futek.com/torque-meter 27 https://www.researchgate.net/profile/Thomas-Povey/publication/230902182/figure/fig1/AS:300574265364481@1448673897827/a-Wheatstone-bridge-circuit-b-strain-gauge-mounting-for-torque-measurements.png 28 Measurements of Brake Power 29 Measurements of Brake Power ✔ One Horsepower is the amount of power needed to lift 75 kg for 1 meter of height in a time period of 1 second ✔ One metric horsepower equals 735 Watt ✔ 1 Watt = 1 joule/s 30 30 Dynamometer A dynamometer is a device used for measuring the power transmitted by a rotating shaft of various equipment, such as diesel engines, gasoline engines, gas turbines, hydraulic turbines, electric motors, etc… Measures the torque and the brake power developed by an engine under certain conditions (inlet air temperature and pressure, coolant temperature, oil temperature, humidity, etc…), or required to operate a certain device (pump or compressor) Used over a range of engine speeds to obtain engine power and torque curves A dynamometer for testing engines must include all the following four elements: Example of the output curves 1. A means for controlling the torque 2. A means for measuring the torque 3. A means for measuring the rotational speed 4. A means for dissipating the output brake power 31 31 Dynamometers can be broadly classified into two types Absorption dynamometers: Measure power or torque developed by power sources such as engines or electric motors. The power absorbed is usually dissipated as heat by some means. Examples are Prony brake dynamometer, Rope brake dynamometer, Eddy current dynamometer, Hydraulic dynamometer, etc… https://www.engineeringclicks.com/dynamometer/ Driving dynamometers: Measure power or torque and also provide energy to operate the device to be tested. Useful for determining the performance characteristics of devices such as pumps and compressors 32 32 Careful with the inlet air temperature and pressure The measured brake power is proportional to the inlet air density (and hence inlet temperature and pressure) https://www.engineeringclicks.com/dynamometer/ 33 33 Prony Brake Dynamometer One of the simplest dynamometers for measuring brake output power It works on the principle of converting power into heat by dry friction The dynamometer attempts to stop the engine by means of a brake on the flywheel and measure the weight which an arm attached to the brake will support, as it tries to rotate with the flywheel. 34 Hydraulic Dynamometer The working medium, usually water, is circulated within the housing creating frictional resistance to the shaft rotation. The engine power is converted to heat and the cooling liquid exits at a higher temperature than its inlet temperature. The casing, which is free to rotate around the input shaft, is restrained by a torque arm. Measurements of https://www.youtube.com/watch?v=1-gTFnC92ug that torque allows estimation of the brake power. The heat developed is carried away by a continuous supply of the cooling fluid The output power is controlled by regulating the channel gates which can be moved in and out to partially or wholly obstruct the flow of water between the casing and the rotating shaft. The capacity of hydraulic dynamometer is a function of speed and the water level 35 Hydraulic Dynamometer 36 Eddy Current Dynamometer When the current flows through the field coils, magnetic field is produced. If the rotor is rotated in the field, the magnetic flux changes and eddy current are produced and they buildup an opposite magnetic field and decelerate the rotor. The braking torque is transferred via the bearing mounted body to the load cell. The dynamometer load is regulated by changing the excitation current. 37 Eddy Current Dynamometer Precision Strain Gauge type Load Cell are used for sensing the Torque developed Rugged and rigid design lead to long working life. Compact design Parts in contact with cooling water are coated with nickel Dry running is avoided by water flow switch. Bi-directional operation Smooth running of Rotor Easy of assembly, wide power range, disc rotor and hence low inertia, very high response to change in input signals, long life uses and long servicing intervals, easy maintenance due to less components, low downtime due to simple mechanical design. High brake power per unit weight of dynamometer. Easy to control and operate. Development of eddy current is smooth hence the torque is also smooth and continuous under all conditions. No natural limit to size, either small or large. 38 Measurements of RPM 39 Mechanical Device: Tachometer The tachometer must be coupled mechanically to the rotating shaft 🡪 The rotary motion is either transmitted by friction or by a gear arrangement. The tachometer employs a small dc permanent-magnet generator to generate a voltage proportional to the rotational speed. The device may be attached permanently to the shaft for continuous monitoring of speed, or connected by friction to measure speed when needed. 40 ii. Non contact optical rpm meter: a)LED photo detector wheel This is a non contact method of rotational speed of a shaft. However it requires a wheel with openings to be mounted on the rotating shaft. The frequency of interruption of the beam is directly proportional to the rpm of the wheel, that is usually the same as the rpm of the shaft to be measured and the number of holes provided along the periphery of the wheel. If there is only one hole the beam is interrupted once every revolution. If there are n holes the beam is interrupted n times per revolution. The rotational speed of the shaft is thus equal to the frequency of interruptions divided by the number of holes in the wheel. https://www.youtube.com/watch?v=HksPmdwazT0 41 ii. Non contact optical rpm meter: b) stroboscope A strobe light is flashed on the rotating shaft or object. A marking on the object itself will appear stationary when the strobe frequency coincides with the rotational speed (frequency). A reading of strobe frequency then determines the angular speed. You must be careful with aliasing https://www.youtube.com/watch?v=KPrSPqfVJhA 42 Flow Rate https://www.tec-science.com/wp-content/uploads/2020/04/en-gases-liquids-fluid-mechanics-hagen-poiseuille-pipe-flow-pressure-drive.png Measurements https://forumautomation.com/uploads/default/original/2X/f/f11fd8a79b4f98d570bda94c783ddf29822df115.png 1 Flow Rate Measurements Obstruction Flow Meters: ▪ Orifice meter ▪ Flow nozzle Q ~ SQRT (P1-P2) ▪ Venturi-meter Laminar flow meter Q ~ (P1-P2) Variable-area flow meter (Rotameter) Q ~ f(float