Food Packaging PDF
Document Details
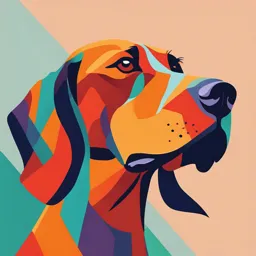
Uploaded by SmoothestCrocus3287
Tags
Summary
This document provides an overview of food packaging, focusing on general definitions, packaging materials, and factors influencing polymer structures. It explores concepts such as crystallinity, physical transitions, and additives in plastics.
Full Transcript
Food Packaging General Definitions Packaging Materials (Plastic ; Glass, Paper ; Metal) Packaging of different Food Categories Modified Atmosphere Packaging (MAP) ; Aseptic Packaging Active & Intelligent Packaging Engineering Design for Food Packages Packaging and Food Safety & Quality ( Migration...
Food Packaging General Definitions Packaging Materials (Plastic ; Glass, Paper ; Metal) Packaging of different Food Categories Modified Atmosphere Packaging (MAP) ; Aseptic Packaging Active & Intelligent Packaging Engineering Design for Food Packages Packaging and Food Safety & Quality ( Migration ; Scalping) Edible Packaging New Trends in Food Packaging Sustainability and Packaging and Legislative Aspects Factors Influencing Polymer Structures and Related Properties * 1. Molecular Structure (Classification of Polymers, Polymerization Process) 2. Molecular Weight 3. Density 4. Crystallinity 5. Physical Transitions in Polymers 6. Chemical Structure (Polyolefins, Copolymers of Ethylene, Substituted Olefins, Polyesters, Polycarbonates, Polyamides, Acrylonitriles) 7. Additives in Plastics (Processing Additives, Plasticizers, Antiaging Additives, Surface Property Modifiers, Optical Property Modifiers, Foaming Agents) PLASTIC POLYMERS THERMOPLASTICS THERMOSETTINGS characterized by extremely long characterized by the presence of molecules with saturated carbon- cross-links. carbon backbones. set into a given network when molecular chains can move manufactured. independently. gradually soften with increasing do not melt on heating but finally temperature and finally melt. blister and char. may be readily molded or cannot be subsequently remolded extruded. to a new shape. CRYSTALLINITY When a low MW material such as a metal crystallizes from the molten state, nucleation occurs at various points, from each of which a crystal or grain grows. Likewise, when a molten crystallizable polymer is cooled, crystallization spreads out from individual nuclei. However, instead of individual grains, a considerably more complex structure develops from each nucleus. The degree of crystallinity is an important factor affecting polymer properties. The great length of polymer chains means that a certain amount of entanglement normally occurs and this prevents complete crystallization on cooling as in the case of metals. This phenomenon is due to the difficulty of aligning every portion of each chain of the polymer. Thus, crystallinity in plastics consists of thousands of small “islands” of crystalline regions surrounded by unordered or amorphous (without structure) material CRYSTALLINITY The crystalline areas are known as crystallites. Unlike crystals of small molecules, crystallites are not composed of whole molecules or molecules of uniform size. High MW, narrow MWD and linearity in the polymer backbone can yield high crystallinity. The crystallization rate is enhanced by the presence of impurities such as catalysts, fillers and pigments. CRYSTALLINITY Stretching a film orients the crystallites and realigns other molecules or segments of molecules, causing the total crystallinity of the film to increase. Such oriented films are generally tougher than either amorphous or unoriented crystalline materials. Orientation and crystallinity are related in that only polymers that are capable of crystallization can be oriented. Non-crystalline, amorphous polymers have no melting point. They simply soften when heated, in much the same way as glass. To summarize, high shear during processing and rapid cooling inhibit crystallinity; annealing and orientation enhance it. CRYSTALLINITY Transparency of unfilled plastics is a function of crystallinity, with non-crystalline polymers such as PS and PC (polycarbonate) having excellent transparency. Other polymers range from cloudy to opaque, depending on the degree of crystallinity PHYSICAL TRANSITIONS IN POLYMERS Noncrystalline (amorphous) polymers are characterized by a glass transition at a temperature called the glass transition temperature (Tg). Crystalline