Additives in Plastics PDF
Document Details
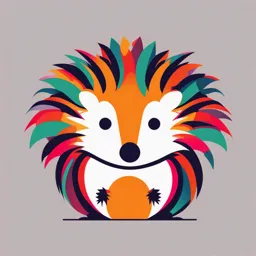
Uploaded by AdaptableOnyx3137
Tags
Summary
This document discusses various additives used in plastics, focusing on their roles in enhancing properties and applications, particularly in food packaging. It examines processing aids, plasticizers, antiaging agents, and other functionalities. Further, it details the advantages and disadvantages of different packaging materials, including plastics, glass, and metals.
Full Transcript
ADDITIVES IN PLASTICS Early in the development of the plastics industry it was realized that to obtain better products, additives needed to be added to the base polymer. Within the context of thermoplastics materials technology, the term additive is used to denote an auxiliary ingredient th...
ADDITIVES IN PLASTICS Early in the development of the plastics industry it was realized that to obtain better products, additives needed to be added to the base polymer. Within the context of thermoplastics materials technology, the term additive is used to denote an auxiliary ingredient that enhances the properties of the parent polymer without appreciably altering its chemical structure. In food packaging, all additives should have received clearance by the appropriate food regulatory authority. Processing Additives The degradation of polymers frequently involves oxidation reactions by a free radical mechanism, and at high temperatures, interaction of O2, with C-H bonds leads to the formation of hydroperoxide groups. These decompose into very reactive OH radicals and lead to molecular scissions. Because it is practically impossible to eliminate O2 from the system, additives are used to inhibit oxidation reactions. This is accomplished by using primary stabilizers or antioxidants such as hindered phenols or aromatic amines, which interrupt the chain reaction by combining with the free radicals; secondary stabilizers or peroxide decomposers such as organic thioesters, phosphites and metal thiocarbamates, which react with hydroperoxides as they are formed; and chelating agents or metal deactivators such as organic phosphites and hydrazides, which protect the polymer by immobilizing metal ions through coordination reactions. Processing Additives With PVC, heat stabilizers or acid absorbers must be used to retard the decomposition of PVC into HCl and dark, degraded polymers The tendency for polymers such as PVC and polyolefins to stick to metal parts during processing can be reduced by adding lubricants such as PE waxes, fatty acid esters and amides, metallic stearates such as zinc and calcium stearate, and paraffin. Plasticizers Brittle polymers such as PVC must be plasticized to obtain flexible films and containers. The plasticizer also gives the material the limp and tacky qualities found in "cling" films. About 80% of all plasticizers are used in PVC. Typically, phthalic esters are used, as well as epoxidized oils and low- MW polyesters. Internal plasticization may be brought about by copolymerization as in the case of PVC, which can be copolymerized with vinyl acetate, ethylene or methylacrylate. Antiaging Additives Aging is the process of deterioration of materials resulting from the combined effects of atmospheric radiation, temperature, O2, water, microorganisms and other atmospheric agents (e.g., gases), indicating that a chemical modification in the structure of the material has occurred. Antioxidants have already been mentioned under processing aids, but they are also necessary in polymeric films such as PP, which degrade in the atmosphere. BHT has been cleared by the FDA and acts as a free radical scavenger. Organophosphites act as hydroperoxide decomposers. It is common for different antioxidants to be used together for synergistic effects. UV stabilizers are used to prevent the deterioration of polymeric films by photooxidation. They act by absorbing high-energy UV radiation and releasing it as lower-energy radiation. Antifogging films In some food packaging applications, moisture tends to condense as small droplets on the internal surface. Antifogging films also let consumers see food in packages clearly. Antifogging polymeric films prevent the formation of fog inside the food packages, for example fresh produce and raw meat packages. The antifogging property is essential for the packaging film when it is used for packaging of frozen products. Untreated LDPE and pullulan-coated antifogging film after removal from the refrigerator (7 days at 4 °C) at 20 °C. Antifogging agents Antifogging materials are typically surface-active agents which