position) Turbine/paddle-wheel flow meter Q ~ f(RPM) Vortex-shedding flow meter Q ~ f(frequency of vortex shedding) Ultrasonic flow meter Q ~ f(transit time) Positive-displacement flow meter Q ~ f(number of turns) Selection criteria Calibration of flow meters 2 Obstruction Flow Meters: Orifice Meters https://neutrium.net/images/fluid-flow/orifice-vena-contracta.png 3 Obstruction Flow Meters: Orifice Meters https://www.automation.com/getmedia/debdcbb6-ec1e-4967-9378-4 b2b9e93c44b/R2.jpg?width=500&height=417 4 Obstruction Flow Meters: Orifice Meters https://www.researchgate.net/profile/Elhadi-Dekam/publication/331438263/figure/fig1/AS:731632315146241@1551446145292/An-orific e-plate-flow-meter-with-vena-contracta.jpg 5 Vena Contracta Fluid Mechanics: Fundamentals And Applications 3rd edition, Yunus Cengel, John Cimbala 6 What is Vena Contracta https://2.bp.blogspot.com/-HfgnHmxdnOQ/W_kx6VpZBWI/AAAAAAAABe4/QGjwgnGieg46UZmYNURWUZuRPWSduAtpQCLcBGAs/s1600/vena%2Bcontracta.png 7 What is Vena Contracta https://qph.cf2.quoracdn.net/main-qimg-8d2c55761e0b1742ab9243b690e41fc3 8 Obstruction Flow Meters Orifice Meter Q ~ SQRT (P1-P2) There is a large permeant loss of pressure at the downstream side Loading effect 9 Obstruction Flow Meters: Three Types Cd ~ 0.60 Cd ~ 0.98 Cd ~ 0.96 https://instrumentationforum.com/uploads/db4532/original/2X/c/c3c99904f9977be894d a2e026374129710abf351.png https://eligoprojects.com/wp-content/uploads/2018/10/venturimeter-construction.png 10 Obstruction Flow Meters: Flow Nozzle The obstruction is rounded on the upstream side as sketched to the right 🡪 more aerodynamic shape Cd ~ 0.96 The flow is more efficiently guided through the opening, and thus the discharge coefficient (~0.96) is much larger than that of an orifice meter (~0.60) https://instrumentationforum.com/uploads/db4532/original/2X/c/c3c99904f9977be894d a2e026374129710abf351.png 11 Obstruction Flow Meters: Venturi Meter Cd ~ 0.98 Flow is efficiently guided on the upstream side AND on the downstream side 🡪 shape closely approximates the streamline pattern 🡪 no boundary layer separation 🡪 the permeant pressure loss is lower than those of orifice meters and flow nozzles 🡪 Larger coefficient of discharge: Cd ~ 0.98 https://instrumentationforum.com/uploads/db4532/original/2X/c/c3c99904f9977be894d a2e026374129710abf351.png https://eligoprojects.com/wp-content/uploads/2018/10/venturimeter-construction.png 12 Obstruction Flow Meters: Streamline patterns Cd ~ 0.98 Cd ~ 0.60 Cd ~ 0.96 https://instrumentationforum.com/uploads/db4532/original/2X/c/c3c99904f9977be894d a2e026374129710abf351.png https://eligoprojects.com/wp-content/uploads/2018/10/venturimeter-construction.png 13 Flow Meters: Obstruction Meters – Governing Equations In reality, some pressure losses caused by the eddies The fluid stream continues to contract past the obstruction, and the vena contracta area is less than the flow area of the obstruction. Both losses can be accounted for by the discharge coefficient Cd Fluid Mechanics: Fundamentals And Applications 3rd whose value is less than one. edition, Yunus Cengel, John Cimbala where A0 = A2 = πd2/4 is the cross-sectional area of the throat or orifice and β = d/D is the ratio of throat diameter to the pipe diameter. Cd = function (β, Re) 14 Flow Meters: Obstruction Meters – Governing Equations A1: pipe/inlet flow area A2: orifice/constriction flow area P1 and P2: Pressure at A1 and A2, respectively https://2.bp.blogspot.com/-Nb7HbZxq9UM/WxzrbBRhdOI/AAAAAAAAETM/IxXqRTpc43cwDJX6X40 Cd: Coefficient of Discharge = f (Reynolds Number and diameter ratio) cyeVicUu7BkzCACLcBGAs/s1600/12.jpg Cd accounts for two factors: 1- the vena contracta area is less than the flow area of the obstruction 2- pressure losses caused by the eddies 15 Obstruction Flow Meters: Three Types Orifice Meters ✔ Inexpensive ✔ Easy to install X Large permanent pressure losses Flow Nozzles X Difficult to install properly ✔ High Accuracy ✔ Good Pressure recovery Venturi Meters X Expensive to construct ✔ Good pressure recovery https://instrumentationforum.com/uploads/db4532/original/2X/c/c3c99904f9977be894d a2e026374129710abf351.png 16 Obstruction Flow Meters: Orifice Meters Advantages: ✔ Requires small space for installation ✔ Easy to install/remove ✔ Can be easily maintained ✔ Measures a wide range of flows ✔ Simple construction ✔ Can easily be fitted between the flanges ✔ Suitable for most gases and liquids ✔ Inexpensive ✔ Price does not increase dramatically with size https://instrumentationforum.com/uploads/db4532/original/2X/c/c3c99904f9977be894d a2e026374129710abf351.png 17 Obstruction Flow Meters: Orifice Meters Limitations: X Can not measure flow reversal X Careful with cavitation X Requires homogeneous fluids X Requires single-phase liquids X Requires the flow of axial velocity vectors. X Causes a large permeant pressure drop X Requires straight conduits to ensure accuracy is maintained X Accuracy is affected by the errors in density, pressure, and the area ratio https://www.flowmeters.com/differential-pressure-for-clean-gas# https://instrumentationforum.com/uploads/db4532/original/2X/c/c3c99904f9977be894d a2e026374129710abf351.png 18 Obstruction Flow Meters: Venturi Meters Advantages: ✔ Less chance of getting stuck with sediment ✔ The discharge coefficient is high (Cd ~0.98) ✔ Its behavior can be predicted perfectly ✔ Can be installed vertically, horizontally or inclined ✔ Can be used for a wide range of flows https://instrumentationforum.com/uploads/db4532/original/2X/c/c3c99904f9977be894d a2e026374129710abf351.png 19 Obstruction Flow Meters: Venturi Meters Limitations: X Can not measure flow reversal X Careful with cavitation X Large in size 🡪 Can not be used where space is limited X Large initial cost X Installation and expensive maintenance X Accurate operation requires a long placement length 🡪 must be driven by a straight tube that has no connections or misalignments upstream and downstream of the meter https://instrumentationforum.com/uploads/db4532/original/2X/c/c3c99904f9977be894d a2e026374129710abf351.png 20 Obstruction Flow Meters: Solved Example 21 Obstruction Flow Meters: Solution 22 Obstruction Flow Meters: Solved Example 23 Flow Rate Measurements Obstruction Flow Meters: ▪ Orifice meter ▪ Flow nozzle Q ~ SQRT (P1-P2) ▪ Venturi-meter Laminar flow meter Q ~ (P1-P2) Variable –area flow meters (Rotameters) Q ~ f(float position) Turbine/paddle-wheel flow meter Q ~ f(RPM) Vortex-shedding flow meter Q ~ f(frequency of vortex shedding) Ultrasonic flow meter Q ~ f(transit time) Positive-displacement flow meters