Chemistry of Engineering Materials PDF
Document Details
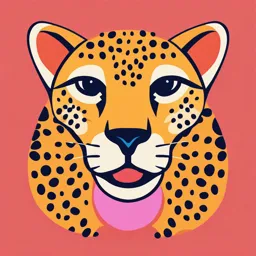
Uploaded by RomanticCedar
Central Philippine University
Tags
Summary
This document provides an introduction to the chemistry of engineering materials. It covers fundamental concepts of crystal structure and metallic crystal structures, including different types of crystal systems and their properties.
Full Transcript
T H E C H E M I ST RY O F E N G I N E E R I N G MAT E R IA L S INTRODUCTION Important for our existence, day to day needs and even our survival Gold was the first metal used by mankind followed by copper Areas of science focusing on the study of materials: 1. Engineering Materials 2. Material...
T H E C H E M I ST RY O F E N G I N E E R I N G MAT E R IA L S INTRODUCTION Important for our existence, day to day needs and even our survival Gold was the first metal used by mankind followed by copper Areas of science focusing on the study of materials: 1. Engineering Materials 2. Material Science 3. Materials Engineering Atomic Arrangement of Crystalline and Amorphous Silicon Dioxide BASIC CONCEPTS OF CRYSTAL STRUCTURE BASIC CONCEPTS OF CRYSTAL STRUCTURE Lattice Point Properties of crystalline solids depend on the crystal structure of the material, or the manner in which atoms, ions, or molecules are spatially arranged Space Lattice or Lattice is a periodic arrangement of points in space with respect to three dimensional network of lines Lattice Point – each atom represented by a point, where Lattice Space the network lines intersect Representation of lattice Lattice Array – arrangement of lattice points point, array and space Lattice Space – the space covered by the lattice points BASIC CONCEPTS OF CRYSTAL STRUCTURE Unit Cell - Tiny blocks formed by arrangements of small groups of atoms Chosen to represent the symmetry of a crystal structure, and may be defined as: 1. Finite representation of infinite lattice 2. Small repeat entity Unit Cell 3. Basic structural unit Representation of unit 4. Building block of crystal structure cell BASIC CONCEPTS OF CRYSTAL STRUCTURE Lattice Parameters Six lattice parameters are: a, b, c, α, β, and γ. Typically in the order of few Angstroms (Å), equal to 0.1 nanometer Based on the length equality or inequality and the orientation (the angles between them, α, β, and γ) a total of 7 crystal systems can be defined Representation of dimensions of a unit cell BASIC TYPES OF CRYSTAL SYSTEMS BASIC TYPES OF CRYSTAL SYSTEMS BASIC CONCEPTS OF CRYSTAL STRUCTURE Bravais Lattices 14 types of crystal systems where presented, with the inclusion of the property of Centering Types of Centering 1. Face-Centered 2. Body-Centered 3. Base-Centered METALLIC CRYSTAL STRUCTURES Simple Cubic (SC) Most elementary crystal structure with three mutually perpendicular axes arbitrarily placed through one of the corners of a cell. Each corner is occupied with one atom Only polonium is packed in this manner Packing density is relatively low METALLIC CRYSTAL STRUCTURES Body-Centered Cubic (BCC) Atoms are arranged at the corners of the cube with another atom at the cube center. Has 2 atoms in one unit cell. Examples: alpha iron, chromium, tungsten, tantalum, and molybdenum Center and corner atoms touch one another along cube diagonals, and unit cell length a and atomic radius R are related through: 4𝑅 𝑎= 3 METALLIC CRYSTAL STRUCTURES Face-Centered Cubic (FCC) Atoms are arranged at the corners and center of each cube face of the cell. Atoms are assumed to touch along face diagonals. Has 4 atoms in one unit cell. Examples: aluminum, copper, gold, lead, silver and nickel Spheres or ion cores touch one another across a face diagonal; the cube edge length a and the atomic radius R are related through: 𝑎 = 2𝑅 2 METALLIC CRYSTAL STRUCTURES Hexagonal Closed-Pack (HCP) Cell of an HCP lattice is visualized as a top and bottom plane of 7 atoms, forming a regular hexagon around a central atom. In between, these planes is a half-hexagon of 3 atoms. There are two lattice parameters in HCP, a and c, representing the basal and height parameters respectively. Has 6 atoms per unit cell. Examples: zinc, lithium, magnesium, beryllium Volume is computed as 3 3𝑎2 𝑐 𝑣= 2 CRYSTALLOGRAPHIC DIRECTIONS Crystallographic Direction a line or vector directed between two points directions are given by specifying the coordinates (x, y, z) of a point on a vector (Pxyz) passing through the origin Notation: [xyz] CRYSTALLOGRAPHIC DIRECTIONS To determine a direction of a line in the crystal: 1. Find the coordinates of the two ends of the line and subtract the coordinates (Head – Tail) OR draw a line from the origin parallel to the line and find its projection lengths on x, y and z axis in terms of the unit vectors a, b and c. 2. Convert fractions, if any, in to integers and reduce to lowest term. 3. Enclose in square brackets [uvw] CRYSTALLOGRAPHIC DIRECTIONS Directions in Hexagonal Crystal If [XYZ] to [xyuz] Notation: [xyuz] x = (2X-Y)/3 Conversion from four notation to three y = (2Y-X)/3 notation: u = -(x+y) or If [xyuz] to [XYZ] u = -(X+Y)/3 X = (x-u) z=Z Y = (y-u) Z=z PROPERTIES OF CRYSTALS : ASSIGNMENT 1 Define the following crystal properties, and provide the equation for each, if there is any. Compute each property for SC, FCC, BCC and HCP. COORDINATION NUMBER ATOMIC PACKING FACTOR (APF) PLANAR DENSITY LINEAR DENSITY PROPERTIES OF CRYSTALS Coordination number is the number of nearest neighbor atoms or ions surrounding an atom or ion. For FCC and HCP systems, the coordination number is 12. For BCC, it’s 8. For SC, coordination number is 6. Atomic packing factor (APF) or packing efficiency indicates how closely atoms are packed in a unit cell and is given by the ratio of volume of atoms in the unit cell and volume of the unit cell. APF = "Volume of atoms" /"Volume of unit cell" SC = 0.52 or 52% FCC = HCP = 0.74 or 74% (26% void space in unit cell) BCC = 0.68 or 68% PROPERTIES OF CRYSTALS Planar density (PD) refers to density of atomic packing on a particular plane. Number of atoms on a plane Planar Density = Area of plane PROPERTIES OF CRYSTALS LINEAR DENSITY Linear density (LD) is the number of atoms per unit length along a particular direction Number of atoms on the direction vector Linear Density = Length of the direction vector METALS INTRODUCTION employed for various engineering purposes and requirements iron is the most popular metal in the field of engineering ALL the metals have a crystalline structure ALLOY An alloy is a mixture or compound of two or more elements, at least one of which is metallic Alloying enhances some properties, as required by engineering applications, such as strength and hardness in comparison to pure metals Classified into solid solution and intermediate phase ALLOY Solid solution is an alloy in which one element is dissolved in another to form a single-phase structure In a solid solution, the solvent or base element is metallic, and the dissolved element can be either metallic or nonmetal Solid solutions can be Substitutional Solid Solution or Interstitial Solid Solution ALLOY Substitutional solid solution - atoms of solvent element are replaced in its unit cell by dissolved element. Interstitial solid solution - atoms of dissolving element fit into vacant spaces between base metal atoms Substitutional solid solution (left) and Interstitial in the lattice structure. solid solution (right) ALLOY Intermediate phases - There are usually limits to the solubility of one element in another. When the amount of the dissolving element in the alloy exceeds the solid solubility limit of the base metal, a second phase forms in the alloy. The term intermediate phase is used to describe it because its chemical composition is intermediate between the two pure elements. Its crystalline structure is also different from those of the pure metals. IMPORTANCE OF METALS High stiffness and strength – can be alloyed for high rigidity, strength, and hardness Toughness – capacity to absorb energy better than other classes of materials Good electrical conductivity – metals are conductors Good thermal conductivity – conduct heat better than ceramics or polymers Cost – the price of steel is very competitive with other engineering materials METALS USED IN MANUFACTURING PROCESS Cast Metal - starting form is a casting Wrought Metal - the metal has been worked or can be worked after casting Powdered Metal - starting form is very small powders for conversion into parts using powder metallurgy techniques CLASSIFICATION OF METALS FERROUS METALS These metals contain iron as main constituent Ferrous metals are classified as cast iron, wrought iron, and steel depending upon ingredients and percentage of carbon content NON-FERROUS METALS These metals practically do not contain iron FERROUS METALS Cast Iron (C.I.) Cast Iron contains a higher percentage of carbon ranging from 2 to 4.23 Cast iron is manufactured by melting of pig iron with coke and lime stone. The furnace used is known as CUPOLA. Properties of Cast Iron 1. It can be hardened by heating and sudden cooling, but it cannot be tempered. 2. It does not rust easily. 3. It is fusible. 4. It is hard and at the same time brittle. FERROUS METALS Cast Iron (C.I.) Classification 1. Grey Cast Iron - The carbon content is about 3% ad when fractured gives a grey appearance. It is soft and readily melts. Its strength is weak and is used for casting cylinders, pistons, manholes etc. 2. White Cast Iron - Its carbon content is 2.0 to 2.5%. It contains carbon in chemical form and on fracturing gives silver white luster. It is hard, not workable on machines and is used for preparing pump liners, drawing dies etc., FERROUS METALS Cast Iron (C.I.) Classification 3. Chilled Cast Iron - Its carbon content is 3.3%. It is produced by casting the molten metal against a metal chiller to obtain a surface of white cast iron. This is hard to a certain depth from the outer surface, which indicates the white iron. The inner portion of the body is made up of grey iron which is soft. It is used for manufacturing rail car wheels, dies, sprockets etc. FERROUS METALS Cast Iron (C.I.) 4. Malleable Cast Iron - Its carbon content is 2.3%. The composition of this is so adjusted that it becomes malleable. It is done by extracting a portion of carbon from cast iron. It has high field strength and used for manufacture of automobile and railway equipment such as rail cars, crank shafts gear boxes etc. 5. Toughened Cast Iron - It is obtained by melting cast-iron with wrought iron scrap. The proportion of wrought-iron scrap is about 1/4 to 1/7th of cast-iron. FERROUS METALS Wrought Iron It is an almost pure iron. Its carbon content is 0.15%. It is manufactured by the following stages: Refining → Puddling → Shingling → Rolling FERROUS METALS Wrought Iron Properties 1. It is soft at white stage of heat. It can be easily forged and welded. 