consist of two parts: a hydrophilic head and a lipophilic tail; examples include glycerol fatty acid ester, polyglycerol fatty acid ester, a fatty acid ester of polyethylene glycol, alkyl ether of polyethylene glycol, ethoxylated alkyl phenol, sorbitan ester, ethoxylated sorbitan ester, and alkanol. When the antifogging agent incorporated in a polymer matrix, the antifogging agent migrates from the matrix to the film surface, reducing the interfacial tension between the polymer and the water drops. As a result, the water drops spread across the film surface and form a continuous thin layer. These so-called antifogging agents can be applied on the surface of the material or compounded internally in the packaging material at levels ranging from 0.5% to 4%. Antistatic agents Static electricity is generated on a polymer surface by friction or by rubbing it against another surface. It can also be generated on fast-moving film during converting operations or on filling lines. Antistatic agents are used to prevent the accumulation of electrical charges in polymeric films, an undesirable effect caused by the fact that polymers are nonconductors of electricity. Electrification of films results from a segregation of charges (electrons and ions), which occurs when two surfaces are parted after close initial contact. The addition of ethoxylated fatty amines, polyhydric alcohols and derivatives, and nonionic and quaternary ammonium compounds overcomes the problem; they migrate to the surface and form a conducting layer through the absorption of atmospheric moisture, which permits the discharge of electrons. Antistatic agents Antiblocking agents Many packaging films or sheets tend to stick together because they are nonconductors of electricity, a phenomenon known as blocking. Anti-block agent reduces the friction between packages so that they can be easily separated from each other. It also ensures that exactly one cup or tray is picked up at automated filling lines. Blocking (which may develop under pressure during storage or use) can be reduced by colloidal silica, clays, starches and silicones that may be applied to the surface either during or after processing to reduce blocking. Anti-blocking agents are used at levels ranging from 0.1% to 0.5%. Recommendations for Antiblock Additives Antiblocking agents Chemically inert, inorganic antiblock additives are most commonly used. Inorganic antiblock additives migrate to the film surface, partially stick out and create a microroughness of the film surface A barrier layer is formed on the plastic film surface, thus inhibiting the two adjacent plastic film layers’ adhesion. Slip agents Slip is when polymeric films slide parallel over each other. Slip is a surface effect. Slip is quantified via the coefficient of friction (COF). If films have a high COF, individual film layers have a high surface friction and tend to stick together instead of sliding over one another. Most commonly, migrating slip agents are fatty acid amides. Optical Property Modifiers The optical properties of a material from a technological aspect are normally described in terms of their ability to transmit light, exhibit color and reflect light from the surface (i.e., gloss). The majority of food packaging films are not pigmented, although some are colored by the addition of colorants that can be dyes (which are soluble in the plastic and tend to migrate, thus limiting their use) and pigments (which are insoluble in the plastic matrix). The principal pigments for use as colorants in packaging are carbon black, white titanium dioxide, red iron oxide, yellow cadmium sulfide, molybdate orange ultramarine blue blue ferric ammonium ferrocyanide, chrome green (Cr2O3). The FDA has questioned the use of some of these colorants in packaging material in contact with food, its concern being with colorants that can migrate from the packaging into the food. Foaming Agents Foaming or blowing agents are used for the production of cellular products and are normally classified into physical and chemical types, according to whether the generation of gases to produce the cells Micrographs showing the cellular takes place through a physical blowing agent (i.e., structure of polypropylene foams inert gases) or by a chemical process (i.e., thermal produced using CO2 as blowing agent decomposition reactions that result in evolution of gases). Today, expanded and extruded PS foams are made using mainly CO2 or sometimes a light aliphatic hydrocarbon such as pentane or butane as the blowing agent. Expanded PET, PP and PVC foams are produced using chemical blowing agents such