Q ~ f(number of turns) Selection criteria Calibration of flow meters 24 Obstruction Flow Meters: Laminar Flow Element Hagen–Poiseuille equation: Q ~ (P1-P2) Incompressible Newtonian fluid Laminar flow region Fully-developed flow Inside a long cylindrical pipe Constant cross section Q = (P1-P2)πr4/8μL Where: Q: Volumetric flow rate P1: Static pressure at the inlet P2: Static pressure at the outlet r: Hydraulic radius of the restriction μ: Absolute viscosity of the fluid L: Length of the restriction 🡪 Q ~ (P1-P2)/ μ https://www.calctool.org/CALC/eng/fluid/hagen-poiseuille.png https://www.tec-science.com/wp-content/uploads/2020/04/en-gases-liqu ids-fluid-mechanics-hagen-poiseuille-pipe-flow-pressure-drive.png 25 Obstruction Flow Meters: Laminar Flow Element Q ~ (P1-P2) Hagen–Poiseuille equation: Q = (P1-P2)πr4/8μL Q: Volumetric flow rate P1: Static pressure at the inlet P2: Static pressure at the outlet r: Pipe radius μ: Absolute viscosity of the fluid L: Length of the restriction 🡪 Q ~ (P1-P2)/μ https://www.researchgate.net/profile/Weidong_Li8/publication/261721143/figure/fig7/AS:66 8856729210882@1536479279101/Sketch-of-the-Hagen-Poiseuille-flow.ppm Note that μ = μ(T) 🡪 Changes in gas temperature affect the absolute viscosity of the gas 🡪 causes systematic errors in the estimation of Q, unless compensated for. 26 Obstruction Flow Meters: Laminar Flow Element Establishment of laminar flow through capillary tubes or honeycomb-like structures The LFE forces the gas molecules to move in parallel paths along the length of the passage, nearly eliminating flow turbulence The differential pressure drop is measured within the laminar region Q ~ (P1-P2)/μ https://www.alicat.com/wp-content/uploads/2017/11/laminar-flow-element.gif https://www.researchgate.net/publication/256489801_Practical_Strategies_for_Stable_Operation_of_HFF-QCM_in_Continuous_Air_Flow/figu res?lo=1&utm_source=google&utm_medium=organic 27 Obstruction Flow Meters: Laminar Flow Element 🡪 Q ~ (P1-P2)/μ Works under the same operating principle as all obstruction flowmeters —pressure drop through an obstruction The difference here is that the flow through the pipe is distributed through hundreds or thousands of small diameter tubes https://control.com/uploads/textbooks/flow12.jpg 28 Laminar Flow Element: Entrance Length Q ~ (P1-P2)/μ For a fully developed laminar flow (Re1 🡪 Q ~ (P1-P2)/μ https://i.ytimg.com/vi/FWaO17n2pIc/maxresdefault.jpg https://www.alicat.com/wp-content/uploads/2017/11/laminar-flow-element.gif 30 Laminar Flow Element: Use With Different Gasses/Different Temperatures Q ~ (P1-P2)/μ Hagen–Poiseuille equation: Q = (P1-P2)πr4/8μL Q: Volumetric flow rate P1: Static pressure at the inlet P2: Static pressure at the outlet r: Pipe radius μ: Absolute viscosity of the fluid L: Length of the restriction 31 Laminar Flow Element: Advantages and Limitations Q ~ (P1-P2)/μ Advantages: Can be used to measure low flow rates Ability to measure the flow of liquids with large viscosity Linear relationship between flow rate and pressure drop 🡪 No need to use square root extractor Low noise Limitations: Affected by temperature 🡪 needs temperature compensation The permeant pressure drop is large compared to that of other obstruction flowmeters Large volume flow rates may require tiny diameter tubes to keep the flow laminar https://www.researchgate.net/publication/256489801_Practical_Strategies_for_Stable_Operation_of_HFF-Q CM_in_Continuous_Air_Flow/figures?lo=1&utm_source=google&utm_medium=organic If the flow is dirty, the tubes may become clogged 32 Flow Rate Measurements Obstruction Flow Meters: ▪ Orifice meter ▪ Flow nozzle Q ~ SQRT (P1-P2) ▪ Venturi-meter Laminar flow meter Q ~ (P1-P2) Variable–area flow meters (Rotameters) Q ~ f(float position) Turbine/paddle-wheel flow meter Q ~ f(RPM) Vortex-shedding flow meter Q ~ f(frequency of vortex shedding) Ultrasonic flow meter Q ~ f(transit time) Positive-displacement flow meters Q ~ f(number of turns) Selection criteria Calibration of flow meters 33 Variable-Area Flow Meters: Rota-Meters 0.5 Cd Afloat ρfluid V2 = g Vfloat (ρfloat – ρf) https://qtxasset.com/files/sensorsmag/nodes/2002/1068/fig1.gif 34 Variable-Area Flow Meters: Rota-Meters Consist of a tapered conical transparent tube with a float inside that is free to move. As fluid flows through the tapered tube, the float rises within the tube to a location where the float weight, drag force, and buoyancy force balance each other. https://qtxasset.com/files/sensorsmag/nodes/2002/1068/fig1.gif 35 Flow Meters: Rota-Meters A floating mass (usually a sphere) called afloat rises due to aerodynamic drag The cross-sectional area of the channel increases with height 🡪 the average fluid speed decreases with height 🡪 the floating mass stabilizes at a vertical location where the float weight, drag force, and buoyancy force balance each other 0.5 Cd Afloat ρfluid V2 = g Vfloat (ρfloat – ρf) https://qtxasset.com/files/sensorsmag/nodes/2002/1068/fig1.gif 36 Flow Meters: Rota-Meters Scale is usually calibrated in units of volume or mass flow rate 🡪 Calibrated for a specific fluid 🡪 Correction is needed for different fluids https://qtxasset.com/files/sensorsmag/nodes/2002/1068/fig1.gif 37 Rota-Meters: Advantages ✔ Passive transducer + indicator included ✔ No moving parts, except the float (which is stationary during steady flow) 🡪 Reliable ✔ Low cost ✔ Provides direct reading, without the need of secondary transducers ✔ Suitable for liquids and/or gases ✔ Changeable floats 🡪 A wide range of flow rates using the same tapered tube ✔ Handle corrosive fluids ✔ Can easily be connected to an alarm switch or a transmitting device https://d1twohzh90cvik.cloudfront.net/assets/files/1064/king_7520_7530_s eries_rotameter.1920x0.jpg 38 Rota-Meters: Limitations X Gravity based 🡪 Must be mounted vertically X Typical accuracy from 1 to 5% 🡪 Not suitable for applications that require large accuracy X Low and medium pressures: Glass or plastic transparent tubes X Glass tubes are subjected to breakage 🡪 High pressure applications use metal tubes 🡪 Float position has to be sensed magnetically or electrically X More difficult to connect to computers or miro-processors https://d1twohzh90cvik.cloudfront.net/assets/files/1064/king_7520_ 7530_series_rotameter.1920x0.jpg 39 Flow Rate Measurements Obstruction Flow Meters: ▪ Orifice meter ▪ Flow nozzle Q ~ SQRT (P1-P2) ▪ Venturi-meter Laminar flow meter Q ~ (P1-P2) Variable-area flow meter (Rotameter) Q ~ f(float position) Turbine/paddle-wheel flow meter Q ~ f(RPM) Vortex-shedding flow meter Q ~ f(frequency