polymers are characterized by a melting transition at a temperature called the crystalline melting temperature (Tm). Tm and Tg are important parameters that define the upper and lower temperature limits for numerous applications, especially for semicrystalline polymers. At a sufficiently high temperature, a thermoplastic polymer is a liquid. In this state, it consists of an amorphous mass of wriggling molecular chains. As it is cooled, the thermal agitation decreases, and, at the Tm, the polymer may crystallize. The Glass Transition Temperature Tg is the main transition temperature found in amorphous polymers. The underlying molecular process is that frozen backbone sequences begin to move at the Tg. Therefore, Tg is determined not only by the main chain architecture but also by its immediate surroundings. Chain ends and low-MW plasticizers lower the Tg of a polymer; a sufficiently large number of cross-links will increase Tg. Above Tg, a few of the carbon atoms in each chain can still move with relative freedom, but below Tg, nearly all the carbon atoms become fixed, and only side groups or very short chain sections can change position. PHYSICAL TRANSITIONS IN POLYMERS For crystalline polymers, an approximate relationship between Tg and Tm is 2 Tg ≈ Tm (for unsymmetrical chains) 3 and 1 Tg ≈ T (for symmetrical chains) 2 m when both temperatures are expressed in Kelvin. PHYSICAL TRANSITIONS IN POLYMERS An important exception to this occurs with copolymers. Copolymerization also tends to broaden the temperature range over which Tg occurs, due to differences in chemical composition among the copolymer chains in the same sample. Generally, the crystalline copolymer is expected to have a lower melting temperature than the corresponding homopolymer. Ordered copolymers exhibit different transition behavior from random copolymers, generally showing a single, characteristic Tg and, if crystallizable, a single, sharp melting temperature. PHYSICAL TRANSITIONS IN POLYMERS The physical properties of a thermoplastic polymer depend on the values of Tm and Tg relative to room temperature. If both Tm and Tg lie below room temperature, the polymer is a liquid. If room temperature lies between Tm and Tg, the polymer is either a very viscous supercooled liquid or s crystalline solid. If both Tm and Tg are above room temperature, an amorphous polymer is glassy in nature, tending to be brittle. Other properties such as stiffness (modulus), refractive index, dielectric properties, gas permeability and heat capacity all change at Tg PHYSICAL TRANSITIONS IN POLYMERS The glass transition temperatures of the majority of the commercially important crystallizable polymers lie below 25 °C. The Tg values are low for flexible, linear polymer such as the PEs, and relatively high for stiff chain polymers such as PET (poly(ethylene terephthalate)) and PC, which require higher temperatures for the onset of molecular motions necessary for the glass transitions. PHYSICAL TRANSITIONS IN POLYMERS Bulky side groups decrease the mobility of the chain and, thus, raise Tg. For instance, substitution of alternate hydrogens in the PE with methyl groups to give PP, or with phenyl groups to give PS, increases Tg from -110°C to -18°C and +100°C, respectively. Molecular symmetry tends to lower Tg. For instance, PVC has a Tg of 87 °C, whereas for PVdC the Tg is -17°C. Because bulky side groups tend to restrict molecular rotation, they also raise Tm. For example, the CH3 side groups on PP are larger than the H atoms found on LDPE, and, therefore, the Tms are 176°C and 115°C, respectively. The presence of polar side groups such as Cl, OH and CN even though not excessively large, leads to significant intermolecular bonding forces and relatively high Tms. For instance, the Tm and Tg for PVC is 212°C and for PAN (poly(acrylonitrile)) 317°C. RESIN Identification CODES * The ASTM International Resin Identification Coding System, often abbreviated as the RIC, is a set of symbols appearing on plastic products that identify the plastic resin out of which the product is made It was developed originally by the Society of the Plastics Industry (now SPI: The Plastics Industry Trade Association) in 1988, but has been administered by ASTM International since 2008 Polyolefins* Olefin means oil-forming and was originally the name given to ethylene. Today polyolefin is a common term in the