Animal Models in Research PDF
Document Details
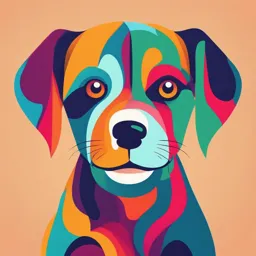
Uploaded by IrreplaceableParadise5419
Radboud University
Tags
Related
- HMB204 Lecture 3: Modern Techniques in Translational Research PDF
- NEUR3101 Motor Learning Lectures 8 & 9 PDF
- Biological Screening of Drug Activities PDF
- LandMunit3 PDF - Learning & Memory
- Ética en la investigación con modelos animales experimentales. PDF
- Neurobiology of Brain and Spinal Cord Disorders - Introduction to Aging (A 780/410G) - January 16, 2025 - PDF
Summary
Document provides a comprehensive overview of animal models in scientific research, covering historical context and explaining the different types of models, motivations for using animal models, and methodologies involved in animal testing. It discusses selecting appropriate species, potential strains, and the various considerations impacting practical, biological, and ethical aspects of the experiments.
Full Transcript
1. Why Use Animal Models? Historical Significance 19th Century Usage: Early in the 19th century, animal studies were not the predominant source of breakthroughs. Less than one third of major advances during this time relied on them. Science at this stage focused heavily on descriptive an...
1. Why Use Animal Models? Historical Significance 19th Century Usage: Early in the 19th century, animal studies were not the predominant source of breakthroughs. Less than one third of major advances during this time relied on them. Science at this stage focused heavily on descriptive anatomy and rudimentary experimentation, often using observational studies in humans rather than manipulative experiments in animals. Later Contributions: By the mid to late 19th century, experimental physiology (like Claude Bernard's work on the pancreas in dogs) began demonstrating the necessity of animal models. Post-19th century, animal studies accounted for more than 50%, sometimes even 75%, of major scientific discoveries. Examples: Vaccines: Pasteur’s development of the rabies vaccine using rabbits. Cardiovascular research: Techniques like cardiac catheterization were developed using animal experiments. Nobel Prize Connection: Out of Nobel prizes awarded since 1901 in Physiology or Medicine, roughly two-thirds involved research using animals. These include: Discovery of insulin in dogs (Banting and Best, 1923). Studies on the polio vaccine (using rhesus monkeys). Scientific Necessity Complexity of Living Systems: While studies on bacteria and isolated cells provide insights, they are limited: Bacteria: Useful for studying basic molecular pathways but inadequate for studying organs or systemic effects. Tissue Cultures: Effective for examining cellular behavior but lack intercellular interactions seen in living organisms. Computer Simulations: Dependent on pre-existing data and models, unable to predict unforeseen biological responses. Examples of Complexity: Blindness: Involves the integration of ocular, neural, and cognitive systems. No single-cell model can mimic this. High Blood Pressure (Hypertension): Arises from cardiovascular, renal, and endocrine interactions. Requires a living system for study. Drug Development: Unanticipated side effects in drugs highlight the need for animal testing. Historical examples: Thalidomide Disaster (1950s-1960s): A sedative that caused birth defects when not tested in pregnant animals. Regulatory bodies like the FDA (US) and EMA (Europe) now mandate animal testing before human trials. 2. Definition of Laboratory Animal Model Official Definition (U.S. National Research Committee on Animal Models for Research on Aging): A laboratory animal model is one that replicates: Normative biology or behavior: Studying baseline biological processes like metabolism or reproduction in mice. Behavior: Social interactions or stress responses in zebrafish. Spontaneous or induced pathological processes: Spontaneous: Diseases naturally occurring in certain breeds, e.g., diabetes in ob/ob mice. Induced: Experimentally creating a disease condition, e.g., injecting pathogens to study immune responses. The key requirement is resemblance to human phenomena: Example: Immune system responses in mice are sufficiently similar to humans to study vaccines. 3. Laboratory Experiments Using Animal Models Experimental Workflow Before the Experiment: Preparation: Extensive literature reviews to avoid redundancy. Consulting experts in specific fields to refine the study approach. Best vs. Common Practices: Best practice implies adherence to the latest ethical and scientific standards. Example: Using analgesics for pain relief during procedures, even if common practice previously ignored this. Frameworks and Guidelines: PREPARE Guidelines: Emphasize planning to reduce unnecessary animal use. Cover aspects like housing, personnel training, and experimental endpoints. During the Experiment: Key components: Execution: Following pre-approved experimental designs. Monitoring: Real-time observation of animals to record behaviors, physiological changes, or signs of distress. Ethical oversight ensures minimizing suffering. After the Experiment: Evaluation: Data is analyzed rigorously to ensure reproducibility and reliability. Refinement of techniques for future studies. Continuous Improvement: Adapting methods to enhance welfare, e.g., switching to less invasive techniques. 4. Classification of Animal Models Normal Animals: These animals exhibit no genetic or physical abnormalities. They are often used as control groups to establish baseline biological responses. Natural Variations: Some populations exhibit extreme phenotypes naturally: Example: Selectively breeding animals for traits like: High vs. low aggression (behavioral studies). High vs. low pathogen resistance (immunological studies). Experimentally Induced Deviations: Diseases or conditions are artificially created for research: Methods: Pharmacological Induction: Administering a drug to cause a specific response (e.g., inducing seizures with pentylenetetrazol). Genetic Engineering: Creating knock-out mice that lack specific genes to study genetic contributions to diseases. Pathogen Introduction: Studying infection progression by introducing a virus. 5. Choosing Species and Strains Species Choice: Mice and Rats: Small, cost-effective, and share mammalian physiology with humans. Example: Used for studying cancer, neurodegeneration, and behavioral sciences. Other Species: Rabbits: Model for eye diseases. Zebrafish: Transparent embryos for developmental studies. Pigs: Closely resemble human cardiovascular systems. Strain Selection: Strains are subsets of a species bred for specific traits: C57BL/6 Mice: General-purpose model for genetic research. Nude Mice: Lack functional T-cells, ideal for cancer and immunological studies. 6. Practical and Biological Considerations Practical: Housing: Certain species require specialized environments. Example: Zebrafish need aquatic tanks. Costs: Smaller species like mice are more economical than primates. Biological: Gender and age impact outcomes: Female animals might show hormonal influences on certain traits. Young vs. old animals could respond differently in aging studies. Overview of Animal Models in Research Animal models are critical tools in research, used to mimic biological and pathological processes to study human conditions. These models help bridge the gap between basic biology and medical applications. The classification described in the slides reflects the types of animal models based on their genetic and experimental modifications. 1. Normal Animals Definition: These are animals without intentional genetic or physical anomalies. They serve as control groups in experiments where deviations caused by treatments are measured against a baseline. Why Important? They provide "clean" baselines since their biological systems represent an unaltered state. Example: Laboratory-bred mice that haven't been subjected to genetic modification or diseases. These could include specific strains bred for consistency in baseline research, such as studying normal metabolism or growth rates. Applications: Baseline physiological and behavioral studies. Comparative analysis to understand the impact of induced anomalies. 2. Naturally Occurring Variants Definition: Animals within this category exhibit genetic differences that arise naturally (e.g., through evolution or random mutations). Subcategories: Genetic Lines with Natural Mutations: Breeding programs identify individuals with unique traits or mutations that could be studied for specific purposes. Example: Mice that naturally carry a mutation associated with diabetes. Selected Extremes in Populations: These animals represent the outliers of a trait spectrum (e.g., highest vs. lowest body weight). Example: Studying aggressive behavior by selecting animals with extreme aggression levels. Why Use Them? Naturally occurring genetic variability mirrors real-world biological diversity, which may help in translating findings to broader populations. Applications: Evolutionary biology: Understanding trait inheritance. Pathogen resistance studies using populations with high or low immunity. 3. Experimentally Induced Deviations/Disease Models Definition: These models are animals in which anomalies, conditions, or diseases are artificially introduced. How Induced? Pharmacological Administration: Drugs are used to trigger certain physiological or pathological states. Example: Using lipopolysaccharides to induce inflammation in rodents. Pathogen Exposure: Introducing infectious agents to study immune responses or disease progression. Surgical or Physical Alterations: Creating lesions or disabling organs to simulate injuries or localized diseases. Genetic Engineering: Techniques like CRISPR allow specific genes to be knocked out (nonfunctional) or modified. Why Use Them? Control over variables: Researchers can design models to reflect specific disease states. Targeted investigation: Study molecular mechanisms or test interventions. Applications: Cancer research: Introducing tumors to study their progression. Neurological studies: Inducing strokes or brain lesions. Special Case Models Elderly Animals: Elderly rodents are used to study aging-related conditions like neurodegeneration or osteoporosis. Key Insight: They naturally model diseases like Alzheimer’s or Parkinson’s, which are harder to simulate in young animals. Nude Mice: Lacking a thymus, these mice have suppressed immune systems. Use: Often employed in cancer research or studies requiring implantation of foreign tissues (e.g., human tumor cells). Inbred vs. Outbred Strains: A Foundational Comparison This section provides a deep dive into why genetic control matters in experiments. Inbred Strains Definition: Produced by over 20 generations of sibling matings to create animals with nearly identical genetic makeups. Aim: Achieve genetic homogeneity (all animals are genetically identical, barring random mutations over time). Detailed Characteristics: Phenotypic Uniformity: All animals exhibit the same traits under similar conditions. This is crucial for reducing noise in experimental data. Reduced Sample Sizes: Since variability is minimized, smaller groups are needed to detect meaningful differences. This reduces the ethical and financial cost of using animals. Reproducibility: Results are consistent across labs and experiments worldwide because the genetic makeup of animals is fixed. Example: A specific inbred mouse strain used in diabetes research will behave identically in multiple laboratories. Implications for Research: Easier to link phenotypic changes to experimental interventions (e.g., drugs, environmental changes). Higher sensitivity to experimental treatments. Applications: Toxicology: Accurately determining dose-response relationships. Pharmacology: Testing drug efficacy and side effects. Genetic Studies: Studying the role of specific genes in diseases. Challenges: Inbreeding Depression: Over many generations, these animals may accumulate deleterious mutations that affect their