of vortex shedding) Ultrasonic flow meter Q ~ f(transit time) Positive-displacement flow meter Q ~ f(number of turns) Selection criteria Calibration of flow meters 40 Flow Meters: Turbine Flow Meters Rotational speed of turbine is proportional to velocity and hence to flow rates High accuracy – Maximum error can be as low as 0.25% of full-scale reading Flow can go in either direction https://encrypted-tbn0.gstatic.com/images?q=tbn:ANd9GcQnZIj5A2lIB-0piLPJHGX hYhaaL0cy0VlOeQ&usqp=CAU Uses a free-spinning turbine wheel, which rotates as the fluid flows through the pipe The RPM of the turbine is directly proportional to the flow velocity of the fluid 🡪 directly proportional to the flow rate 41 Flow Meters: Turbine Flow Meters Uses a free-spinning turbine wheel, which rotates as the fluid flows through the pipe The RPM of the turbine is directly proportional to the flow velocity of the fluid 🡪 directly proportional to the flow rate Q = k * RPM https://encrypted-tbn0.gstatic.com/images?q=tbn:ANd9GcQnZIj5A2lIB-0piLPJHGX The meter factor K is found by direct calibration hYhaaL0cy0VlOeQ&usqp=CAU 42 Turbine Flow Meters: Advantages and Limitations Advantages: ✔ Excellent rangeability ✔ Can operate in wide range of temperature and pressure ✔ Easy to install, maintain and to calibrate ✔ Low permeant pressure drop across the turbine Limitations: X Corrosive fluids may damage the turbine blades https://encrypted-tbn0.gstatic.com/images?q=tbn:ANd9GcQnZIj5A2lIB-0piLPJHGX X Careful with cavitation hYhaaL0cy0VlOeQ&usqp=CAU X Very sensitive to fluid viscosity 🡪 may need temperature correction because the viscosity is a function of temperature X Operation with moving parts 🡪 Lower reliability and durability 43 Flow Meters: Paddle Flow Meters Similar to the turbine flow meter but does not obstruct the entire cross section 🡪 Lower pressure drop but lower sensitivity than the turbine meter https://www.flowmeters.com/images/6-big_0_0.gif https://www.epgco.com/wp-content/uploads/2016/10/reg_eseriesflowsensor01.gif https://encrypted-tbn0.gstatic.com/images?q=tbn:ANd9GcQnZIj5A2lIB-0piLPJHG XhYhaaL0cy0VlOeQ&usqp=CAU 44 Flow Rate Measurements Obstruction Flow Meters: ▪ Orifice meter ▪ Flow nozzle Q ~ SQRT (P1-P2) ▪ Venturi-meter Laminar flow meter Q ~ (P1-P2) Variable–area flow meters (Rotameters) Q ~ f(float position) Turbine/paddle-wheel flow meter Q ~ f(RPM) Vortex-shedding flow meter Q ~ f(frequency of vortex shedding) Ultrasonic flow meter Q ~ f(transit time) Positive-displacement flow meters Q ~ f(number of turns) Selection criteria Calibration of flow meters 45 Vortex Shedding when flow passes by a bluff body (a disc, a stubby cylinder or a body of square or triangular cross section) at a certain Reynolds number range, vortices are shed periodically The frequency of the vortex shedding is directly proportional to the velocity of the fluid flowing in the pipeline Von Kármán effect https://hmf.enseeiht.fr/travaux/projnum/sites/default/files/users/tha https://upload.wikimedia.org/wikipedia/commons/b/b4/Vortex-street-animation.gif n/Untitled.png 46 Strouhal Number The nondimensional Strouhal number St = f*d/V is related to the Reynolds number d is the characteristic dimension of the bluff body, f is the vortex shedding frequency, and V is the average flow velocity https://upload.wikimedia.org/wikipedia/commons/0/05/Srrrpd.png https://upload.wikimedia.org/wikipedia/commons/b/b4/Vortex-street-animation.gif 47 Flow Meters: Vortex-Shedding Meters https://www.efunda.com/designstandards/sensors/flowmeters/images/vortex2.gif https://instrumentationtools.com/wp-content/uploads/2015/07/Vortex-Flow-Met er-Working-Animation.gif 48 https://i.gifer.com/DELU.gif 49 Vortex-Shedding Meters Advantages: ✔ No moving parts 🡪 simple structure 🡪 reliable performance + long service life + low maintenance ✔ Wide measuring range 🡪 the turndown ratio can reach 1:10 ✔ Operation is not affected by temperature, pressure, density or viscosity of the fluid being measured 🡪 Flows with excessive temperatures/pressures can be measured ✔ Operates with liquids, gases or vapors https://instrumentationtools.com/wp-content/uploads/2015/07/Vortex-Flow-Met er-Working-Animation.gif 50 Vortex-Shedding Meters Limitations X Not useful for very low Reynolds numbers X Large permeant pressure loss (Large loading effect) X External vibrations can cause measurement errors 🡪 poor anti-vibration performance X High flow velocity shock of the incoming fluid may cause vibrations in the vortex body 🡪 reduces the measurement accuracy X Cannot measure dirty media https://instrumentationtools.com/wp-content/uploads/2015/07/Vortex-Flow-Met er-Working-Animation.gif X Straight pipe requirements are high when mounting the vortex flow meter X Not suitable for low Reynolds number fluids measurements X Not suitable for pulsating flows 51 Flow Rate Measurements Obstruction Flow Meters: ▪ Orifice meter ▪ Flow nozzle Q ~ SQRT (P1-P2) ▪ Venturi-meter Laminar flow meter Q ~ (P1-P2) Variable-area flow meter (Rotameter) Q ~ f(float position) Turbine/paddle-wheel flow meter Q ~ f(RPM) Vortex-shedding flow meter Q ~ f(frequency of vortex shedding) Ultrasonic flow meter Q ~ f(transit time) Positive-displacement flow meter Q ~ f(number of turns) Selection criteria Calibration of flow meters 52 Ultra-Sonic Flow Meters Time-of-travel time Non-intrusive Doppler-effect Uses sound waves to infer the volume flow rate 🡪 Ultrasonic means that the frequency of the wave is higher than the human range of hearing (i.e. > 20 kHz), typically 1 MHz 53 Ultra-Sonic Flow Meters Ultrasonic flowmeters can be divided into time-of-travel meters versus Doppler meters. Time-of-travel Two transducers mounted on each side of the pipe. A time difference proportional to the flow can be detected. https://instrumentationtools.com/wp-content/uploads/2019/02/V-Type-Transit-Time-Flow-M eter.png Non-intrusive Doppler-effect Measures the frequency shift caused by the liquid flow. Two transducers are mounted in a case 🡪 The frequency shift is proportional to the liquid velocity. https://www.ufmflowmeters.com/wp-content/uploads/2016/02/Doppler-measur e-principle_U-F-M_Ultrasonic-Flow-Management.png 54 Ultra-Sonic Flow Meters: Time of Travel Flow Meter An upstream transmitter sends a wave🡪 The wave travels through the flow to the downstream transmitter The downstream transmitter sends another wave 🡪 The wave travels through the flow to the upstream transmitter The downstream sound waves are carried along by the flow 🡪 they https://instrumentationtools.com/wp-content/uploads/2019/02/V-Type-Transit-Time-Flow-M