as CO2 and N2. Cellular structure of PP foams produced using different amounts of azodicarbonamide Plastic Food Packaging Material Advantages Low-cost (package manufacturing and storage) Often practical for in-line package formation Moldable Heat sealable (most) Light weight Transparent (most) Microwaveable (some) Dual-ovenable (some) Non-corrodible Physical and thermal shock-resistant Stronger and better flexible barrier than paper Recyclable (some) Plastic Food Packaging Material Disadvantages Permeable to moisture, O2, CO2, ethanol & aromas Lower compressive strength than glass & metal Less heat resistant than glass & metal Potential for interaction with food Some plastics not recyclable Multi-layer plastic packages not recyclable Packaging Materials Plastics Polyolefins Copolymers of ethylene Substituted olefins Polyesters Polyamides Glass Metal Paper Packaging Materials: Glass It is an inert packaging material with absolute barrier to gases and moisture making it versatile packaging material to retain the flavor and freshness of delicate food products like beer and wine. The other advantages of using glass are that it can withstand high ADVANTAGE thermal processing conditions, provides good insulation, can be formed into different shapes, and can be supplied in different colors with an optical transmission between nearly opaque and nearly transparent. Additional oxide coatings help to improve the mechanical properties and give a barrier against chemical attack. Heavy weight, and fragility to internal pressure, impact and thermal shock are some of the limitations for their extensive DISADVANTAGE use in food industry. Packaging Materials: Glass An amorphous, inorganic product of fusion that has been cooled to a rigid condition without crystallizing. Although rigid, glass is a highly viscous liquid that exists in a vitreous or glassy state. A typical formula for soda-lime glass o silica, SiO2 68–73% o calcia, CaO 10–13% o soda, Na2O 12–15% o alumina, Al2O3 1.5–2% o iron oxides, FeO 0.05–0.25% Packaging Materials: Glass ✓ Excellent barrier to water vapor, gases, and odors. × Incorrectly designed or applied closure may negate the benefits that glass packaging offers in protecting foods from deterioration. - The two main types of glass containers used in food packaging are; Bottles (which have narrow necks) Jars (which have wide mouths or openings) - Approximately 75% of all glass food containers are bottles. - Approximately 80% of glass container is clear, the remainder being mainly amber or green. Today’s glass containers are lighter but stronger than their predecessors, that is how glass container has remained competitive and continues to play a significant although declining role in the packaging of foods. Glass Container Preferences Glass Packaging Institute Glass Container Preferences Glass Packaging Institute Glass Packaging Production Glass Packaging Institute Glass Container Trends Lightweighting – 1966 beer bottle: 11 oz – 2000 beer bottle: 6.5 oz Development of Narrow Neck Press & Blow Process – Better distribution of glass throughout the bottle – Glass thickness varies with need for strength Improvement of Surface Treatments (reduced scratching) Innovative Container Shapes, Labels & Decorating Packaging Materials: Metals - Four metals that are commonly used for the packaging of foods: Aluminum (Al) Chromium (Cr) Steel Tin (Sn) - Tin and steel, and chromium and steel, are used as composite materials in the form of tinplate and electrolytically chromium-coated steel (ECCS) or tin-free steel (TFS). - Aluminum is used in the form of purified alloys containing small and carefully controlled amounts of magnesium and manganese. Packaging Materials: Metals The basic metals used for food packaging are steel and aluminum. Steel has to be plated against corrosion by tin (thus giving tinplate) or chromium. Tin resists corrosion from water, but can be corroded by acids and alkalis They offer excellent barrier properties, physical protection, printability, consumer acceptance, and recyclability. Tinplate is produced from low-carbon steel, coated on both sides with a thin layer of tin. In most applications, they are further coated with epoxy or polyester resins to provide an additional barrier to the food materials against corrosion. Containers from tinplate or aluminum are commonly used in retort processing of fruit and vegetables, meat, fish, pulses, cans for drinks, containers for baby foods or powders, confectionery, etc. Aluminum is further used to produce flexible packaging materials like foil, laminated paper/plastic films, and metalized