health or longevity. Lack of Diversity: Results may not translate well to genetically diverse populations, such as humans. Outbred Strains Definition: Animals bred with some genetic variation retained to mimic real-world diversity. Detailed Characteristics: Genetic Diversity: These animals are more similar to natural populations but differ genetically from one another within the same study. Challenges in Research: Uncontrolled Genetic Variables: Differences in experimental outcomes may arise from genetic variability rather than the treatment. Example: A treatment may appear ineffective because genetic variability masks its effects. Larger Sample Sizes Needed: To achieve the same level of statistical power as inbred strains, more animals must be used to account for increased variability. Unreliable Background Data: Traits may shift over time as breeding populations evolve. Applications: Preliminary toxicology studies where variability mimics real-world conditions. Behavioral studies where diverse genetics mirror natural environments. Implications of Genetic Control in Research Controlled Experiments: The principle of controlled experiments is that treated and control groups should only differ by the treatment applied. Inbred strains help achieve this by removing genetic differences as a confounding variable. Statistical Precision: Inbred animals increase the statistical power of experiments, reducing the likelihood of false-negative results. Reproducibility: With searchable databases like The Jackson Laboratory, researchers can consistently use and compare identical strains globally. Why Inbred Strains Are Favored in Toxicology Inbred strains ensure stable, repeatable results, which is critical for safety testing, dose-response studies, and drug development. Using multiple inbred strains in factorial experiments (testing multiple variables simultaneously) avoids increasing the total number of animals while maintaining high precision. Hypothetical Scenarios Outbred Use Case: A drug trial uses outbred rats. The study finds variability in drug response. Was the variability due to the drug or genetic differences? It’s hard to know, requiring more animals and statistical adjustments. Inbred Use Case: The same trial uses inbred rats. The lack of genetic variability ensures observed effects are due to the drug itself, enabling clearer conclusions. New Development: ‘Diversity Outbreds’ What are Diversity Outbreds? These are specially bred animals that have high genetic diversity. Unlike inbred strains, which are genetically identical, diversity outbreds represent a wide range of genetic variations, simulating natural populations. Why Use Them? Traditional inbred animal models might fail to account for genetic variability present in human populations. For example: Inbred animals often react uniformly to a drug, but human responses vary widely. Diversity outbreds allow researchers to evaluate processes like: Reproduction: Understanding fertility and heritability across different genetic makeups. Physiological Processes: Study universal systems like metabolism or stress responses. Toxicology: Test chemical effects across diverse genetic profiles. Key Advantages: Reproducibility Across Generations: By carefully controlling the genetic diversity spread, experiments can be repeated while maintaining genetic variability similar to the original study. Control vs. Experimental Group Similarity: Ensures no genetic bias between the groups, which is critical for accurately attributing outcomes to experimental treatments. Large Sample Size Needs: Genetic variability demands more animals to ensure statistical power. Applications: Quantitative Trait Loci (QTL) Mapping: This is a method to locate genes associated with specific traits (e.g., disease susceptibility, behavioral tendencies). Example: Finding genes that influence susceptibility to stress by correlating genetic markers with cortisol levels. Environmental Effects: Diversity outbreds help isolate how the environment interacts with genetics to influence disease or behavior. Design and Preparation of Experiments Importance of Good Preparation: A poorly designed experiment wastes time, resources, and animal lives, violating ethical principles. Following best practices ensures validity and usefulness of findings. Steps in Experiment Preparation: Literature Review: Surveying previous studies prevents duplication and identifies gaps. Helps in understanding the strengths and weaknesses of existing models and approaches. Consultation with Experts: Experts may provide insights on lesser-known models or techniques. Collaboration ensures broader perspectives, improving the study's quality. Formulating a Precise Research Question: Narrow questions reduce ambiguity and make data interpretation clearer. Example: Instead of "Does stress affect rats?" focus on "How does chronic immobilization stress affect corticosterone levels in female Sprague-Dawley rats?" Designing the Experiment: Write a study plan outlining: Hypotheses to test. Methods, including controls, variables, and replication strategies. Ethical considerations. Follow guidelines like PREPARE to ensure animal welfare and robust experimental design. Choosing the ‘Right Animal Model’ Considerations in Model Selection: Species Choice: Select the species with the lowest complexity that can still meet the research objectives. Example: Studying a genetic disease with a well-conserved gene in zebrafish saves resources while providing relevant insights. If zebrafish genes don’t align, mice may be better due to closer genetic homology to humans. Life Stage: Choose appropriate developmental stages based on research needs (e.g., juvenile for neurodevelopmental studies, adult for chronic disease models). Genetic Modifications: Decide if transgenic (genetically altered) animals are necessary to mimic specific conditions (e.g., inserting a human gene to study its function). Why is Selection Important? Choosing based on availability or cost risks invalid results. Example: Guinea pigs might be inexpensive and familiar, but if studying a condition related to immune response, they could be irrelevant due to their immune system differences from humans. Continuous Evolution in Models: Animal models evolve with advancements in genetics and understanding of diseases. New models might better replicate human conditions, offering improved predictive power for translational research. Laboratory Animal Research Goals Ultimate Objective: Generate insights that predict phenomena in humans or other animals. Examples of phenomena: Physiological Processes: Heart rate variability in stress. Abnormal Behaviors: Depression-like symptoms in mice. Anatomy: Brain changes in neurodegenerative disease models. Factors Enhancing Research Quality: Biological/Clinical Relevance: Ensure the model aligns with the research target. Ethical Values: Minimize harm while maximizing benefits. Standardization: Control variables like housing conditions and diet to reduce noise and improve: Internal Validity: Consistency of results within the study. External Validity: Applicability to broader contexts. Model Validation What is Model Validation? Process of confirming that the animal model accurately mimics the human condition or target phenomenon. Types of Validity: Face Validity: The model displays observable signs similar to the human condition. Example: A mouse showing depressive behaviors like immobility in forced swim tests. Predictive Validity: The model responds to treatments as humans do. Example: A rat model of epilepsy that improves with anti-epileptic drugs. Construct Validity: The underlying mechanisms match the human condition. Example: A genetically modified mouse with the same mutation causing cystic fibrosis in humans. Examples from Slides: Lithium Response in Mice: Mice strains show varying antidepressant-like responses to lithium in the forced swim test. Utility: Helps understand genetic differences in drug response. OHSS Model in Rats: Mimics ovarian hyperstimulation syndrome (OHSS) in women using hormonal treatments. Validated by showing similar physiological changes, like ovarian edema. Ethical Considerations in Model Use Critical Question: Should animals be used if their model lacks construct validity? Models with poor construct validity might mislead conclusions, wasting resources and raising ethical concerns. Choosing the Source of Animals Regulations for Animal Sourcing: Must be bred or purchased from licensed suppliers, as per laws like the Dutch Wod. Ensures traceability, health, and quality of animals. Exemptions: Some animals (e.g., goats, pigs) may not require sourcing from licensed breeders. Slide 1: "How Well Do You Know Your B6 Mice?" The question emphasizes the importance of understanding the genetic and phenotypic differences between substrains of C57BL/6 mice. Even within a single strain, subtle genetic differences can have profound impacts on research outcomes. Why are A and B most similar? Genetic Similarity: A & B differ by a single allele, Tyrc-2J, which causes a coat color mutation. Tyrc-2J stands for "Tyrosinase, recessive allele, 2J" and relates to melanin synthesis, affecting pigmentation. Other aspects of their genome remain identical, minimizing variability. Phenotypic Similarity: Since the only difference between A and B is in pigmentation, they are phenotypically almost identical except for coat color. Why are B and C more different? Multiple Allelic Variations: B and C diverge across multiple genes that affect: Metabolism: Differences in energy production, nutrient absorption, or drug metabolism. Neurobiology: Variability in brain structure, function, and behavior. Immunology: Altered immune responses could affect disease models. Sensory Systems: Variations in genes affecting vision and hearing. These cumulative differences can profoundly affect experimental results. Contextual Note: Even subtle genetic differences can interact with experimental conditions (e.g., diet, stress) to yield different outcomes, which is why researchers must choose substrains carefully. Slide 2: Visible Genetic Drift – Coat Color Mutations What is Genetic Drift? Genetic drift refers to random changes in allele frequencies within a population over generations. In laboratory mouse colonies, this can occur due to: Small breeding populations. Lack of outcrossing (breeding with unrelated mice). Random mutations accumulating over time. Result: Phenotypic changes, such as coat color, may appear. Examples of Coat Color Mutations in C57BL/6 Substrains: C57BL/6J-Aw-J/J: Mutation in agouti gene, affecting coat color patterns. B6(Cg)-Tyrc-2J/J: Tyrosinase mutation, causing lighter pigmentation (albino-like phenotype). C57BL/6J-Lystbg-J/J: Mutation in lysosomal trafficking gene (Lyst), leading to pigmentation issues and other systemic effects. Implications for Research: Coat color mutations serve as visible markers of genetic drift but often correlate with deeper, invisible genetic changes. These mutations may seem superficial but can signal underlying changes in other physiological systems, affecting research reproducibility. Slide 3: B6J vs. B6N C57BL/6J (B6J): This substrain is the "gold standard" in mouse research due to its: High health status: Rigorous quality control ensures minimal infections or undetected health issues. Phenotypic data: Decades of studies provide detailed insights into its characteristics. Reproducibility: Researchers can trust results across studies using this substrain. C57BL/6NJ (B6N): Developed as an NIH substrain (designated "N"). Genetic divergence from B6J has occurred due to separate breeding colonies and environmental conditions. Why Do These Differences Matter? Subtle Genetic Divergence: Even substrains of the same parent strain (e.g., C57BL/6) can differ due to: Single Nucleotide Polymorphisms (SNPs): Tiny DNA changes that alter gene function. Epigenetic Factors: Environmental influences like diet or housing can cause heritable gene expression changes. Experimental Outcomes: B6N may behave differently in