travel faster than the wave sent by the upstream transmitter eter.png Electronic circuits measure the difference in travel time The difference in travel time = f(flow velocity) = f(flow rate) The diameter is known and the volume flow rate is estimated as an average velocity * cross-sectional area of the pipe 55 Ultra-Sonic Flow Meters: Doppler Effect The flow meter is installed on the outside surface of a pipe The transducer transmits a sound wave at a fixed frequency through the pipe wall and into the flowing fluid The sound waves are reflected by impurities (suspended solid particles or entrained gas bubbles) 🡪 the reflected waves are sensed by a receiver mounted close to the transmitter. Due to the Doppler effect, the frequency shift between the reflected https://www.ufmflowmeters.com/wp-content/uploads/2016/02/Doppler-measur sound waves and the transmitted sound waves is proportional to the e-principle_U-F-M_Ultrasonic-Flow-Management.png average flow velocity in the pipe The diameter is known and the volume flow rate is estimated as an average velocity * cross-sectional area of the pipe 56 Ultra-Sonic Flow Meters Advantages ✔ High accuracy and high precision ✔ Non-intrusive: Can clamp on a pipe without disconnecting the pipe 🡪 Can work with flows with extreme pressures and temperatures ✔ No moving parts: Durable + reliable + low maintenance Limitations X High initial cost X Dirt and impurities inside the fluid impact the measurement accuracy X Noise on the same frequency range interfere with the detection of sonic pulses 57 Flow Rate Measurements Obstruction Flow Meters: ▪ Orifice meter ▪ Flow nozzle Q ~ SQRT (P1-P2) ▪ Venturi-meter Laminar flow meter Q ~ (P1-P2) Variable-area flow meter (Rotameter) Q ~ f(float position) Turbine/paddle-wheel flow meter Q ~ f(RPM) Vortex-shedding flow meter Q ~ f(frequency of vortex shedding) Ultrasonic flow meter Q ~ f(transit time) Positive-displacement flow meter Q ~ f(number of turns) Selection criteria Calibration of flow meters 58 Positive-Displacement Flow Meters The fluid flows into a chamber of known volume and is trapped there The fluid trapped into the chamber is displaced from upstream to downstream and then is discharged The number of discharges is counted per unit time Usually indicate the total flow in volume units, as opposed to flow rates in volume/time units Suitable with high viscosity liquids because as the viscosity increases, the fluid slippage between the chambers drops and the accuracy increases. 59 Positive-Displacement Flow Meters Advantages ✔ Low-cost measurements 🡪 Suitable for measurements of utilities (E.G., gas and water at home) ✔ Best in smaller pipe sizes ✔ Suitable for low flowrates ✔ Suitable for fluids of large viscosity ✔ Durable ✔ Insensitive to distortions in inlet flow profile 60 Positive-Displacement Flow Meters Limitations ✔ Moving parts🡪 Less durability and reliability and more need for maintenance ✔ Can create large permanent pressure drops downstream of https://www.cfw.com.my/lz_images/NBDC/Oval%20Gear%20Positive%20Displace the meter ment%20Flowmeter/LC11-1.jpg ✔ Dirty fluids 🡪 must disassemble and clean ✔ Measures discrete fluid flows (m3) instead of a flow rate (m3/s) https://image.made-in-china.com/2f0j00tgOUYIoGJBkj/High-Flow-Positive-Displac ement-Flow-Meter.jpg 61 Flow Meters: Selection Criteria 62 Flow Meters: Calibration Flow meter calibration involves comparing the measurements of a certain flow meter in operation to that of a standard flow measurement device under the same conditions Make leak-free connections, then produce and maintain steady-state flow, then compare the reference flow meter with the Device Under Test (DUT) over the same time period Many standards require a 4:1 ratio between the specified https://www.equilibar.com/application/flow-meter-calibration/ tolerance of the device under test (DUT) and the uncertainty of the calibration equipment 63 Flow Rate Measurements Obstruction Flow Meters: ▪ Orifice meter Cd ~ 0.60 ▪ Flow nozzle Q ~ SQRT (P1-P2) Cd ~ 0.96 ▪ Venturi-meter Cd ~ 0.98 Laminar flow meter Wide range of flow rates Q ~ (P1-P2) Variable-area flow meter (Rotameter) Q ~ f(float position) Direct reading Turbine/paddle-wheel flow meter Most accurate Q ~ f(RPM) Vortex-shedding flow meter Q ~ f(frequency of vortex shedding) Ultrasonic flow meter No moving parts Q ~ f(transit time) Non intrusive Positive-displacement flow meter Q ~ f(number of turns) Low flow rates and Selection criteria utility applications Calibration of flow meters 64 Fluid Velocity Measurements 1 Fluid Velocity Measurements Velocity is a vector that consists of a magnitude (speed) and a direction. Velocity is always a vector, while speed is always a scalar. Two words are used interchangeably to describe the measurement of velocity: anemometry and velocimetry. 2 Fluid Velocity Measurements 3 Fluid Velocity Measurements Pitot-static tubes: Potential flow theory & Bernoulli’s theory Hot wire/film anemometer: Newton’s law of cooling Laser Doppler velocimetry/anemometry (LDV /LDA): Doppler theory Particle-Image Velocimetry: Direct measurement 4 Pitot-Static Tubes https://cdn.instrumentationtools.com/wp-content/uploads/2016/06/instrumentationtools.com_pitot-tube-working-principle-animation.gif 5 Pitot-static tubes in aero planes https://www.keyshone.com/wp-content/uploads/2014/11/pitot-tube-21.jpg Tube can be blocked by moisture, ice, dirt, insects, etc…. 6 Pitot-Static Tubes 7 Pitot-Static Tubes https://www.youtube.com/watch?v=IAojySsbnhg 8 Pitot-Static Tubes ✔ Pitot-static tube measure the velocity of a steady incompressible flow velocity at a point ✔ Pressure sensors are needed to measure the total and static pressures 9 Pitot-Static Tubes: Compressibility Effect 10 Pitot-Static Tubes: Stagnation (Total) Pressure ✔ The stagnation pressure is the pressure measured when the velocity is zero. ✔ The stagnation pressure is obtained when the fluid is brought to rest through an isentropic process ✔ The static port measures static or still fluid — fluid that is not affected by the airplane's speed through the air 11 Pitot-Static Tubes: Static Pressure Static pressure holes should be at least a distance of 3D from the leading edge and at least 8D from the stem. 12 Pitot-Static Tubes: Wall Boundary Effects The static pressure indication is sensitive to distance from solid boundaries. The probe and boundary form a Venturi passage, which accelerates the flow and decreases the static pressure on one side. Static readings should not be taken closer than 5 tube diameters from the wall for 1% accuracy Ten tube diameters yields ~ 0% error. 