films. It has several advantages over other metals such as light weight, corrosion resistance and good recycling properties. Packaging Materials: Metals Aluminum, the earth’s most abundant metallic constituent, is used to manufacture both metal cans and thin foil in thicknesses ranging from 4 to 150 µm; foils thinner than 20 µm contain minute pinholes that are permeable to gases and water vapor. In both applications, alloying agents including silicon, iron, copper, manganese, magnesium, chromium, zinc, and titanium are added to impart strength, and improve formability and corrosion resistance. Metal Containers Steel Cans – Food Cans (most) – Beverage Cans (few) – Aerosol Cans (minor use) Aluminum Cans – Food Cans (few) – Beverage Cans (most) – Aerosol Cans (minor use) Aluminum Trays and Pans Aluminum Foils Aluminum “Bottles” Metal Container Advantages Metal impervious to H2O, O2, CO2, aromas, etc. Lighter weight than glass – Glass bottle ~220g, steel can ~ 35g, aluminum can ~15g. Can double-seam closure hermetically sealed Impervious to light High heat resistance High resistance to physical and thermal shock Faster heat processing than glass container Tamper evident Recyclable Metal Container Disadvantages Multi-step manufacturing One shape (shaped cans expensive & difficult to run) Cylindrical shape occupies excess space in storage Do not allow viewing of product Non-hygienic to drink from can Not microwaveable Perceived as “old fashioned” &/or do not add value Not refillable or reclosable/resealable (generally) Metal Container Utilization Al Soft Drink Al Beer St Human Food St Pet Food Can Manufacturers Institute Paper Packaging Packaging Materials: Paper Paper is another commonly used material in the packaging of several food products. Plain paper is hardly ever used for food packaging alone. It has to be modified with several additives (lacquers, waxes, resins, etc.) or extrusion coated with other polymers to improve the barrier properties. Paper and paper boards are used in several forms such as corrugated boxes, cartons, bags, sacks, and wrapping paper. Packaging Materials: Paper Selected forms of paper used for packaging: 1. Kraft paper - Available as natural brown, unbleached, and bleached white. It is the strongest type of paper and is used for bags and wrappings. 2. Sulfite paper - It is usually glazed to improve the appearance, wet strength, and oil resistance. Sulfite paper is lighter and weaker than kraft paper but has high print quality. It is often used in plastic or foil laminates. 3. Densified and greaseproof papers of different types For example, glassine or parchment is used to package biscuits, confectionery, and fats. They are resistant to fat but have high moisture permeability. 4. Paperboard - Available in several forms (whiteboard, solidboard, chipboard, fiberboard, and paper laminates), mainly used as secondary packages to improve the handling and distribution of food products. Packaging Materials: Paper ❑Almost all paper is converted after manufacture by undergoing further treatment such as embossing, coating, laminating, and forming into special shapes and sizes such as bags and boxes. ❑Further surface treatment involving the application of adhesives and printing inks are common, depending on the end use. ❑Disadvantage: Paper and paperboard are poor barriers to gases and water vapor; uncoated paper packaging provides little more than protection from light and minor mechanical damage. Packaging Materials: Paper In many packaging applications, a barrier is needed against water vapor and/or gases such as O2. A water barrier can be formed by changing the wettability of the paper surface with sizing agents, whereas coating the paper with plastic polymers provides a good barrier to gases and water vapor. Beverage cartons consist of layers of paperboard coated internally and externally with low density polyethylene (LDPE), resulting in a carton that is impermeable to liquids and in which the internal and external surfaces may be heat sealed. There may also be a thin layer (6.5 µm) of aluminum foil, which acts as a gas and light barrier. Paper/board Advantages ❑ Provide barrier to light ❑ Paper can be made into a variety of single-wall bags (e.g., grocery bags) and multi-wall bags (e.g., flour, sugar) ❑ Paperboard provides strength and mechanical protection in many packages ❑ Paper and paperboard can be coated or laminated with wax, ❑ LDPE, PP or PET to improve H2O and O2 barrier properties and/or allow heat-sealability (LDPE) or heat-resistance (PP or PET) ❑ Uncoated/unlaminated paper and paperboard can be recycled Paper/board