disease models or drug trials compared to B6J due to genetic or metabolic differences. Slide 4: Genetic Drift Over Generations Invisible Genetic Drift: Unlike coat color, many genetic changes from drift are not visible but can still affect physiology. Sibling Mating: Common practice in labs for maintaining genetically uniform colonies inadvertently accelerates drift. Example: Lab A and Lab B: Each conducts sibling mating for 10 and 20 generations respectively. Combined, their mice are now 30 generations apart genetically. Consequences of Genetic Drift: Over time, colonies diverge into distinct substrains, even if they started with the same ancestors. Drift introduces unintended variability in: Drug response. Disease progression. Behavioral traits. Slide 5: Proper Nomenclature Why Nomenclature Matters: Mouse strain names indicate their genetic background, origin, and maintenance method. Example: C57BL/6J: "C57BL" refers to the strain lineage. "/6J" indicates the substrain and the originating laboratory (Jackson Labs). Common Designations: NIH: C57BL/6N substrains from the NIH. Crl: C57BL/6NCrl substrains maintained by Charles River Labs. Proper naming avoids confusion and ensures reproducibility by clearly distinguishing substrains. Slide 6: Substrain Divergence How Substrains Diverge: Differences arise due to random mutations, selective breeding, or environmental conditions. Common genetic variations include: SNPs, Indels, and CNVs. Spontaneous mutations affecting metabolism, behavior, or immunity. Phenotypic Implications: Metabolic traits (e.g., obesity or diabetes models) may vary between substrains. Neurobehavioral traits (e.g., anxiety or learning models) depend heavily on genetic background. Immune response differences could alter results in infection or vaccine studies. Slide 7: Choosing the Right Model Key Considerations: Consult literature to ensure the chosen substrain is suited for the specific research question. Account for known phenotypic and genotypic differences between substrains. Common Mistakes: Assuming all C57BL/6 mice are identical. Ignoring genetic drift or substrain-specific traits. Slide 8: Broader Implications Mice as Models: Despite differences from humans, mice are invaluable due to: Genetic similarity in specific pathways. Ability to control environmental and genetic factors. Study-Specific Validity: Models must be validated for each study to ensure relevance. Focus on the specific process being studied (e.g., immune response, cancer growth). Pain in Animals: Physiology, Assessment, and Alleviation What Do We Mean by Pain? General Definition: The International Association for the Study of Pain (IASP) defines pain (2020) as:"An unpleasant sensory and emotional experience associated with, or resembling that associated with, actual or potential tissue damage." This reflects a complex, multi-dimensional phenomenon involving: Sensory Component: Detection and transmission of harmful stimuli. Emotional Component: The distress or suffering associated with the sensation. Cognitive Component: Conscious evaluation and interpretation of the pain. Etymology: Pain originates from the Latin word poena, meaning penalty or punishment, highlighting its association with suffering and harm. Pain vs. Nociception: Pain is a conscious experience that includes emotional and cognitive responses to harmful stimuli. Nociception refers solely to the neural processes involved in detecting harmful (noxious) stimuli. For example, nociceptors (specialized nerve endings) sense stimuli like extreme heat or pressure and send signals to the brain. Under anesthesia, nociception occurs without the perception of pain because the brain’s higher processing centers are not active. Do Animals Feel Pain? Vertebrates: Behavioral and physiological responses in vertebrates (e.g., mammals, birds, reptiles) suggest they experience pain similarly to humans. Examples include: Behavioral Changes: Vocalizations (whining, crying), guarding behaviors, limping, or avoiding movement. Physiological Markers: Elevated heart rate, stress hormones (cortisol), and changes in respiration. Invertebrates: Traditionally debated, but evidence shows some invertebrates like octopuses experience pain: Octopuses avoid places where they previously experienced harm, demonstrating contextual learning. In experiments, injections of acetic acid caused site grooming, which was reduced when lidocaine (a local anesthetic) was applied. This indicates not just nociception but a higher-level processing of pain. Components of Pain Symptom vs. Illness: Pain can serve an adaptive role by alerting the body to injury or potential harm (e.g., withdrawing from a hot surface). Chronic or maladaptive pain (e.g., neuropathic pain) can negatively affect physical, social, and psychological well-being. Verbal and Non-Verbal Expression: Pain communication varies. Humans often describe it verbally, while animals and non verbal humans express it through behavior or physiological responses. An inability to communicate does not negate the experience of pain, emphasizing the importance of indirect observation for assessment. Learning: Pain perception is influenced by prior experiences. For example: A child learns to avoid sharp objects after a painful experience. Animals can associate specific environments with painful stimuli, avoiding those environments later. Subjective Experience: Pain is personal, shaped by: Biological Factors: Genetic predisposition, nervous system sensitivity. Psychological Factors: Stress, anxiety, or depression. Social Factors: Support systems or isolation. The Pain Matrix Pain processing involves a network of brain regions rather than a single "pain center." This interconnected system includes: Sensory/Discriminative Pathway: Key regions: Thalamus, Primary (SI) and Secondary (SII) Somatosensory Cortex. Function: Determines pain’s location, intensity, and duration. Affective/Motivational Pathway: Key regions: Anterior Cingulate Cortex (ACC), Insula (INS). Function: Assigns emotional value to pain, such as distress or discomfort. Cognitive/Evaluative Pathway: Key regions: Dorsolateral Prefrontal Cortex (dlPFC). Function: Modulates pain perception based on attention, prior experiences, and expectations. Pain Pathway Physiology The transmission and modulation of pain occur in distinct stages: Peripheral Nociception: Nociceptors in tissues detect harmful stimuli (e.g., thermal, mechanical, chemical). Signals travel via: A-delta fibers: Myelinated, fast-conducting fibers for sharp, localized pain. C-fibers: Unmyelinated, slower-conducting fibers for dull, aching pain. Central Processing: Signals from the peripheral nerves synapse in the spinal cord's dorsal horn. Ascend through pathways like the spinothalamic tract to the brainstem and thalamus. Relayed to cortical areas for higher-level processing. Cognitive/Evaluative Pathway: Key regions: Dorsolateral Prefrontal Cortex (dlPFC). Function: Modulates pain perception based on attention, prior experiences, and expectations. Pain Pathway Physiology The transmission and modulation of pain occur in distinct stages: Peripheral Nociception: Nociceptors in tissues detect harmful stimuli (e.g., thermal, mechanical, chemical). Signals travel via: A-delta fibers: Myelinated, fast-conducting fibers for sharp, localized pain. C-fibers: Unmyelinated, slower-conducting fibers for dull, aching pain. Central Processing: Signals from the peripheral nerves synapse in the spinal cord's dorsal horn. Ascend through pathways like the spinothalamic tract to the brainstem and thalamus. Relayed to cortical areas for higher-level processing. Descending Modulation: The brain can amplify or suppress pain signals through descending pathways involving: Periaqueductal Gray (PAG): Releases endorphins and enkephalins, natural painkillers. Neurochemicals like serotonin, noradrenaline, and dynorphin. Neurochemical Mediators of Pain Pain Initiators: Central Mediators: Glutamate: Excitatory neurotransmitter in spinal and brain circuits. Substance P: Amplifies pain signals in the central nervous system (CNS). Peripheral Mediators: Bradykinin and Prostaglandins: Produced at injury sites, sensitizing nociceptors. Pain Inhibitors: Endogenous chemicals modulate or block pain signals: Serotonin: Modulates pain pathways. Endorphins and Enkephalins: Bind to opioid receptors to inhibit pain perception. Dynorphins: Play a role in stress-induced analgesia. Nociceptive Circuit Modulation Pharmacological agents influence pain circuits: Opioid Agonists: Mimic endorphins, reducing pain perception (e.g., morphine). NMDA Antagonists: Block glutamate receptors, reducing central sensitization (e.g., ketamine). Alpha-2 Adrenergic Agonists: Reduce neurotransmitter release in pain pathways (e.g., clonidine). Antiprostaglandins: Inhibit prostaglandin synthesis to decrease peripheral sensitization (e.g., NSAIDs). Pleasant Pain and Cognitive Modulation Example: Capsaicin in hot peppers triggers nociceptors, causing a burning sensation without actual harm. Once the brain "learns" this is not harmful, the sensation becomes enjoyable, illustrating how cognitive evaluation influences pain perception. 1. Do Animals Feel Emotions? This question addresses a philosophical and scientific debate: whether animals experience emotions in ways comparable to humans. Recent research supports the idea that many animals, including rodents, exhibit measurable emotional responses. Scientific Background What are Emotions in Animals? Emotions are internal states that are typically characterized by physiological, behavioral, and neural responses to stimuli. For humans, emotions include subjective feelings (joy, sadness, pain). Animals cannot report subjective experiences, so scientists rely on observable proxies. Observable Emotional Cues in Rodents: Behavioral Indicators: Changes in grooming, freezing, exploratory behavior, and social interactions. Physiological Responses: Heart rate, stress hormone levels (e.g., corticosterone), and changes in respiration. Facial Expressions: Mice and rats exhibit distinct facial expressions, particularly in response to pain or distress. Supporting Research (Science 2020): This study analyzed facial expressions, behavioral patterns, and possibly neural activity to confirm emotional expressions in rodents. It reinforced that emotions, including pain and distress, have evolutionary roots and are shared across species. Ethical Implications: Recognizing that animals feel pain and emotions compels us to improve their treatment in laboratories, farms, and homes. Laws such as the 3Rs (Replace, Reduce, Refine) for animal research hinge on such findings to minimize suffering. 2. Facial Expression of Acute Pain This slide discusses how the pain experienced by rodents is captured, analyzed, and quantified. The methodology involves combining videography, computational learning, and brain imaging. Acute Pain in Rodents Pain Responses: Acute pain triggers immediate behavioral and physiological changes. Rodents react with tail-flicking, vocalizations, and distinct facial expressions when subjected to noxious stimuli like a tail shock. Facial Expressions as Pain Indicators: Specific Changes in Features: Eyes: Narrowing (orbital tightening). Nose: Bulging or changes in shape (nostril widening). Ears: Altered position or rotation backward. Whiskers: Flattening against the face or forward orientation. These specific changes form the "prototypic pain expression." Analysis Process: Videography: High-resolution cameras capture the rodent’s facial expressions during acute pain. Every frame is analyzed for subtle changes in the facial musculature. Computer Learning: Machine learning algorithms compare the observed facial expression with a database of known expressions. Pearson’s Correlation (r): This statistical measure calculates the similarity between the current expression and the prototypic pain expression. A value close to 1 indicates high specificity for pain. Brain Imaging: Techniques like fMRI or calcium imaging are used to identify neural circuits activated during pain. Regions such as the somatosensory cortex, insular cortex, and thalamus likely show increased activity, correlating with observed pain expressions. Importance of Specificity: Ensures that the observed expression is directly linked to pain and not confounded by other states like fear or fatigue. Advancements in Pain Research: Why Facial Analysis Matters: It provides a non-invasive, reliable method to assess pain severity. Unlike subjective behavioral tests, facial analysis is quantifiable and reproducible. Applications: Developing pain-relieving drugs. Evaluating the efficacy of treatments in preclinical models. 