13 Pitot-Static Tubes: Dynamic Pressure The differential pressure is typically quite small for air flow as compared to liquid flows Small differential pressures can be measured by an electric pressure transducer 14 Dynamic Response of Pitot-Static Tubes The dynamic response depends on the length and diameter of the pressure passages inside the probe the size of the pressure tubes to the manometer, and the displacement volume of the manometer. Dynamic response time is inversely proportional to tube diameters For this reason, 1/16" OD is the smallest recommended size for ordinary use. This probe will take 15 to 60 seconds to reach equilibrium pressure with typical manometer connections. Tubes with an OD of 1/32" have a time constant of as long as 15 minutes and they clog up very easily with fine dirt in the flow stream. 15 Pitot Tubes for Multi-Dimensional Flows 2-hole pitot-static probe (1D) 3-hole pitot-static probe (2D) 5-hole pitot-static probe (3D) 7-hole pitot-static probe (3D) 16 16 Five-Hole Pitot-Static Tube https://amt.copernicus.org/articles/11/2583/2018/amt-11-2583-2018.pdf 17 Five-Hole Pitot-Static Tube https://ars.els-cdn.com/content/image/1-s2.0-S0955598614000843-gr1.jpg 18 Five-Hole Pitot-Static Tube ✔The five-hole probe can be used to deduce the total pressure, the static pressure, the dynamic pressure, the flow velocity and the flow direction in subsonic multi-dimensional flows ✔The probe consists of four direction-sensing ports plus a center port. It has two holes in the yaw plan and two holes in the pitch plan ✔By sampling this distribution in five points it is possible to determine the direction and magnitude of the velocity vector 19 Five-Hole Pitot Tube: Pitch and Yaw Angles 20 Seven-Hole Pitot-Static Tube 21 Seven-Hole Pitot-Static Tube 22 7-Hole Probe 23 7-Hole Probe 24 A pitot tube is inserted in an air flow at STP to measure the flow speed. The tube is inserted so that it points upstream into the flow and the pressure sensed by the tube is the stagnation pressure. The static pressure is measured at the same location in the flow, using a wall pressure tap. If the pressure difference is 30 mm of mercury, determine the flow speed and the Mach number. Assumptions: (1) Steady flow (2) Incompressible flow (3) Flow along a streamline (4) Isentropic deceleration along stagnation streamline (5) No shaft work or heat transfer At T= 20°C, the speed of sound in air is 343 m/s. Hence, M = 0.236 and the assumption of incompressible flow is valid. 25 Hot-wire Anemometry: Big Picture 26 Hot-wire Anemometry: Constant-Temperature Mode 27 Lasers – Light Amplification by Stimulated Emission of Radiation: LDA Laser light is very different from normal light. Laser light has the following properties: The light released is monochromatic. It contains one specific wavelength of light (one specific color). The wavelength of light is determined by the amount of energy released when the electron drops to a lower orbit. The light released is coherent. It is “organized” -- each photon moves in phase with the others. This means that all of the photons have wave fronts that launch in union. The light is very directional. A laser light has a very tight beam and is very strong and concentrated. A flashlight, on the other hand, releases light in many directions, and the light is very diffuse. 28 Lasers – Light Amplification by Stimulated Emission of Radiation: LDA 29 1 Pressure Measurements https://www.dlr.de/as/en/Portaldata/5/Resources/images/abteilungen/abt_ev/artikel/PSP_img1_xl.jpg cdn4.explainthatstuff.com/torricellian-barometer.png 2 3.bp.blogspot.com/-sSndbtIzn1c/TmJdYTzkCPI/AAAAAAAABHc/5xHtU7CdYwA/s1600/Utube.jpg Pressure Measurements Definition Units Pressure terminology Different sensing methods ❑ Gravitational ▪ Liquid columns ▪ Pistons and weight ❑ Mechanical Bourdon tubes Diaphragms Bellows ❑ Electrical ▪ Piezo-resistive ▪ Piezo-electric ▪ Capacitive ▪ LVDT Pressure measurements inside fluid fields Pressure sensitive paints Intelligent pressure transducers i.pinimg.com/originals/fa/9b/0a/fa9b0a46f59dbd11436760539e4d7797.png Pressure Sensors: Selection Guides cdn4.explainthatstuff.com/torricellian-barometer.png www.instrumentationtoday.com/wp-content/uploads/2011/09/Bourdon-Tube-Pressure-Gauge.jpg 3 3 Definition of Pressure Pressure is the normal force exerted by a fluid per unit area https://img.webmd.com/dtmcms/live/webmd/consumer_assets/site_images/articles/health_tools/worst_shoes_slideshow/webmd_rf_photo_of_ foot_pain_from_heels.jpg https://hips.hearstapps.com/vader-prod.s3.amazonaws.com/1598629418-dr-scholls-shoes-no-bad-vibes-sneaker-1598629386.jp g upload.wikimedia.org/wikipedia/commons/thumb/f/ff/Pressure_force_area.svg/200px-Pressure_force_area.svg.p ng 4 Units of Pressure 1 bar = 1.019716213 kgf/cm2 = 105 Pa = 14.5038 PSI 1 atm = 101325 Pa 1 torr = 1 mm Hg =101.325 kPa = 1.01325 bar =14.696 PSI =760 mm Hg =29.9 in. Hg =760 torr 5 Electric Pressure Sensors: Terminology Pressure Transducer – Provides an output voltage proportional to the applied pressure. Pressure Transmitter – Provides an output current proportional to the applied pressure. https://i.ytimg.com/vi/APK1nG5U2hw/maxresdefault.jpg 6 Pressure Terminology Absolute Pressure Pressure measured relative to zero pressure Gage Pressure Pressure measured relative to the ambient atmospheric pressure (Pabsolute > Patmosphere) Vacuum pressure Pressure measured relative to the ambient atmospheric pressure (Pabsolute < Patmosphere) https://nptel.ac.in/content/storage2/courses/112104118/lecture-4/images/fig4.1.gif 7 Pressure Terminology Differential Pressure Pressure measured between two different ports https://media.cheggcdn.com/media/ae9/ae972b6d-c398-4761-bb9d-ba8df82ffda7/p hp2ztmiy.png 8 Pressure Terminology Standard Temperature and Pressure STP When working with gases, standard temperatures and pressures are referred to Standard temperature: 273.15 K ( = 0 oC) Standard pressure = 1 atm 9 Electric Pressure Sensors: Terminology Proof Pressure – Maximum pressure that can be applied without changing the performance beyond specifications. Typically 1x to 3x operating range Burst Pressure – Maximum pressure that may be applied without physical damage to the sensing element Thermal Error – Maximum change in output that occurs when the temperature is changed from room temperature to some other specified temperature extreme Thermal Zero Drift – The zero shift due to changes of the ambient temperature from room temperature to the