plastics industry and refers to the family of plastics based on ethylene and propylene. The term alkene is used for hydrocarbons containing a carbon-carbon double bond, for example, ethylene, propylene and octene. Polyolefins form an important class of thermoplastics and include low, very low, linear, medium and high density PEs and polypropylene (PP). Industry commonly divides PEs into the following broad categories based on density: HDPE 940-975 kg m-3, MDPE 926-940 kg m-3, LDPE 915-940 kg m-3, LLDPE 915-925 kg m-3, VLDPE 880-915 kg m-3. Linear low-density polyethylene (LLDPE) and very low density polyethylene (VLDPE) Low Density Polyethylene This is the largest volume single polymer used in food packaging in both the film and blow molded form. It is a polymer of ethylene, a hydrocarbon gas available in large quantities as a byproduct of petroleum refining and other processes. Increasing quantities are now also being produced by the catalytic dehydration of ethanol produced by the fermentation of bio-based materials, especially sugarcane. LDPE Blown Film Extrusion Low Density Polyethylene The simplest structure for PE is a completely unbranched structure of –CH2- units as shown However, the vigorous nature of the high pressure process leads to a great deal of chain branching, with both short and long chains being formed. Low Density Polyethylene The branch contains a terminal methyl (-CH3-) group. A convenient way of characterizing branching is by the number of methyl groups per 1000 carbon atoms. HDPE short chain branches (SCB) and LDPE with long-chain Branched PE branches (LCB) are good examples for the schematic representation of linear and branched polymers. The branch chains prevent close packing of the main polymer chains, resulting in the production of PEs of relatively low density (typically 910-940 kg m-3). MWs also tend to be relatively low. The great length of the polymer chains results in a certain amount of entanglement that prevents complete crystallization on cooling. Low Density Polyethylene The crystallinity of LDPE usually varies between 55% and 70%. The softening point is also affected by chain branching. The softening point of LDPE is just below 100°C, thus precluding the use of steam to sterilize it in certain food packaging applications. While it is an excellent barrier to water and water vapor, it is not a good barrier to gases. It has excellent chemical resistance, particularly to acids, alkalis and inorganic solutions, but is sensitive to hydrocarbons, halogenated hydrocarbons, oils and greases. These latter compounds are absorbed by the LDPE, which then swells. Low Density Polyethylene At the present time, there are many hundreds of grades of LDPE available, most of which differ in their properties in one way or another. Differences arise from the following variables: 1. Variation in the degree of short chain branching in the polymer, 2. Variation in the degree of long chain branching, 3. Variation in the average MW, 4. Variation in the molecular weight distribution (which may in part depend on the long-chain branching), 5. Presence of small amounts of comonomer residues, 6. Presence of impurities or polymerization residues, some of which may be combined with the polymer. Linear Low Density Polyethylene The term linear in LLDPE is used to imply the absence of long chain branches (LCBs). LLDPE has a similar molecular structure to HDPE and although virtually free of LCBs, it does contain numerous short side chains. These arise as a result of copolymerizing ethylene with a small amount of a higher alkene (α- olefin) such as butene. Linear Low Density Polyethylene Due to the linearity of its molecules, LLDPE is stiffer but less transparent than LDPE, resulting in an increase of 10°C-15°C in the melting point of LLDPE compared to LDPE. The linearity provides strength, while the branching provides toughness. LLDPE combines the main features of both LDPE and HDPE, a major feature being that its MWD is narrower than that of LDPE. Generally, the advantages of LLDPE over LDPE are improved chemical resistance, improved performance at low and high temperatures, higher surface gloss, higher strength at a given density, better heat sealing properties. Very Low Density Polyethylene VLDPE (sometimes referred to as ultralow density polyethylene or ULDPE) is a subclass of LLDPE with a density 4 mol% and a crystallinity of