3. Empathy in Mice Empathy refers to the ability to perceive and share the emotional or sensory experiences of another. This slide explores groundbreaking evidence that mice demonstrate empathy through social transmission of pain and fear. What is Social Transmission? Definition: Social transmission occurs when an emotional or sensory state (e.g., pain or fear) in one individual is communicated to and experienced by another. In mice, this could occur through behavioral cues, olfactory signals, or other sensory modalities. Experimental Evidence: Pain Transmission: When a mouse experiencing pain (e.g., after an injection or injury) interacts with a cage mate, the second mouse often exhibits signs of distress, even without direct exposure to the painful stimulus. Mechanism: Olfactory Cues: Chemicals like pheromones signal distress. Visual Signals: Behavioral changes such as limping or grooming intensity may alert other mice. Auditory Cues: Ultrasonic vocalizations may communicate discomfort. Fear Transmission: Mice exposed to a predator or an aversive stimulus (like a loud noise) display fear responses such as freezing. Cage mates observing this behavior often freeze too, despite not being directly exposed to the threat. Neural Mechanisms: Brain Areas Involved: Anterior Cingulate Cortex (ACC): Central to empathy and social pain in both humans and rodents. Amygdala: Processes fear and emotional salience. Somatosensory Cortex: Contributes to vicarious pain perception. Plasticity: Synaptic changes in these regions may strengthen the capacity for empathy over repeated exposures. Science 2021 Study: This paper demonstrated that mice could "share" pain through specific neural pathways. Likely combined tools such as: Optogenetics: To manipulate neurons involved in empathy. Calcium Imaging: To visualize real-time neural activity during social interactions. Implications: Evolutionary Perspective: Empathy may enhance survival in social species by promoting cooperation and group cohesion. Recognizing distress in others allows rapid responses to potential threats or injuries. Animal Welfare and Research: Understanding social transmission of pain could improve how animals are housed and treated. It underscores the importance of minimizing stress in laboratory environments. Relevance to Humans: These findings provide models for studying empathy-related disorders (e.g., autism spectrum disorder, psychopathy). Insights into the neural basis of empathy can inform treatments for social dysfunction. Why This Research Matters Advances in Pain Assessment: Tools like videography and machine learning refine how we measure and interpret pain in animals. These methods improve the reliability of preclinical studies, ensuring better translation to human medicine. Understanding Empathy: The discovery of empathy-like behaviors in rodents bridges the gap between animal and human studies. It highlights that empathy is not exclusive to humans but rather a shared trait with deep evolutionary roots. Ethics and Welfare: Demonstrating emotional and empathetic capacities in animals mandates higher ethical standards. Researchers must consider how laboratory conditions impact animal well-being and social behaviors. Slide 22: The Brain Modulates Pain Overview: Pain is a subjective experience heavily regulated by the brain. The idea that "no brain, no pain" emphasizes that pain isn’t just a reflexive response to injury or nociceptive signals; it requires processing by the central nervous system (CNS). The brain integrates sensory, cognitive, and emotional inputs to create the subjective perception of pain. This means pain is not just a physical sensation but a complex interaction of psychological and physiological factors. Affective State (Emotions): Emotions significantly impact how pain is perceived: Negative emotional states such as fear, anxiety, stress, or depression can amplify the sensation of pain. This amplification occurs due to increased activity in brain regions like the amygdala and prefrontal cortex, which are involved in emotional processing and stress response. Positive emotional states like happiness, comfort, or relaxation can reduce pain. Positive emotions activate brain areas such as the nucleus accumbens and ventral striatum, which are part of the brain's reward and pleasure systems, dampening the pain response. Example: A person in a good mood may describe a painful event as "not so bad," while someone experiencing stress may find the same event excruciating. Cognitive Processes: Cognitive factors like attention, expectation, and memory play pivotal roles in pain perception: Attention: Focusing on pain heightens its perception, as attentional resources are directed toward the nociceptive input. Conversely, diverting attention away (e.g., by engaging in a task or using distraction) reduces the perception of pain. This is due to competition for processing capacity in the anterior cingulate cortex (ACC) and parietal lobes. Expectation: If pain is anticipated (e.g., knowing an injection is coming), the brain primes itself for pain, increasing sensitivity. Conversely, a lack of expectation can reduce pain. Memory: Past experiences of pain can alter future perceptions. A person who remembers a painful procedure vividly may experience heightened anxiety and amplified pain during a similar procedure. Cognitive and emotional factors interact dynamically. For example, anxiety (emotional) focuses attention on pain (cognitive), creating a feedback loop that intensifies the experience. Slide 24: The Role of Distraction in Pain Management Brain’s Processing Capacity: The brain is a finite processor and cannot handle all incoming stimuli with equal efficiency. When the brain is engaged in multiple tasks, the processing of one task (e.g., pain signals) becomes less efficient. Pain perception involves the somatosensory cortex, insula, and ACC. Distraction diverts these regions’ activity to other tasks, reducing the neural resources available for pain processing. Application of Distraction in Humans: Distraction is a well-documented method to reduce pain perception in medical settings. For example: During pediatric immunizations, children are often distracted with toys, videos, or engaging conversations, which significantly reduce their focus on the painful procedure. Distraction techniques leverage the gate control theory of pain, which proposes that non- painful stimuli (e.g., sensory input from distraction) close the neural "gate" to pain signals, inhibiting their transmission to the brain. Novel Application in Animals: The use of distraction in animals is a relatively new concept. Animals, like humans, experience reduced pain when their attention is drawn to something else. Effective distractors include: Tactile stimulation: Actions like tickling or gentle tapping activate mechanoreceptors in the skin, which compete with nociceptive input. Cognitive distractions: Offering treats (e.g., food) redirects the animal's attention away from the painful stimulus. This works because the brain’s reward pathways are activated, reducing the salience of pain. Additionally, distractions can serve as positive reinforcement, creating an association between procedures and rewarding experiences. Slide 25: Minimizing Anxiety During Handling Impact of Handling on Emotional State: Handling methods can influence an animal’s anxiety levels and, consequently, their perception of pain: Tail handling causes significant stress and aversion because it is perceived as a predatory act. Elevated stress levels activate the hypothalamic-pituitary-adrenal (HPA) axis, leading to the release of stress hormones like cortisol, which sensitize pain pathways. Tunnel handling or using an open-hand approach reduces stress because these methods are non-threatening. Animals voluntarily approach and engage with handlers, resulting in lower anxiety levels. Link Between Anxiety and Pain: Stress and anxiety amplify pain perception through increased activation of the amygdala, which enhances pain-related neural activity in the spinal cord and brainstem. Conversely, low-anxiety states (e.g., induced by gentle handling) dampen pain signaling, likely by activating the periaqueductal gray (PAG), a key region for descending pain inhibition. Slides 28–29: Comparing Handling Methods Standard Method (Tail Handling): First Injection: Tail handling induces high aversion, as the animal associates it with a threat. Restraint causes mild stress, but this is manageable at first. The injection itself causes pain. Second Injection: The tail-handling process triggers even higher aversion due to the animal’s memory of the first injection. Restraint now induces high stress due to the anticipation of pain. This heightened stress activates the HPA axis, releasing cortisol and amplifying the perception of pain. The injection is perceived as more painful, partly due to the combined effects of stress and memory of previous discomfort. Refined Method (Tunnel Handling): First Injection: Tunnel handling eliminates aversion because the animal perceives the tunnel as a safe environment. Restraint still induces mild stress, but this is lessened by the animal’s sense of control and security. The injection causes pain, but the introduction of a reward (e.g., Nutella or tickling) activates the brain’s reward system, offsetting the discomfort. Second Injection: The absence of aversion continues because the tunnel method remains consistent. Restraint causes only mild stress because the animal does not anticipate pain due to the positive experience of the first injection. Pain perception is mitigated further by the expectation of a reward, which reduces anticipatory anxiety. Broader Implications: Animal Welfare: Refining handling techniques reduces animal suffering and enhances the reliability of experimental results, as stress-induced variability in behavior and physiology is minimized. Neurobiological Basis: Understanding the brain regions involved in pain and emotional processing (e.g., amygdala, PAG, ACC) provides insight into how handling techniques and distractions influence pain perception at a neural level. Practical Recommendations: Transitioning to refined handling methods (e.g., tunnels) and incorporating rewards can transform painful procedures into manageable experiences for animals, fostering better compliance and welfare. Core Concept: How People are Concerned About Animal Pain This explores the nuances of empathy and recognition of pain in others, emphasizing both human and animal contexts. Let’s break this into layers: Recognizing the Pain of the Other Familiarity as a Factor: Pain recognition is influenced by social closeness. This means individuals are biologically and psychologically primed to be more attuned to the suffering of those they know personally. Underlying Mechanisms: Mirror neurons may play a role. These neurons activate when observing others in pain, especially when the person is familiar. The emotional bond strengthens the intensity of this mirrored response. Evolutionarily, this makes sense because recognizing pain in family or close social circles could have survival advantages, ensuring group cohesion and care. Strangers and Stress: When dealing with strangers, the stress response can dominate over empathy: Endogenous Corticosteroids: These are stress hormones (e.g., cortisol) that prepare the body for "fight or flight" but suppress