Disadvantages Negligible moisture, gas, or microorganism barrier properties when not coated or laminated Not heat sealable when not coated or laminated Coated or laminated paper and paperboard are, in general, not recyclable Paper Plastic Glass Metal Paper and cardboard / Plastic / Aluminium Plastic Metal Paper Plastic Foiled paper lid Plastic Glass lid lid Plastics / aluminum Glass Paper Polypropylene Low Density Poly Ethylene Plastics / aluminum- Paper and cardboard / Polyethylene Smooth Cardboard Polypropylene plastics/aluminum- terephthalate Paper Polyethylene Paper and cardboard / White Glass Terephthalate plastics/aluminum- Paper Packaging Material Properties Mechanical Properties Optical Properties of Glass and Plastic Packaging Barrier Properties of Plastic Packaging Factors Affecting Permeability Packaging Materials Plastics Polyolefins Copolymers of ethylene Substituted olefins Polyesters Polyamides Glass Metal Paper Packaging Material Properties Mechanical Properties Optical Properties of Glass and Plastic Packaging Barrier Properties of Plastic Packaging Factors Affecting Permeability Tensile Strength The tensile strength of a material quantifies how much stress the material will endure before failing. In general tensile strength increases with polymer chain length. Tensile stress is the force exerted per unit cross-sectional area of the object whereas the tensile strain is the extension per unit original length of the object. Tensile Strength Mechanical behavior of amorphous and semi-crystalline polymers is strongly affected by Tg Polymers whose Tg is above the service temperature are strong, stiff and sometimes brittle (Tg about 100oC) e.g. Polystyrene (cheap, clear,hard and brittle plastic drink cups) Polymers whose Tg (-100oC) is below the service temperature are weaker, less rigid, and more ductile (flexible) Polyethylene (milk jugs) ASTM D882 is a common testing standard that is used to determine the tensile properties of thin plastic films and is commonly used for in-line quality control purposes. American Society for Testing and Materials Optical Properties of Glass and Plastic Packaging Glass, because it has no crystalline structure, is optically isotropic when it is homogeneous and free from any stresses. The optical properties of glass relate to the degree of penetration of light and the subsequent effect of that transmission, transmission being a function of wavelength. Light transmission may be controlled by the addition of coloring additives such as metallic oxides, sulfides, or selenides. Most of the transition metal oxides (e.g., cobalt, nickel, chromium, iron, etc.) give rise to absorption bands, not only in the visible but also in the UV and IR regions of the spectrum. The three main colors of glass used to produce food containers are flint or clear, amber or brown, and green. Typical light transmission of the glasses Amber glass provides a reasonable degree of light protection quite economically. A light-resistant container is defined as one that passes no more than 10% of incident radiation at any wavelength between 290 nm and 450 nm through the average sidewall thickness. Optical Properties of Glass and Plastic Packaging Optical properties of plastics are related to both the degree of crystallinity and the actual polymer structure. Optical properties of importance with thermoplastic polymers include clarity, haze, color, transmittance, reflectance, gloss, and refractive index. The clarity of a film indicates the degree of distortion of an object when viewed through the film, with ‘see-through’ clarity referring to the ability of the film to resolve fine details of fairly distant images viewed through the film. Barrier Properties of Plastic Packaging Unlike metal and glass packages, plastic packages are permeable and the concept of permeability is normally associated with the quantitative evaluation of the barrier properties of a plastic. A plastic that is a good barrier has a low permeability. The protection of foods from gas and vapor exchange with the environment depends on the integrity of packages (including their seals and closures), and on the permeability of the packaging materials themselves. The barrier properties of plastics indicate their resistance to sorption and diffusion of substances such as gases and flavor and aroma compounds. Barrier Properties of Plastic Packaging Under steady-state conditions, a gas or vapor diffuses through a polymer at a constant rate if a constant pressure difference is maintained across the polymer. The diffusive flux, J, of a permeant (vapor) in a polymer can be defined as the