pixabay.com/photos/pressure-gauge-industrial-clock-980392/ specified limits of the operating range 10 Pressure Measurements Definition Units Pressure terminology Different sensing methods ❑ Gravitational ▪ Liquid columns ▪ Pistons and weight ❑ Mechanical Bourdon tubes Diaphragms Bellows ❑ Electrical ▪ Piezo-resistive ▪ Piezo-electric ▪ Capacitive ▪ LVDT Pressure measurements inside fluid fields Pressure sensitive paints Intelligent pressure transducers i.pinimg.com/originals/fa/9b/0a/fa9b0a46f59dbd11436760539e4d7797.png Pressure Sensors: Selection Guides cdn4.explainthatstuff.com/torricellian-barometer.png www.instrumentationtoday.com/wp-content/uploads/2011/09/Bourdon-Tube-Pressure-Gauge.jpg 1111 Gravitational Pressure Sensors Barometers Ambient pressure affects the results of a lot of experiments Used to measure atmospheric air pressure Ambient pressure is used to predict short-term changes in the weather ΔP = ρm g h cdn4.explainthatstuff.com/torricellian-barometer.png 12 Gravitational Pressure Sensors Manometers Typical manometric fluids are mercury, oil or water The units of measures are inches of mercury, inches of 3.bp.blogspot.com/-sSndbtIzn1c/TmJdYTzkCPI/AAAAAAAABHc/5xHtU7CdYwA/s1600/Utube.jpg oil or inches of water https://qtxasset.com/files/sensorsmag/nodes/2003/969/fig3.gif ΔP = (ρm - ρf) g h Keep in mind that ρ = ρ (T) 13 Gravitational Pressure Sensors Manometers www.engineeringclicks.com/wp-content/uploads/2019/01/u-tube-manometer-basic- principle-high.jpg https://chbe241.github.io/_images/Combined-Mano-2.svg ΔP = (ρm - ρf) g h 14 Gravitational Pressure Sensors Inclined U-Tube Manometers The indicating tube of the U-type manometer is inclined -> greater movement along the tube for a given pressure difference -> increased readability + increased sensitivity Accurate reading in this case requires that the inclined portion of the scale must be at the exact angle for which it is designed https://encrypted-tbn0.gstatic.com/images?q=tbn:ANd9GcRXKksJ1XYeqFkV83WwLajHzhwQT7XyusPdfw&usqp=CAU Can be used to measure lower pressures than U-tube manometers 15 Gravitational Pressure Sensors Well-Type Manometers To overcome the U-tube requirement of readings at two different places, the well-type manometer is used The well (reservoir) is made large enough so that the change of level in the reservoir is negligible / or the scale is compensated for the change in the liquid level in the reservoir www.polytechnichub.com/wp-content/uploads/2017/08/well-type-manometer.jpg Inaccuracies in the diameter of the reservoir or the indicating tube -> systematic error If the indicating tube is not perpendicular to the ground -> systematic error 16 Gravitational Pressure Sensors Inclined Well-Type Manometers The indicating tube of the well-type manometer is inclined -> greater movement along the tube for a given pressure difference -> increased readability + increased sensitivity Accurate reading in this case requires that the https://cdn.instrumentationtools.com/wp-content/uploads/2017/09/instrumentationtools.c inclined portion of the scale must be at the exact om_inclined-limb-manometer-principle.png angle for which it is designed 17 Manometers Inclined-Tube Manometer U-Tube Manometer Differential U-Tube Manometer ΔP = (ρm - ρf) g h Barometer https://www.ecourses.ou.edu/cgi-bin/eBook.cgi?doc=session_blank&topic=fl&chap_sec=02.2&page=theory 18 Gravitational Pressure Sensors Manometers: Factors affecting performance and usage I- Improve readability ✔ Red oil is used as an indicating fluid ✔ Scales are compensated to read pressure directly in the required units ✔ Use of fluids that give a uniform, well defined https://upload.wikimedia.org/wikipedia/commons/thumb/3/34/Parallax_Example_en.svg/46 meniscus facilitate accurate reading 5px-Parallax_Example_en.svg.png ✔ Parallax-free reading is made by aligning meniscus with its reflection in the polished scale ✔ Smoothly machined inside diameters of the manometer further enhance the visibility of the meniscus https://www.dwyer-inst.com/images/manometer-intro5_pic.gif 19 19 Gravitational Pressure Sensors Manometers: Factors affecting performance and usage II- Characteristics of indicating fluid ✔ The fluid must have good wetting https://study.com/cimages/multimages/16/meniscus.png characteristics -> well-shaped meniscus ✔ Large sensitivity requires low density ✔ Large operating range requires large density ✔ Example: The density of mercury is nearly 13.6 times the density of water. Mercury gives larger measurement range but lower sensitivity https://control.com/uploads/textbooks/006.jpg ΔP = (ρm - ρf) g h 20 Gravitational Pressure Sensors Manometers: Factors affecting performance and usage III- Bore straightness ✔ The tube must be straight -> careful with 90 degree angle with the ground ✔ Avoid bumps or vibration in the tube https://instrumentationtools.com/wp-content/uploads/2017/09/instrumentationtools.com_ u-tube-manometer-principle.png 21 Gravitational Pressure Sensors Manometers: Summary U-tube and inclined manometers are simplest pressure sensing devices – Micro-manometers Advantages – Simple – Inexpensive – Can be highly sensitive (inclined) Limitations – Poor dynamic response – No remote sensing – Limited pressure range https://instrumentationtools.com/wp-content/uploads/2017/09/instrumentationtools.com_u-tu Not practical over 7 bar be-manometer-principle.png ΔP = (ρm - ρf) g h 22 Gravitational Pressure Sensors Dead Weight Tester: Pascal’s Law A pressure change at any point in a confined incompressible fluid is transmitted throughout the fluid such that the same change occurs everywhere https://previews.123rf.com/images/udaix/udaix1710/udaix171000018/88190000-pascal%E2%80%99s-law-infographic-diagra m-showing-an-example-of-body-of-fluid-held-by-hand-and-an-external-pr.jpg https://cdn.britannica.com/06/156806-050-3D1E6425/principle-press-Illustration-work-force-Pascal-pressure.jpg 23 Gravitational Pressure Sensors Dead Weight Tester A dead weight tester is an instrument that calibrates pressure by determining the weight divided by the area on which the force is applied P = (weight force = mg) / A ( = area on which the force is applied) -> primary measurement -> High accuracy -> Uncertainty is within 0.01 to 0.05% of the reading Primary standards -> Used primarily for static calibration Can measure extremely high pressures (up to 10,000 PSI) Used for static calibration of pressure measuring devices P=F/A F = weight force = mg A = area on which the force is applied 24 Gravitational Pressure Sensors Dead Weight Tester Advantages: 1. Simple in