complex emotional responses, including empathy. In high- stress situations, individuals prioritize self-preservation over empathizing with strangers. Stress-induced empathy reduction is an adaptive mechanism to manage emotional overload in unfamiliar or threatening contexts. Slide 2: Empathy and Pain Recognition Core Concept: Empathy’s Role and Limitations Definition of Empathy: Empathy involves: Affective Empathy: The ability to feel another's pain or emotion as if it were your own. Cognitive Empathy: The ability to understand another's emotional state intellectually, without necessarily feeling it. Stress as a Limiting Factor: Why Stress Reduces Empathy: Stress diverts cognitive and emotional resources to self-regulation and survival instincts. Neurologically, the prefrontal cortex (involved in empathy and decision-making) is less active during stress, while the amygdala (associated with threat detection) becomes dominant. Implications: In environments like hospitals or emergency settings, the stress experienced by caregivers can lead to a reduced ability to connect emotionally with patients, especially those perceived as strangers. Slide 3: Emotional Contagion and Stress Core Concept: How Pain Spreads Emotionally Emotional Contagion: This refers to the subconscious mirroring of another's emotions or pain, creating a shared emotional experience. In Familiar (Cage) Mice: Mechanism: Familiarity fosters trust and emotional openness, making emotional contagion stronger among cage mates. Stress Inhibition: Stress acts as a physiological barrier to this shared emotional response. Elevated stress hormones suppress the neural circuits that enable emotional mirroring, like those in the anterior insula and cingulate cortex. In Foreign Mice: Stress Modulation: Reducing stress can allow emotional contagion to occur even among unfamiliar individuals. Applications to Humans: This shows that creating stress-free environments can enhance empathy and emotional connections, even among strangers. Slide 4: Racial Bias in Pain Perception Core Concept: Disparities in Pain Recognition and Treatment Evidence of Racial Bias: Afro-American patients receive fewer and lower-dose analgesics compared to white patients. This disparity persists despite similar levels of reported pain. Underlying Factors: Implicit Bias: Healthcare professionals may subconsciously underestimate the pain experienced by Black patients, influenced by stereotypes (e.g., that Black individuals have higher pain thresholds). Historical Context: Misinformation dating back to slavery-era pseudo-science, such as claims that Black individuals were less sensitive to pain, persists in subtle ways today. Consequences: Health Inequities: Untreated pain can lead to chronic conditions, lower quality of life, and mistrust in healthcare systems. Opioid Epidemic: While Black patients may receive fewer opioids, the disproportionate prescribing to white populations has contributed to widespread addiction, showing how biases have complex societal impacts. Statistics: Pain-related complaints are some of the most common reasons for emergency visits, highlighting the importance of equitable pain management. Slide 5: Species Bias in Pain Empathy Core Concept: Empathy Towards Animals Varies by Species Empathy and Phylogenetic Distance: Findings: Empathy decreases as the evolutionary (phylogenetic) distance from humans increases. Graph Interpretation: Dogs and cats, being domesticated and close companions, evoke higher empathy. Species like reptiles or insects, which are evolutionarily distant and culturally unfamiliar, evoke less. Veterinary Practice Example: Survey results indicate dogs receive more analgesia during surgery compared to cats. Misconceptions like "cats are less sensitive to pain" reflect cognitive biases rather than scientific evidence. Slide 6: Empathy for Laboratory Animals Core Concept: Challenges in Empathy for Research Subjects Why Laboratory Animals are Perceived as "Strangers": Laboratory animals are often seen as tools for research rather than beings capable of suffering. This perception is reinforced by historical attitudes and practical detachment in research environments. Improving Pain Assessment: Reliance on objective measures of pain (e.g., behavioral scoring systems, physiological markers) can counteract subjective biases and improve animal welfare in research. Extended Applications: For Healthcare: Reducing stress for caregivers can improve empathy towards patients, especially those from marginalized groups or unfamiliar backgrounds. Training programs addressing implicit biases can reduce racial disparities in treatment. For Animal Welfare: Raising awareness about species-specific pain responses and the limitations of anthropocentric empathy can lead to better treatment across all species. Objective pain assessment tools in veterinary and laboratory settings ensure more equitable treatment. Challenges in Assessing Pain in Animals: Absence of Verbal Communication: Humans express pain through words, providing clarity about their suffering. Animals, however, lack this ability, forcing researchers, veterinarians, and caretakers to rely entirely on indirect indicators. The rhetorical statement, “They do not speak = they do not suffer?”, challenges the misconception that pain cannot exist without verbalization. This is an ethical dilemma that requires us to rely on indirect evidence of pain. Indirect Methods of Determination: Asking: In the context of humans, we ask directly to gauge pain. With animals, "asking" translates into interpreting their behaviors, body language, or physiological responses to infer their state. Observing: Systematic observation of behaviors and physical changes becomes essential. This involves understanding species-specific responses, as these vary across animals (e.g., a hunched posture in rats vs. hiding in rabbits). Philosophical and Ethical Implications: Assessing pain in animals is not just a scientific challenge but an ethical necessity. If pain is ignored due to lack of understanding or tools, animals may suffer unnecessarily. This underpins the importance of developing accurate pain assessment techniques. Slide 2: Causes of Suboptimal Use of Painkillers Why Pain Management in Animals Can Be Inadequate: Lack of Awareness: Many researchers or caregivers may not fully recognize the signs of pain, especially subtle ones. Misinterpretation of behavior (e.g., assuming aggression is a natural trait rather than a sign of discomfort) can lead to inadequate analgesia. Fear of Side Effects: Painkillers can sometimes cause side effects, such as sedation, loss of appetite, or other physiological impacts. In experimental settings, this may interfere with study results, causing reluctance in their administration. Limited Research and Validation: Tools for assessing pain and the efficacy of painkillers in animals are often underdeveloped or poorly validated. This can lead to reliance on outdated methods. Ethical Oversight: Institutional and regulatory bodies sometimes impose strict rules about analgesic use, prioritizing the avoidance of interference with scientific results over animal welfare. Slide 3: Indicators of Pain in Common Laboratory Animals Species-Specific Signs of Pain: Each animal exhibits pain differently, and understanding these signs requires familiarity with their natural behavior and physiology. The table presented outlines key indicators: Rats: Behavioral Indicators: Reduced activity and reluctance to explore. Self-mutilation: Rats may excessively groom or bite themselves as a response to pain. Increased vocalizations and aggression: Pain may make rats more irritable, leading to defensive behaviors. Appearance: Ungroomed fur and piloerection: These are signs of poor well-being, often caused by stress or discomfort. Hunched posture (described as "dormouse posture"): This indicates abdominal or systemic pain. Porphyrin secretion ("red tears") around the eyes and nose: A stress-induced response, commonly mistaken for blood. Physiological Indicators: Sleep disruption: Pain interferes with normal rest cycles. Hypothermia: A drop in body temperature is a physiological stress response. Breathing patterns: Pain can cause rapid and shallow respiration or grunting, particularly during expiration. Mice: Display similar behaviors and physical signs as rats. However, they do not exhibit ocular porphyrin secretion, making other signs (e.g., posture, activity levels) more critical for detection. Guinea Pigs: Squealing and stampeding when handled are common signs of distress. Their physiological response mirrors that of rats but may also include unique vocalization patterns. Rabbits: Pain makes rabbits anxious and reclusive, often leading them to hide. Tonic immobility (freezing) is a common stress response that can mask signs of pain. Physiological indicators like salivation and shallow breathing may occur, but rabbits are less likely to exhibit dramatic physical changes than rodents. Slide 4: Pain Assessment in Rodents Pain Assessment Methods: Simple Descriptive Scale (SDS): Categorizes pain into qualitative levels: No Pain: The animal behaves and looks normal. Mild Pain: Subtle signs such as flinching during palpation. Moderate Pain: Clear behavioral and physiological changes (e.g., hunched posture, reduced activity). Severe Pain: Obvious and persistent distress (e.g., vocalizations, rapid breathing). Unbearable Pain: Continuous, violent responses indicating extreme suffering. Strength: Easy to implement in routine assessments. Limitation: Highly subjective and dependent on observer experience. Visual Analog Scale (VAS): Pain is assessed along a continuum marked on a scale (e.g., from 0 = no pain to 10 = maximum pain). Requires observers to estimate the pain intensity, making it slightly more quantitative than SDS. Numerical Rating Scale (NRS): Observers assign a numerical value to the pain intensity, often based on predefined criteria (e.g., 0 = no pain, 5 = moderate pain, 10 = unbearable pain). Composite Scales: Examples include the Glasgow, Colorado, and Melbourne Pain Scales. Combine behavioral, physiological, and appearance-related factors into a single score. Comprehensive but require training for proper use. Slide 5: Specific Pain Scales and Indicators Detailed Examples: Rat-Specific Pain Scale: Focuses on acute behavioral responses: Lumbar cutaneous muscle contractions: A reflexive response to abdominal pain. Back arching and wound licking: Signs of discomfort from surgical procedures. Timing of behaviors: Typically assessed within 5 minutes post-surgery to gauge immediate pain levels. Facial Grimace Scales: Developed to assess pain based on changes in facial expressions (e.g., narrowing of the eyes, flattening of the cheeks). These are objective, repeatable, and less invasive than traditional methods. Example: The "Mouse Grimace Scale" is widely used in research for evaluating pain intensity. Slide 6: AI in Pain Assessment for Cats Artificial Intelligence (AI) Applications: Overview: AI systems analyze behavioral and physiological data to detect pain in animals automatically. These systems reduce subjectivity and increase the accuracy of assessments. Applications in Cats: Facial recognition algorithms can detect subtle changes in expressions (e.g., squinting, lip tension). Movement tracking systems analyze posture and activity levels to identify deviations caused by pain. 3. Future Potential: AI could revolutionize pain assessment by providing real-time monitoring and reducing the reliance on human observers. Integration with veterinary practices and animal research could lead to more humane and precise care. This slide sets the foundation for understanding pain management, particularly in research and clinical settings involving animals. Analgesic Efficacy and Strain Variability Definition: Analgesic efficacy refers to the ability of a drug to reduce or eliminate pain. Strain variability highlights how genetic differences between strains (e.g., of rodents) affect their physiological and