amount passing through a plane (surface) of unit area normal to the direction of flow during unit time as follows: 𝐽 = 𝑄/𝐴𝑡 where Q is the total amount of permeant that has passed through area A during time t. Barrier Properties of Plastic Packaging The relationship between the rate of permeation and the concentration gradient is one of direct proportionality and is embodied in Fick’s first law: 𝛿𝑐 𝐽 = −𝐷 𝛿𝑥 Equation can be used to calculate the steady-state rate of diffusion assuming that D is constant and the concentration is a function only of the geometric position inside the polymer. Barrier Properties of Plastic Packaging Consider a polymer X mm thick, of area A, exposed to a permeant at pressure p1 on one side and at a lower pressure p2 on the other as shown in Figure. The concentration of permeant in the first layer of the polymer is c1 and in the last layer c2. When steady-state diffusion has been reached, J=constant and ODE can be integrated across the total thickness of the polymer X, and between the two concentrations, assuming D to be constant and independent of c: 𝐽𝑋 = −𝐷(c2 − c1) 𝐷 c2 − c1 𝐴𝑡 𝑄= 𝑋 Barrier Properties of Plastic Packaging By substituting for J, the quantity of permeant Q diffusing through a polymer of area A in time t can be calculated: 𝐷 c2 − c1 𝐴𝑡 𝑄= 𝑋 Rather than the actual concentration, it is more convenient when the permeant is a gas to measure the vapor pressure p, which is at equilibrium with the polymer, rather than measure the actual concentration. Henry’s law applies at low concentrations and c can be expressed as: 𝑐 = 𝑆𝑝 where S is the solubility coefficient of the permeant in the polymer (it reflects the amount of permeant in the polymer). Barrier Properties of Plastic Packaging 𝐷 c2 − c1 𝐴𝑡 𝑄= 𝑐 = 𝑆𝑝 𝑋 𝐷𝑆 𝑝1 − p2 𝐴𝑡 𝑄= 𝑋 The product DS is referred to as the permeability coefficient or constant and is represented by the symbol P: 𝑃 = 𝐷𝑆 Barrier Properties of Plastic Packaging Thus, the permeability coefficient is the product of a kinetic term (D), which reflects the dynamics of the penetrant-polymer system, and of a thermodynamic term (S), which depends on the penetrant-polymer interactions. P represents the ease with which a gas permeates through a polymer when subjected to a pressure gradient. 𝑄𝑋 𝑃= 𝐴𝑡 𝑝1 − p2 or 𝑄 𝑃 = 𝐴(Δ𝑝) 𝑡 𝑋 Barrier Properties of Plastic Packaging 𝑄 𝑃 = 𝐴(Δ𝑝) 𝑡 𝑋 ❑The term P/X is called the permeance; it is not a property of the material but a performance evaluation indicator. ❑Although steady-state is usually attained in a few hours for small molecules such as O 2 , larger molecules in barrier polymers (especially glassy polymers) can take a long time to reach steady-state, with this time possibly exceeding the anticipated shelf life. ❑Although D and S are independent of concentration for many gases such as O2, N2, and, to a certain extent, CO2, this is not the case where considerable interaction between polymer and permeant takes place (e.g., water and hydrophilic films such as EVOH and PAs, or many solvent vapors diffusing through polymer films). Barrier Properties of Plastic Packaging ❑Although the chemical structure of a polymer can be considered to be the predominant factor that controls the magnitude of P, it also varies with the morphology of the polymer and depends on many physical factors such as density, crystallinity, and orientation. ❑The dimensions of P are: 𝑞𝑢𝑎𝑛𝑡𝑖𝑡𝑦 𝑜𝑓 𝑝𝑒𝑟𝑚𝑒𝑎𝑛𝑡 𝑢𝑛𝑑𝑒𝑟 𝑠𝑡𝑎𝑡𝑒𝑑 𝑐𝑜𝑛𝑑𝑖𝑡𝑖𝑜𝑛𝑠 (𝑡ℎ𝑖𝑐𝑘𝑛𝑒𝑠𝑠) 𝑃= 𝑎𝑟𝑒𝑎 𝑡𝑖𝑚𝑒 (𝑝𝑟𝑒𝑠𝑠𝑢𝑟𝑒 𝑑𝑟𝑜𝑝 𝑎𝑐𝑟𝑜𝑠𝑠 𝑝𝑜𝑙𝑦𝑚𝑒𝑟) Packaging Material Properties Mechanical Properties Optical Properties of Glass and Plastic Packaging Barrier Properties of Plastic Packaging Factors Affecting Permeability Tensile Strength The tensile strength of a material quantifies how much stress the material will endure before failing. In general tensile strength increases with polymer chain length. Tensile Strength Mechanical behavior of amorphous and semi-crystalline polymers is strongly affected by Tg Polymers whose Tg is above the service temperature are strong, stiff and sometimes brittle e.g. Polystyrene (cheap, clear plastic drink cups) Polymers whose Tg is below the service temperature are weaker, less rigid, and more ductile Polyethylene (milk jugs) Optical Properties of Glass and Plastic Packaging Glass, because it has no crystalline structure, is optically isotropic when it is homogeneous and free from any stresses. The optical properties of glass relate to the degree of penetration of light and the subsequent effect of that transmission, transmission being a function of wavelength. Light transmission may be controlled by the addition of coloring