construction and easy to use 2. All the hardware required to generate, control and measure static pressures are contained within the instrument 3. Fluid pressure can be easily varied by adding weights or by changing the piston-cylinder combination 4. It can be used to calibrate a wide range of pressure measuring devices Limitations: 1. Affected by the friction between the piston and cylinder 2. Affected by the uncertainty of the value of gravitational constant g Poor dynamic response -> Can NOT be used for dynamic pressure measurements -> Used for static calibration of pressure-measuring devices 25 Pressure Measurements Definition Units Pressure terminology Different sensing methods ❑ Gravitational ▪ Liquid columns ▪ Pistons and weight ❑ Mechanical Bourdon tubes Diaphragms Bellows ❑ Electrical ▪ Piezo-resistive ▪ Piezo-electric ▪ Capacitive ▪ LVDT Pressure measurements inside fluid fields Pressure sensitive paints Intelligent pressure transducers i.pinimg.com/originals/fa/9b/0a/fa9b0a46f59dbd11436760539e4d7797.png Pressure Sensors: Selection Guides cdn4.explainthatstuff.com/torricellian-barometer.png www.instrumentationtoday.com/wp-content/uploads/2011/09/Bourdon-Tube-Pressure-Gauge.jpg 2626 Mechanical Pressure Sensors Bourdon Tube Gages Contain an elastic element that deforms under pressure and creates a linear or angular displacement -> The displacement is transformed to mechanical linkages and then displayed on a dial / OR transformed to an electric signal then displayed https://ars.els-cdn.com/content/image/3-s2.0-B9780080552330000042-gr22.jpg Response time is mechanical in nature -> not useful for dynamic pressure measurements -> used only in static applications, like monitoring the supply pressure Avoid excessive input pressures in order to avoid plastic deformations cdn4.explainthatstuff.com/torricellian-barometer.png 27 27 3.bp.blogspot.com/-sSndbtIzn1c/TmJdYTzkCPI/AAAAAAAABHc/5xHtU7CdYwA/s1600/Utube.jpg Mechanical Pressure Sensors Bourdon Tube Gages: C Type https://www.scienceabc.com/wp-content/uploads/2020/01/Pressure-gauge-manometer-closeupEnsup ers.jpg https://www.instrumentationtoday.com/wp-content/uploads/2011/09/Bourdon-Tube-Pressu https://cdn.instrumentationtools.com/wp-content/uploads/2015/08/Bourdon-Tube-Working-Princi re-Gauge.jpg ple-Animation.gif 28 Mechanical Pressure Sensors Bourdon Tube Gages: Spiral and Helical Types Spiral and Helical Bourdon tube gages: Can measure pressures up to 1000 psi at large sensitivity https://www.scienceabc.com/wp-content/uploads/2020/01/bourdon-tube.jpg https://www.computatest.com/documents/Hysteresis_files/image001.png 29 Mechanical Pressure Sensors Bourdon Tube Gages: Diaphragms A diaphragm extends or contracts like a spring until the force induced by the Hooke’s law balances the pressure difference force Converts pressure into a physical displacement https://instrumentationtools.com/wp-content/uploads/2017/11/instrumentationtools.com_ bellows-diaphragm-and-bourdon-tube.png 30 30 Mechanical Pressure Sensors Bourdon Tube Gages: Bellows Converts pressure differential into a physical displacement, like the diaphragm The displacement here is more straight-line expansion than with the diaphragm Accurate to 0.5% FS (full-scale) https://lh3.googleusercontent.com/proxy/73COxkYax3d4hvE96189YbGe6QRdu48gLtfp8gdR_X9Tj 3mZZccFGntDpFU5py6nf7d87DCJvzz5brJ3ZgQKMgqqaZkeeZIlQ4Z4-7RkGthL Not fast enough for transient measurements Typically used for small pressures 31 https://ars.els-cdn.com/content/image/3-s2.0-B9781483213552500108-f05-03-9781483213552.jpg Mechanical Pressure Sensors Bourdon Tube Gages: Types All except diaphragms provide relatively large physical displacement https://eng.libretexts.org/@api/deki/files/17263/image-15.gif?revision=1 32 Pressure Measurements Definition Units Pressure terminology Different sensing methods ❑ Gravitational ▪ Liquid columns ▪ Pistons and weight ❑ Mechanical Bourdon tubes Diaphragms Bellows ❑ Electrical ▪ Piezo-resistive ▪ Piezo-electric ▪ Capacitive ▪ LVDT Pressure measurements inside fluid fields Pressure sensitive paints Intelligent pressure transducers i.pinimg.com/originals/fa/9b/0a/fa9b0a46f59dbd11436760539e4d7797.png Pressure Sensors: Selection Guides cdn4.explainthatstuff.com/torricellian-barometer.png www.instrumentationtoday.com/wp-content/uploads/2011/09/Bourdon-Tube-Pressure-Gauge.jpg 3333 Electric Pressure Sensors Molecular versus Parametrical Transducers Provide an electric output that depends on the input pressure Output is linear /non-linear with the input pressure Molecular Sensors: The applied input pressure produces a change in a certain electric property of the material https://upload.wikimedia.org/wikipedia/commons/thumb/c/c4/SchemaPiezo.gif/220px-Sche A piezoelectric pressure transducer generates a small maPiezo.gif electric charge when a dynamic pressure is applied to it A piezo resistive pressure transducer has its resistance changes with the applied pressure Parametric Sensors: The applied input pressure causes a change in an electric property (e.g., resistance, inductance, capacitance) of an electric circuit i.pinimg.com/originals/fa/9b/0a/fa9b0a46f59dbd11436760539e4d7797.png 34 Electric Pressure Sensors Molecular Type: Piezoelectric Sensors Polarized crystalline structures like quartz generate a small electric charge when a dynamic pressure is applied on them The charge quantity is proportional to the applied pressure The charge polarity depends on the direction of the force Provides an output only if the input pressure is changing with time-> Dynamic sensors that can only be used when the input pressure is changing https://base.imgix.net/files/base/ebm/electronicdesign/image/2016/09/electronicdesign_com_sites_electronicdesign.com_files _uploads_2015_02_0816_CTE_Yang_F1.png?auto=format&fit=max&w=1440 Dynamic measurements ONLY 35 Electric Pressure Sensors Molecular Type: Piezoelectric Sensors Piezoelectric transducers are bi-directional transducers capable of converting pressure into an electric charge and vice versa Have high output electric impedance -> Avoid connecting the output to electronics that withdraw large currents Have high output electric impedance -> Can use an internal amplifier (charge amplifier) to lower the output electric impedance and thus increase the output current of the piezo-electric sensor https://upload.wikimedia.org/wikipedia/commons/thumb/c/c4/SchemaPiezo.gif/220px-Sche maPiezo.gif 36 Electric Pressure Sensors Molecular Type: Piezoelectric Sensors endevco.com/contentStore/MktgContent/Endevco/uploads/2019/08/EDV-Catalog_Lowres.pd f