pharmacological responses to drugs. Strain Variability and Its Implications: Rodents of different genetic backgrounds require different doses of the same analgesic for equivalent pain relief. Example: Some rat strains might metabolize analgesics more efficiently, leading to reduced drug levels at pain sites, while others might have heightened receptor sensitivity, needing less drug for the same effect. Research Evidence: Morgan et al. (1999): This study found that response variability to μ-opioids (e.g., morphine) depends on: Strain genetics: Some rat strains have a higher density or sensitivity of μ-opioid receptors. Stimulus intensity: High-intensity pain may require higher drug doses regardless of strain. Intrinsic efficacy: The ability of a drug to activate receptors fully (some drugs are partial agonists and may not fully activate receptors, leading to different effects). This implies that standardizing drug doses without accounting for strain-specific differences can lead to inadequate pain management or overdose. Key Takeaway: Analgesic therapy cannot be generalized across populations. Precision medicine is essential, even in preclinical animal studies. Dispelling the Myth: "Analgesics Always Provide Analgesia" Why This is a Myth: Pain is a complex physiological and psychological experience. It involves nociception (detection of painful stimuli by sensory neurons) and central processing in the brain. Analgesics target specific points in the pain pathway but might not address all aspects of pain, such as emotional distress or chronic pain mechanisms (e.g., central sensitization). Practical Implication: Pain management protocols must go beyond drug administration. They should include: Regular assessment of pain levels. Adjustments based on efficacy. Non-pharmacological interventions when necessary. Ideal Analgesic Therapy Matching Analgesics to Procedure Severity: Pain severity varies with procedure type. For instance: Minor procedures: NSAIDs may suffice to control inflammation and mild pain. Major surgeries: Opioids are often necessary due to their potency in controlling severe pain. Example: In abdominal surgery on mice, NSAIDs like meloxicam can address postoperative inflammation, but an opioid like buprenorphine may be required for immediate, severe pain relief. Assessing Analgesic Efficacy: Regular pain assessments are crucial. Techniques include: Behavioral observation: Changes in grooming, posture, or activity levels. Physiological measures: Heart rate or cortisol levels as stress indicators. If the analgesic is ineffective: Increase the dose or frequency. Switch to a more potent drug or combine analgesics. Re-evaluating Therapy: Pain is dynamic, especially post-surgery, as healing progresses. A static analgesic plan might lead to over- or under-treatment. Frequent re-assessment ensures proper management. Types of Analgesics NSAIDs (Non-Steroidal Anti-Inflammatory Drugs) Mechanism of Action: NSAIDs inhibit cyclooxygenase (COX) enzymes, which are crucial for the production of prostaglandins. Prostaglandins: Lipid compounds that sensitize nerve endings to pain and cause inflammation. By reducing prostaglandins, NSAIDs alleviate both pain and inflammation. Advantages: Long duration of action. Anti-inflammatory effects are beneficial for surgical recovery. Limitations: Potential side effects like gastrointestinal irritation and kidney damage, especially with prolonged use. Opioids Mechanism of Action: Bind to opioid receptors in the brain and spinal cord, inhibiting pain signal transmission. Main types of opioid receptors: μ-opioid receptor: Primary target for most analgesics like morphine and fentanyl. κ- and δ-opioid receptors: Contribute to pain relief but also produce side effects like dysphoria or sedation. Advantages: Highly effective for severe pain. Rapid onset of action. Limitations: Side effects: Respiratory depression, tolerance, and dependence. Overdose risk requires careful dose adjustment. Other Analgesics: Drugs like gabapentin target neuropathic pain, which is less responsive to traditional NSAIDs or opioids. Recommendations for Pain Management Preventive Analgesia: Giving analgesics preoperatively prevents central sensitization, where the nervous system becomes hyper-responsive to pain. Example: Pre-surgery meloxicam reduces postoperative inflammation and prevents exaggerated pain responses. Polymodal Analgesia: Combining drugs with different mechanisms maximizes pain relief while minimizing side effects. Example: NSAID + opioid = Reduced inflammation and potent central pain control. Frequent Pain Assessment: Pain scales adapted for animals (e.g., Grimace Scale for rodents) help in regular evaluation. Adjust drugs and doses as needed. Comprehensive Pain Relief Approach Pain relief requires a holistic approach that addresses environmental, procedural, and pharmacological factors: Environment: Stress and poor handling exacerbate pain perception. Ensuring minimal stress improves overall outcomes. Anesthesia-Analgesia: Sedation during surgery avoids immediate nociceptive responses. Post-surgery analgesia ensures recovery is pain-free. Procedure/Surgery: Minimally invasive surgical techniques and perioperative care reduce the need for high-dose analgesics. Conclusions Pain Must Be Relieved: Pain is a welfare issue. Managing it effectively involves selecting the right drug, dose, and administration timing. Assessment is Key: Pain is dynamic. Continuous reassessment ensures therapy adapts to the animal's needs. Beyond Drugs: Non-pharmacological measures (refined techniques, stress reduction) are equally important for comprehensive pain relief. Legislation 1. Quality Assurance in Animal Experimentation Quality assurance ensures that any use of animals in experiments complies with ethical, legal, and scientific standards. This involves: Adherence to Legal Standards: Following laws like Directive 2010/63/EU, which provides a framework to protect animal welfare in scientific contexts. Ethical Principles: The "3Rs" principle (Replace, Reduce, Refine) serves as a foundation for minimizing the use and suffering of animals. Replace: Use alternatives like cell cultures or computer models when possible. Reduce: Minimize the number of animals used without compromising statistical validity. Refine: Enhance procedures to reduce pain and stress. This system ensures that: The experiments are scientifically justified. They are performed only when no alternatives exist. The conditions for the animals are humane and legally compliant. 2. Learning Outcomes This section outlines the knowledge and competencies expected from participants, focusing on laws, procedures, and roles in animal experimentation. 2.1 Identify and Describe National and EU Laws The regulatory framework for animal experimentation ensures: Uniform protection across member states (via Directive 2010/63/EU). The foundation for permits, ethical review processes, and compliance inspections. Key points: Directive 2010/63/EU: Updates and replaces Directive 86/609/EU, setting modern standards for welfare and experimental ethics. National Laws: Each country transposes the EU Directive into national laws, e.g., the Dutch Experiments on Animals Act. 2.2 Identify Related Animal Welfare Legislation Animal welfare laws often extend beyond laboratory use: Health and Welfare Law for Animals (1992): General welfare of animals, including pets and livestock. Law on Animals (2013): Covers transport, housing, and handling. 2.3 Authorization Authorization ensures ethical oversight: Institutional Level: Researchers and facilities need licenses. Project Level: Every study involving animals must pass ethical reviews and be approved. 2.4 Information Sources Key resources include: National and EU legal databases (e.g., wetten.overheid.nl for Dutch laws). Guidance from local animal welfare bodies and national committees. 2.5 Roles of Personnel (Articles 24-26) Articles 24-26 of Directive 2010/63/EU define responsibilities for: Breeders, Suppliers, and Users: Licensing and compliance. AWOs and Committees: Provide ethical oversight, training, and welfare monitoring. 2.6 Roles of Local and National Committees Local Animal Welfare Bodies (AWBs): Support researchers in planning humane experiments. Monitor animal welfare within institutions. National Committees: Provide guidance on ethical dilemmas and best practices. Ensure harmonized implementation of Directive 2010/63/EU. 2.7 Compliance Responsibility Institutions bear ultimate responsibility for compliance, often through the AWB. Mechanisms include: Regular audits. Training programs. Incident reporting systems. 3. Legal Framework The legal framework exists to regulate the use of animals in scientific research while ensuring ethical and humane treatment. 3.1 Intent of Legislation Legislation aims to: Protect animals as sentient beings (Intrinsic Value – Art. 1a). Limit experiments unless justified ("No, unless..." – Art. 1d). Promote 3R principles. 3.2 Historical Context UK (1876): The Cruelty to Animals Act was the first major legislation to regulate animal experimentation. Netherlands (Timeline): 1886: Criminal code penalized general animal cruelty. 1977: The Dutch Experiments on Animals Act formalized regulations. 2014: The revised Act aligned with modern EU standards (Directive 2010/63/EU). 3.3 Current Legislation Experiments on Animals Act: Core Dutch legislation for laboratory use. Experiments on Animals Decree and Regulation: Detail the implementation of the Act. Directive 2010/63/EU: Harmonizes animal welfare standards across the EU. 4. Other Relevant Legislation This section highlights laws indirectly related to animal experimentation. 4.1 Law of Nature Preservation Field experiments involving wildlife must adhere to: Species protection measures. Special permits for field studies. 4.2 Law on Animals Covers multiple aspects of animal care, including: Transport: Ensures humane conditions during transportation. Weaning and Handling: Regulates practices like early weaning to minimize stress. Housing Standards: Decree on keepers of animals sets strict housing requirements. 4.3 Law on Environmental Pollution This includes regulations on: GMO Research: Ensures safe use of genetically modified organisms in experiments. 5. Execution of Animal Experiments 6. Final Registration 5.1 Definition After an experiment: Results are reported to authorities. An animal experiment involves procedures performed on live animals to: Compliance with permits and ethical requirements is documented. Study biological phenomena. Long-term impact assessments may be Test medical devices or treatments. conducted. Fulfill regulatory requirements (e.g., drug safety testing). 5.2 Parties Involved Researchers: Plan and conduct experiments. Animal Welfare Officers: Oversee ethical and legal compliance. Institutional Committees: Review and approve projects. 5.3 Local Execution Io Housing: Animals must be provided with clean, spacious, and enriched environments. Discomfort: Researchers must minimize pain or distress and justify its necessity. Humane Endpoints: Predefined criteria determine when to terminate experiments to prevent undue suffering. Euthanasia: Procedures must be swift, painless, and follow regulatory guidelines. Slide 10: Definition of Animal Experiment The slide provides a legal definition of an animal experiment, breaking it into key components that determine whether a procedure involving animals falls under regulatory oversight. Let’s analyze this in extreme detail: 1. What is Defined as an Animal Experiment? An animal experiment, legally, includes: The use of an animal for scientific, experimental, or educational purposes: This includes activities designed to generate new knowledge (e.g., biomedical studies, drug testing) or teach (e.g., anatomy dissection). It applies regardless of the outcome: Known outcome: When the expected results are predictable (e.g., verifying a hypothesis). Unknown outcome: When the procedure is exploratory (e.g., testing a new treatment without certainty of its effects). Invasive and Non-Invasive Use: Invasive: Procedures that physically intrude into the animal's body (e.g., surgery, injections). Non-Invasive: Activities that do not physically penetrate the body but may still cause distress or suffering (e.g., behavioral studies under stressful conditions). Threshold for Inclusion: The procedure is classified as an animal experiment if it causes pain, suffering, distress, or lasting harmequivalent to or greater than a needle insertion. 