additives such as metallic oxides, sulfides, or selenides. Most of the transition metal oxides (e.g., cobalt, nickel, chromium, iron, etc.) give rise to absorption bands, not only in the visible but also in the UV and IR regions of the spectrum. The three main colors of glass used to produce food containers are flint or clear, amber or brown, and green. Typical light transmission of the glasses Amber glass provides this degree of light protection quite economically. A light-resistant container is defined as one that passes no more than 10% of incident radiation at any wavelength between 290 nm and 450 nm through the average sidewall thickness. Optical Properties of Glass and Plastic Packaging Optical properties of plastics are related to both the degree of crystallinity and the actual polymer structure. Optical properties of importance with thermoplastic polymers include clarity, haze, color, transmittance, reflectance, gloss, and refractive index. Barrier Properties of Plastic Packaging Unlike metal and glass packages, plastic packages are permeable and the concept of permeability is normally associated with the quantitative evaluation of the barrier properties of a plastic. A plastic that is a good barrier has a low permeability. The protection of foods from gas and vapor exchange with the environment depends on the integrity of packages (including their seals and closures), and on the permeability of the packaging materials themselves. The barrier properties of plastics indicate their resistance to sorption and diffusion of substances such as gases and flavor and aroma compounds. Barrier Properties of Plastic Packaging Under steady-state conditions, a gas or vapor diffuses through a polymer at a constant rate if a constant pressure difference is maintained across the polymer. The diffusive flux, J, of a permeant (vapor) in a polymer can be defined as the amount passing through a plane (surface) of unit area normal to the direction of flow during unit time as follows: 𝐽 = 𝑄/𝐴𝑡 where Q is the total amount of permeant that has passed through area A during time t. Barrier Properties of Plastic Packaging The relationship between the rate of permeation and the concentration gradient is one of direct proportionality and is embodied in Fick’s first law: 𝛿𝑐 𝐽 = −𝐷 𝛿𝑥 Equation can be used to calculate the steady-state rate of diffusion assuming that D is constant and the concentration is a function only of the geometric position inside the polymer. Barrier Properties of Plastic Packaging Consider a polymer X mm thick, of area A, exposed to a permeant at pressure p1 on one side and at a lower pressure p2 on the other as shown in Figure. The concentration of permeant in the first layer of the polymer is c1 and in the last layer c2. When steady-state diffusion has been reached, J=constant and ODE can be integrated across the total thickness of the polymer X, and between the two concentrations, assuming D to be constant and independent of c: 𝐽𝑋 = −𝐷(c2 − c1) 𝐷 c2 − c1 𝐴𝑡 𝑄= 𝑋 Barrier Properties of Plastic Packaging By substituting for J, the quantity of permeant Q diffusing through a polymer of area A in time t can be calculated: 𝐷 c2 − c1 𝐴𝑡 𝑄= 𝑋 Rather than the actual concentration, it is more convenient when the permeant is a gas to measure the vapor pressure p, which is at equilibrium with the polymer, rather than measure the actual concentration. Henry’s law applies at low concentrations and c can be expressed as: 𝑐 = 𝑆𝑝 where S is the solubility coefficient of the permeant in the polymer (it reflects the amount of permeant in the polymer). Barrier Properties of Plastic Packaging 𝐷 c2 − c1 𝐴𝑡 𝑄= 𝑐 = 𝑆𝑝 𝑋 𝐷𝑆 𝑝1 − p2 𝐴𝑡 𝑄= 𝑋 The product DS is referred to as the permeability coefficient or constant and is represented by the symbol P: 𝑃 = 𝐷𝑆 Barrier Properties of Plastic Packaging Thus, the permeability coefficient is the product of a kinetic term (D), which reflects the dynamics of the penetrant-polymer system, and of a thermodynamic term (S), which depends on the penetrant-polymer interactions. P represents the ease with which a gas permeates through a polymer when subjected to a pressure gradient. 