2. The Pain Threshold: Why a Needle? The comparison to a needle insertion is significant: Benchmark for Pain: This is a minimally invasive procedure commonly encountered in veterinary care, making it a clear, standardized reference point. Universal Applicability: Using such a baseline ensures consistency across varying types of experiments and species. Subjectivity of Animal Pain: Since animals cannot verbally express their pain, scientists and regulators rely on observable signs (e.g., vocalizations, changes in behavior, physiological stress markers). 3. Scope of the Definition This definition ensures: Inclusivity: Covers a broad spectrum of procedures to avoid loopholes where harmful practices might escape regulation. Preventative Regulation: Any procedure that might potentially cause harm (even if uncertain) is regulated to err on the side of caution. This is critical for experimental contexts where risks may not yet be fully understood. 4. Ethical Implications The inclusion of non-invasive methods in this definition shows sensitivity to the subjective experience of animals. Even if a procedure seems benign to humans, it could cause significant distress to animals unfamiliar with such handling or environments. The emphasis on scientific and educational purposes ensures that procedures unrelated to legitimate goals (e.g., unregulated testing or entertainment) are excluded. Slide 11: The Three R’s – Restrictions on Animal Experiments Animal experiments are restricted unless they align with the Three R's. These principles were introduced in the 1950s by Russell and Burch and have since become a global standard in animal research ethics. 1. Replacement Objective: Avoid or replace the use of live animals where alternative methods are available. Examples: In vitro testing: Using cell cultures instead of whole animals to study biological processes. Computer models: Simulating drug interactions or disease progression computationally. Human-based methods: Utilizing human tissue samples, organ-on-chip technology, or volunteer studies. Challenge: Despite technological advances, complete replacement is not always possible due to the complexity of living systems. 2. Reduction Objective: Minimize the number of animals used without compromising the validity of the results. Strategies: Efficient Experimental Design: Use advanced statistical methods to obtain maximum data from fewer animals. Perform pilot studies to refine procedures before full-scale experiments. Sharing Data: Encourage collaboration to avoid duplicating experiments unnecessarily. Practical Example: Instead of testing a new drug on 50 animals, researchers might achieve comparable results using 20 animals with improved methodologies. 3. Refinement Objective: Enhance the procedures to minimize pain, suffering, or distress and improve animal welfare. Examples: Using better anesthetics and analgesics during surgery. Providing enriched environments (e.g., toys, companions) to reduce psychological stress. Training personnel to handle animals humanely and reduce anxiety-inducing interactions. Impacts: Refinement not only benefits animals but also improves the quality of scientific results, as stressed animals may produce unreliable data. Slide 12: Deviation from European Directive 1. European Directive on Animal Experiments Core Principle: Excludes certain procedures, such as killing animals solely for the use of their organs or tissues, from being classified as animal experiments. Reasoning: Such activities, while involving animals, are not intended to study living systems or cause distress during the experiment itself. 2. Dutch Interpretation The Netherlands imposes stricter rules: Inclusion: Killing animals to harvest organs or tissues is considered an animal experiment under the Dutch Experiments on Animals Act. Requirement: Researchers must obtain project authorization for these procedures, even if they fall outside the scope of the EU directive. 3. Implications of the Dutch Approach Ethical Rigor: By broadening the scope, Dutch law ensures greater oversight and accountability, preventing potential misuse of animal sacrifices. Administrative Burden: While it enhances animal welfare, this approach may increase bureaucratic complexity for researchers. Slide 15: Involved Parties and Responsibilities This slide outlines the organizational structure overseeing animal experimentation in the Netherlands. Let’s explore each entity in extreme detail. 1. Ministry of Agriculture Role: Governs the implementation of legislation related to animal experiments. 4. National Committee for the Authority: Ensures national compliance with EU directives and oversees the subordinate agencies. Protection of Animals Used for Scientific Purposes (NCad) Functions: 2. Central Authority for Scientific Procedures on Animals (CCD) Provides expert advice to policymakers, researchers, and Responsibilities: ethical committees. Ethical Review: Promotes best practices and Evaluates whether proposed experiments adhere to ethical standards and the Three R’s. innovation in ethical animal research. Balances scientific merit against animal welfare considerations. Facilitates communication between stakeholders to harmonize Project Licenses: approaches nationally and Approves specific research projects, ensuring all experiments are justified and meet ethical guidelines. Iointernationally. 3. Netherlands Food and Consumer Product Safety Authority (NVWA) Institutional Licenses: Grants licenses to institutions conducting animal experiments, ensuring they meet infrastructure and competency standards. External Supervision: Conducts inspections to monitor compliance with laws and ethical practices. Investigates complaints and reports of non-compliance. Annual Reporting: Requires institutions to document and submit details of their animal research activities annually. Slide 6: From Idea to Animal Experiment (Part 1) Article 2-8: Responsible Researcher Who is a Responsible Researcher? The responsible researcher is the person with ultimate accountability for the design, setup, and execution of an animal experiment or project. This role exists to ensure that the experiments meet high scientific, ethical, and regulatory standards. Qualifications: Master's Degree (MSc): A postgraduate qualification, typically obtained after a bachelor's degree, indicating advanced knowledge in a specific field. For this role, the MSc should focus on life sciences or closely related disciplines (e.g., biology, veterinary medicine, neuroscience). 500 Hours of Education in Basic Biology and LAS: Basic Biology: Fundamental understanding of biological principles like genetics, cell biology, physiology, and ecology. Ensures researchers have a robust knowledge base for interpreting results accurately and ethically. Laboratory Animal Science (LAS): A specialized course designed to teach ethical principles, regulations, and practical skills for working with laboratory animals. Includes modules on: Animal handling and care: Proper ways to handle animals to minimize stress or injury. Regulatory compliance: Laws and guidelines governing the use of animals in research. Humane endpoints (HEPs): Identifying when an experiment should stop to prevent unnecessary suffering. Species-Specific Knowledge: Why Species-Specific Knowledge is Essential: Each species used in research has unique needs and behaviors. For example: Rodents: Require enriched environments (e.g., tunnels, nesting materials). Primates: Need social interactions and cognitive stimulation. Fish: Sensitive to water quality and light conditions. Mismanagement can lead to stress, pain, or invalid results. What This Knowledge Covers: Behavioral Understanding: Recognizing signs of stress or pain (e.g., abnormal grooming in rodents, reduced appetite in primates). Anatomical and Physiological Insights: Knowing optimal sites for injection, blood collection, or drug administration. Understanding their metabolic rates to determine proper drug dosages. Importance of This Role: Acts as the ethical and scientific checkpoint. Balances research goals with animal welfare by: Ensuring methods are scientifically justified and ethically defensible. Minimizing unnecessary use of animals. Ensuring adherence to the 3Rs (Reduction, Refinement, Replacement), a cornerstone of animal research ethics. Slide 7: From Idea to Animal Experiment (Part 2) Article 9: Application for Project Licence Why is a Project Licence Required? The use of animals in experiments is strictly regulated because it involves ethical concerns about animal welfare. A licence is a legal document that: Authorizes researchers to conduct specific experiments. Confirms compliance with ethical and regulatory standards. Without this licence, the experiments are considered illegal and unethical. Contents of the Application: A. Non-Technical Summary (NTS): Written in Simple Dutch: Accessible to the general public, not just scientists. Example: Instead of "Neurogenesis in rodent hippocampi," say, "How brain cells grow in mice." Public Availability: Builds transparency and public trust. Demonstrates the importance of the research while addressing ethical considerations. B. Project Proposal: Background: Explains the scientific or societal issue the research addresses. Example: Developing treatments for Alzheimer’s or improving vaccine efficiency. Goal and Importance: Defines what the experiment aims to achieve and its potential impact. Example: If testing a new drug, the goal might be to confirm its safety and efficacy. Research Strategy: Describes the methods and procedures in detail to ensure replicability. Includes statistical designs to minimize the number of animals needed. C. Appendices for Animal Experiments: Details of Procedures: Step-by-step description of every experiment. Example: If inducing diabetes in mice, specify how and when. Number of Animals: A justification for the number used, balancing statistical power with ethical responsibility. Example: Use the power analysis technique to determine minimum sample size. Discomfort: Anticipated levels of pain or distress are classified (e.g., mild, moderate, severe). Humane Endpoints (HEPs): Criteria for stopping experiments early to prevent unnecessary suffering. Ethical Judgement: Evaluates whether: The potential benefits outweigh the costs to animal welfare. The study design minimizes harm and maximizes scientific validity. Slide 8: From Idea to Animal Experiment (Part 3) Article 2-8: Animal Welfare Body Structure: Composed of: Alternatives Experts: Specialists who propose non-animal methods. Designated Veterinarians: Experts in animal health and welfare. Scientists: Researchers familiar with the scientific aspects of the experiment. Responsibilities: Advisory Role: Provides guidance on: Regulatory compliance. Use of alternative methods (e.g., in vitro models, computer simulations). Example: Recommending cell culture studies to replace initial animal testing phases. Supervisory Role: Ensures: Protocol adherence. Timely adjustments to protect animal welfare during experiments. Articles 13F3 & 14: Balancing Priorities Animal Welfare: Ensures that housing, handling, and experimental conditions meet welfare standards. Scientific Quality: Checks the research methodology to confirm it will produce meaningful results. Slide 8: From Idea to Animal Experiment (Part 4) Article 18a: Competent Authority Centrale Commissie Dierproeven (CCD): Role: The final authority granting project licences after evaluating ethical and scientific reviews. Evaluation Criteria: Checks whether: The project adheres to national laws and EU regulations. The ethical review is thorough and unbiased. Granting Licences: Ethical Judgement: Performed by considering: Societal benefits: Is the research valuable enough to justify using animals? Adherence to the 3Rs. Relationship with Other Entities: Relies on recommendations from: Animal Welfare Bodies. Independent Animal Ethics Committees. Key Extensions: Why So Many Oversight Bodies? Research involving animals requires multiple levels of review to safeguard ethical standards and prevent misuse of animals. This system minimizes conflicts of interest while ensuring robust scientific outcomes. Challenges in Implementation: Balancing scientific ambition with animal rights is complex. Researchers must navigate regulatory frameworks and ethical debates while producing valid, reproducible results. Part 4: Law and Regulations 1. Intent of Legislation The overarching goal of animal experimentation laws is to align scientific progress with ethical responsibility. Key aspects include: Balance of Interests: On one hand, researchers need to use animals to understand biological processes, develop medicines, or test safety. On the other hand, society demands that animals are treated with dignity, minimizing their suffering. Public Accountability: These laws reassure the public that animal use in research is strictly regulated and ethical. Without such regulations, public trust in scientific institutions could erode. Ethical Frameworks: Laws reflect societal values about animal welfare. For example, animals are recognized as sentient beings capable of suffering, so regulations are designed to respect their intrinsic value beyond their utility in research. 2. Brief History Understanding the history of animal experimentation regulations highlights why current laws are so stringent: Pre-Regulation Era: Experiments were conducted without oversight, often leading to significant animal suffering. Public backlash against high-profile cases (e.g., LD50 tests where animals were poisoned to death) prompted reform. Milestone Legislations: Laws like the UK's Cruelty to Animals Act 1876 or the EU's Directive 2010/63introduced systematic controls on animal experiments, including requirements for ethical review and licensing. 3. What is an Animal Experiment? An animal experiment is defined as any procedure performed on a live vertebrate or cephalopod that could cause pain, suffering, distress, or lasting harm. Examples include: Biomedical Research: Testing new cancer drugs on mice to evaluate efficacy. Toxicity Testing: Ensuring chemicals in household products are safe. Behavioral Studies: Observing stress responses in social animals. From Idea to Animal Experiment 1. Articles 2-8: Working Protocol A working protocol is the blueprint for conducting an animal experiment. Each component ensures that ethical and scientific standards are met: Details of Experiments: Researchers must provide a step-by-step plan. For example: Hypothesis: "Does Drug X reduce inflammation?" Procedure: Administer Drug X to a test group of rats and monitor inflammation levels. Expected Outcome: Rats treated with Drug X will show reduced inflammation. Method of Housing: Animal welfare must align with guidelines such as: Cage Dimensions: Defined minimum sizes to prevent confinement stress. Enrichment: Toys, nesting materials, or running wheels for mental stimulation. Social Housing: Group-housing rats unless they are aggressive or require isolation for scientific reasons. Level of Discomfort: Discomfort levels are categorized (mild, moderate, severe), and steps to alleviate it must be outlined. Example: If a procedure causes moderate pain, the plan should include analgesics (painkillers). Humane Endpoints: Criteria for stopping experiments before significant suffering include: Observable weight loss beyond 20% of body weight. Behavioral signs such as lethargy or self-harm. Method of Killing: Killing must be humane, such as: Overdose of Anesthetic: Ensures unconsciousness and death without pain. CO₂ Inhalation: Only under carefully controlled conditions to minimize distress. Agreements and Responsibilities: The protocol specifies: The principal investigator’s role (e.g., ensuring ethical compliance). Responsibilities of veterinary staff (e.g., assessing animal health daily). Amendments: If research objectives change (e.g., additional tests are needed), the protocol must be revised and reapproved by the ethics committee. Housing and Welfare (Article 13F2) 1. Housing Standards Animals must be kept in environments that meet their physical and psychological needs: Enrichment: Examples: Mice are given nesting materials to exhibit natural burrowing behaviors. Monkeys may have climbing structures to mimic their natural habitat. Social Housing: Social species like rodents should live in groups, unless isolation is required for health or experimental reasons. Risks of isolation: Increased stress levels, depression-like behavior. Climate Control: Temperature and humidity must match species-specific needs. For example, tropical species like frogs require higher humidity, while mice need dry conditions. Cage Size: Minimum space requirements are set to prevent overcrowding. Example: A single rat requires a cage at least 800 cm² with a height of 18 cm. Level of Discomfort (Article 10B) 1. Anesthesia and Analgesics Pain management is essential unless justified: Anesthesia: Ensures the animal is unconscious during invasive procedures. Example: Using isoflurane gas during surgery. Analgesics: Painkillers like buprenorphine are administered post-surgery. 2. Avoiding Suffering Ethical guidelines mandate replacing death or severe suffering with alternative metrics: Death as a Parameter: Example: In toxicity studies, death should not be the endpoint. Instead, researchers monitor biomarkers (e.g., liver enzyme levels) to determine toxicity. Humane Endpoints: Animals are removed from experiments before severe suffering, as detailed below. Humane Endpoints (Article 13B) 1. Criteria for Stopping an Experiment Experiments must stop if: Results are Achieved: Example: If drug efficacy is confirmed after 10 days, extending the experiment serves no purpose. Unintentional Suffering: Example: If an animal develops an unexpected illness during the study, the experiment must end. Model Unsuitability: Example: If a mouse develops tumors that compromise its mobility, it no longer serves as a valid cancer model. 2. Misunderstandings about Humane Endpoints Killing the animal is not always required at a humane endpoint. For example: Case 1: An animal in mild distress can be treated and monitored. Case 2: If conditions worsen (e.g., severe tumor growth), euthanasia may be the only humane option. 3. Indicators of Suffering Monitoring parameters include: Behavioral Changes: Examples: Mice may stop grooming, leading to unkempt fur. Lethargy or excessive hiding. Physical Signs: Examples: Tumor growth exceeding 1 cm³. Open wounds that fail to heal. Food and Water Intake: A decline often indicates illness or distress. Ethical Considerations Russell and Burch’s quote highlights the 3Rs Principle, emphasizing that humane treatment is integral to reliable scientific outcomes. By reducing stress and pain, researchers ensure the validity of their data (e.g., stress hormones can skew results). Slide 12: Humane Endpoints (Type 2) Introduction to Humane Endpoints Humane endpoints refer to predetermined criteria or conditions under which an animal in an experiment is euthanized to avoid or minimize unnecessary suffering. These are essential for ensuring ethical practices in animal research and balancing the welfare of the animals with the scientific goals of the study. Key Considerations For the Animal: Preventing unnecessary discomfort: Experiments can involve procedures that may cause pain, stress, or other adverse effects. Humane endpoints ensure that animals do not endure suffering beyond what is absolutely necessary for the scientific goals. Indicators of suffering: Researchers must establish and recognize clear signs of distress or deteriorating health. For instance, signs like weight loss, inability to eat, severe lethargy, or abnormal behavior might trigger the humane endpoint. For the Researcher: Unreliable results: An animal in poor health may exhibit biological responses unrelated to the experimental variables, increasing variability and undermining the reliability of the results. Loss of data: If an animal dies spontaneously without controlled euthanasia, critical data might be lost, reducing the overall scientific yield of the experiment and possibly requiring additional animals to be used. Practical Implementation Researchers must develop a welfare monitoring plan that includes humane endpoints before starting the experiment. This plan should be reviewed and approved by an Animal Welfare Body (AWB) or an equivalent ethical committee. Continuous monitoring ensures that any signs of distress are promptly addressed. Connection to Broader Ethical Principles This aligns with the 3Rs principle in animal research: Replacement: Avoiding or replacing animal use where possible. Reduction: Minimizing the number of animals used. Refinement: Enhancing experimental techniques to reduce suffering. Slide 13: Methods of Killing (Art. 13a-c) Directive 2010/63/EU Overview This directive governs the use of animals in scientific experiments across the European Union. Annex IV specifies permissible methods of euthanasia to ensure humane treatment. Methods of Killing The approved methods reflect a balance between scientific needs and animal welfare: Cessation of Circulation: Methods like intravenous injection of an overdose of anesthetics are used to stop the heart. This method is quick and minimizes pain. Verification is crucial: Tools like ECG (electrocardiography) might confirm the heart has stopped. Destruction of the Brain: This involves physical or chemical means to ensure the animal is no longer capable of perception or experiencing pain. Common methods include captive bolt devices or chemical agents. Neck Dislocation: A manual or mechanical method that instantly severs the spinal cord, causing rapid unconsciousness and death. It is typically reserved for small animals like rodents and must be performed by trained personnel to ensure effectiveness. Exsanguination: The removal of blood, typically performed after the animal has been rendered unconscious. Often used in conjunction with other methods to ensure the animal is deceased. Rigor Mortis Confirmation: The stiffening of the body post-mortem confirms death. This is a final step for assurance, particularly in situations where other methods leave ambiguity. Legal and Ethical Safeguards Sedation is mandated if alternative methods are used, ensuring the animal does not experience pain. The AWB oversees adherence to these methods and ensures proper training for personnel. Slide 14: Euthanasia (Art. 13c) Integration of Ethical, Legal, and Scientific Demands Legal Demands: Researchers must strictly follow approved euthanasia methods and document the procedures. Non-compliance can lead to legal actions, including withdrawal of licenses. Animal Welfare: The process must prioritize minimizing pain, fear, and distress for the animal. A humane approach to euthanasia respects the dignity of the animal. Scientific Quality: Proper euthanasia ensures that biological samples remain uncontaminated and reliable. Unethical euthanasia practices can compromise scientific integrity and public trust. Slide 15-16: From Idea to Animal Experiment (Art. 2-8) Personnel and Qualifications Animal Caretaker (MBO Level 3): Responsible for routine care, including feeding, cleaning, and observing animals for signs of distress. Acts as the first line of defense in identifying welfare issues. Animal Technician (MBO Level 4, HBO): Trained to perform technical procedures, such as administering medications or collecting biological samples. Plays a role in experimental setups and welfare monitoring. Veterinarian: Ensures the overall health of the animals. Provides expertis