𝑄𝑋 𝑃= 𝐴𝑡 𝑝1 − p2 or 𝑄 𝑃 = 𝐴(Δ𝑝) 𝑡 𝑋 Barrier Properties of Plastic Packaging 𝑄 𝑃 = 𝐴(Δ𝑝) 𝑡 𝑋 ❑The term P/X is called the permeance; it is not a property of the material but a performance evaluation indicator. ❑Although steady-state is usually attained in a few hours for small molecules such as O2, larger molecules in barrier polymers (especially glassy polymers) can take a long time to reach steady-state, with this time possibly exceeding the anticipated shelf life. ❑Although D and S are independent of concentration for many gases such as O2, N2, and, to a certain extent, CO2, this is not the case where considerable interaction between polymer and permeant takes place (e.g., water and hydrophilic films such as EVOH and PAs, or many solvent vapors diffusing through polymer films). Barrier Properties of Plastic Packaging ❑Although the chemical structure of a polymer can be considered to be the predominant factor that controls the magnitude of P, it also varies with the morphology of the polymer and depends on many physical factors such as density, crystallinity, and orientation. ❑The unit of P is: 𝑞𝑢𝑎𝑛𝑡𝑖𝑡𝑦 𝑜𝑓 𝑝𝑒𝑟𝑚𝑒𝑎𝑛𝑡 𝑢𝑛𝑑𝑒𝑟 𝑠𝑡𝑎𝑡𝑒𝑑 𝑐𝑜𝑛𝑑𝑖𝑡𝑖𝑜𝑛𝑠 (𝑡ℎ𝑖𝑐𝑘𝑛𝑒𝑠𝑠) 𝑃= 𝑎𝑟𝑒𝑎 𝑡𝑖𝑚𝑒 (𝑝𝑟𝑒𝑠𝑠𝑢𝑟𝑒 𝑑𝑟𝑜𝑝 𝑎𝑐𝑟𝑜𝑠𝑠 𝑝𝑜𝑙𝑦𝑚𝑒𝑟) Barrier Properties of Plastic Packaging ❑The treatment of steady-state diffusion assumed that both D and S are independent of concentration but in practice deviations do occur. ❑It does not hold for heterogeneous materials such as coated or laminated films, or when there is interaction between polymer and permeant. The property is then defined as the transmission rate (TR) of the material, where: 𝑄 𝑇𝑅 = 𝐴𝑡 where Q is the amount of permeant passing through the polymer, A is the area, and t is the time. ❑Permeabilities of polymers to water and organic compounds are often presented in this way, and in the case of water and O 2 , the terms WVTR and oxygen transmission rate (OTR) are in common usage. Barrier Properties of Plastic Packaging It is critical that the thickness of the film or laminate, the temperature and the partial pressure difference of the gas or water vapor are specified for a particular TR. Typically, units of (g m-2 day-1) are used for WVTR and (ml m-2 day -1) for OTR. Often the WVTRs are given for so called tropical conditions of 38 °C/90% RH but sometimes for so-called temperate conditions of 25 °C/75% RH; OTRs are often given for 23 °C/0% RH. TR is related to P as follows: 𝑄𝑋 𝑋 𝑃= = 𝑇𝑅 𝐴𝑡Δ𝑝 Δ𝑝 25 µm (2.5 x 10-3 cm) thickness Permeability Units 𝑄𝑢𝑎𝑛𝑡𝑖𝑡𝑦 𝑜𝑓 𝑃𝑒𝑟𝑚𝑒𝑎𝑛𝑡 𝑈𝑛𝑑𝑒𝑟 𝑆𝑡𝑎𝑡𝑒𝑑 𝐶𝑜𝑛𝑑𝑖𝑡𝑖𝑜𝑛𝑠 (𝑇ℎ𝑖𝑐𝑘𝑛𝑒𝑠𝑠) 𝑃 = 𝑃𝑒𝑟𝑚𝑒𝑎𝑏𝑖𝑙𝑖𝑡𝑦 = 𝑎𝑟𝑒𝑎 𝑡𝑖𝑚𝑒 (𝑝𝑟𝑒𝑠𝑠𝑢𝑟𝑒 𝑑𝑟𝑜𝑝 𝑎𝑐𝑟𝑜𝑜𝑠 𝑡ℎ𝑒 𝑝𝑜𝑙𝑦𝑚𝑒𝑟) ∆𝑄 𝑥 𝑥 𝑃= = 𝑇𝑅 ∗ > 𝑇𝑅 = 𝑇𝑟𝑎𝑛𝑠𝑓𝑒𝑟 𝑅𝑎𝑡𝑒 ∶ 𝑂𝑇𝑅 𝑓𝑜𝑟 𝑜𝑥𝑦𝑔𝑒𝑛 ∆𝑡. 𝐴 𝑝1 − 𝑝2 𝑝1 − 𝑝2 WVTR : Water Vapor Transfer Rate 10−10 (𝑚𝑙 𝑆𝑇𝑃൯ 𝑐𝑚 Barrer = 𝑐𝑚2 𝑠 (𝑐𝑚 𝐻𝑔) EXAMPLE: Calculate the permeability coefficient P of an OPP film to O2 at 23 oC given that the Oxygen Transmission Rate (OTR) through a 2.5*10-2 mm thick film with air on one side and inert gas on the other side is 1550 mL m-2 day-1 EXAMPLE Calculate the permeability coefficient P of an OPP film to O2 at 23 oC given that the Oxygen Transmission Rate (OTR) through a 2.5*10-2 mm thick film with air on one side and inert gas on the other side is 1550 mL m-2 day-1 Air contains approximately 21 % O2 = 0.21 atm=16 cm Hg 𝑂𝑇𝑅 1550 𝑚𝐿 𝑚−2 𝑑𝑎𝑦 −1 𝑃= 𝑡ℎ𝑖𝑐𝑘𝑛𝑒𝑠𝑠 = ∗ 2.5 ∗ 10−2 𝑚𝑚 ∆𝑝 16 (𝑐𝑚 𝐻𝑔) P= 2.8*10-10 𝑚𝐿 𝑐𝑚 𝑐𝑚−2 𝑠 −1 𝑐𝑚 𝐻𝑔−1 = 2.8 barrer EXAMPLE WVTR of 290 mm thick OPLA at 37.8 oC and 100% RH as 36.7 g m-2 d-1. What is the permeability coefficient in barrer ? Saturated Vapor Pressure of Water @ 37.8 oC is 4.9167 cm Hg 𝑊𝑉𝑇𝑅 𝑃= 𝑡ℎ𝑖𝑐𝑘𝑛𝑒𝑠𝑠 ∆𝑝 P= 1.88 10−11 kg 𝑚 𝑚−2 𝑠 −1 𝑃𝑎 −1 = 3.12x106 barrer WVTR WVTR could also be expressed based on the relative humidity difference. In that case WVTR will have units of ; kg.m-2.s-1 OR g.m-2.d-1 𝑊𝑉𝑇𝑅 𝑃= 𝑡ℎ𝑖𝑐𝑘𝑛𝑒𝑠s ∆𝑹𝑯 PERMEABILITY OF MULTILAYER PACKAGING MATERIALS These calculations will be valid for dry gas but not for moisture-sensitive materials. For moisture-sensitive material permeability will depend on the average partial pressure at the center thus equation should be modified. Paper (80%): to provide strength and stiffness Polyethylene (15%): to make packages liquid tight and to provide a barrier to microorganisms Aluminium foil (5%): to keep out air, light, and off flavors - all the things that can cause food to deteriorate Case PET Cola Bottle: What is the loss of carbondioxide and water from a closed bottle of cola in 1 year? Given information: Volume: 1 liter Wall thickness: 0.5 mm Surface area: 0.1 m2 CO2 Transmission Rate: 320 ml/(m2 day bar) for 25 µm PET Amount of CO2 : 4 liter gas/liter cola Water Transmission Rate: 20 g/(m2 day) at RH of 100% Pressure in bottle: 4 bar Cola is store in a RH of 50% CO2: Transmission Rate for 0.5 mm: 320/20 = 16 ml/(m2 day bar) For bottle with area 0.1 m2 and pressure 4 bar: Loss is: 16 x 0.1 x 4 = 6.4 ml/day; in 1 year = 6.4 x 365= 2.3 liter = >50% Water: Transmission Rate for 0.5 mm: 20/20 = 1 g/(m2 day) For bottle with area 0.1 m2 and assumed RH = 50%: Loss is: 1 x 0.1 x 0.5 = 0.05 g/day; in 1 year = 0.05 x 365= 18 g =