2. It is ductile, malleable and tough. 3. Its melting point is 1500°C. 4. It is resistant to corrosion. FERROUS METALS Steel Steel is defined as the iron alloy with a carbon content of up to 2.0%. Types of steel include low carbon steel or mild steel, medium carbon steel, and high carbon steel. FERROUS METALS Steel Carbon Properties Uses Content Low Carbon or Mild 0.10 to 0.3% It can be easily hardened and tempered Sheets, Tin Plates It is malleable and ductile It can be forged and welded It rusts easily Specific gravity is 7.8 Medium Carbon 0.3 to 0.6% These steels have high strength, toughness, Boiler plates, Railway tyres, hardness and stiffness pressing dies High Carbon 0.6 to 1.50% It can be easily hammered and tempered Springs, Hammers, Drills, It can be magnetize permanently Chisel It is granular in structure Specific gravity is 7.9 FERROUS METALS Alloy Steel Steel, to which elements other than carbon are added in sufficient quantity, in order to obtain special properties, is known as alloy steel. Examples of alloy steels include chromium steel, cobalt steel, manganese steel, tungsten steel, vanadium steel and Nickel steel. FERROUS METALS Alloy Steel Alloying elements added Properties Uses Chromium Steel Chromium up to 0.9%, Highly ductile, can be easily Used for locomotive springs, Vanadium up to 0.15% worked and welded pistons and bolts Cobalt Steel Cobalt is added to high Possesses magnetic properties Used for making permanent carbon steel magnet with strong magnetic field Manganese Steel Manganese up to 1.90% It is hard, strong and ductile. It Used for gears gives resistance to abrasion. Tungsten Steel or Tungsten content up to 7% It is hard and maintains cutting Used for lathe tools, drill High Speed Steel power at high temperature cutters Nickel Steel Nickel up to 3.5% It is hard and ductile Used for boiler plates, structural steel propeller shafts Vanadium Steel Vanadium content up to 0.2% Strong and ductile, elastic limit Used for automobile parts, is high, resists shocks springs NON-FERROUS METALS Aluminum It is a very light and useful non-ferrous metal. It Uses of Aluminum is produced mainly from bauxite which is 1. For manufacturing of electrical conductors. hydrated oxide of aluminum. 2. Making alloys. Properties of Aluminum 3. For manufacture of cooking utensils, surgical 1. a tin white metal. instruments, etc. 2. good conductor of heat and electricity. 4. For making parts of air crafts. 3. malleable, ductile and very light. 5. In manufacture of paints 4. highly electro positive element. NON-FERROUS METALS Copper Copper is one of the most useful non-ferrous Uses of Copper metals. It occurs in nature in a free state as well as 1. For the manufacture of electrical cables and combined state. wires and lightning conductors. Properties of Copper 2. For making alloys. 1. Bright shining metal of reddish color. 3. For house hold utensils. 2. high tensile strength. 4. For bolts and nuts. 3. very malleable and ductile. 5. For tubes, etc. 4. a good conductor of heat and electricity. 5. It melts at 1083°C NON-FERROUS METALS Tin Tin is a very important non-ferrous metal which is Uses of Tin obtained from ore, tin pyrites or tinstone. 1. Used for plating. Properties of Tin 2. For lining and lead pipes. 1. When a bar of tin is bent, a peculiar noise 3. For making alloys and solders. takes place which is known as a cry of tin. 4. For making trunks, boxes, cans, pans, etc. 2. white metal with brilliant luster. 3. soft and malleable. 4. withstands corrosion due to acids. 5. melts at 232°C. NON-FERROUS METALS Zinc Zinc is one of the most extensively used non- 4. can be rolled into sheets. ferrous metals. Occurs in wide variety of 5. melting point is 420°C combination in nature. Zinc is manufactured from ores by roasting and subsequent distillation with carbon. Uses of Zinc Properties of Zinc 1. Used for galvanizing steel sheets. 1. bluish white metal. 2. For making roofing sheets, pipes, ventilators. 2. easily fused. 3. Used in brass making. 3. brittle when cold, but malleable at a high 4. For making negative poles of batteries. temperature. NON-FERROUS METALS Lead Lead is extracted from galvena ores. It is a very Uses of Lead cheap, but useful nonferrous metal. It is produced 1. For making shots and bullets. from the ores by smelting in a reverberatory furnace. 2. Used for making gas pipes. Properties of Lead 3. Printers type letters. 1. very soft, heavy blush grey in color. 4. Flushing tank. 2. can be easily cut with a knife. 5. Roof covers. 3. makes impression on paper. 4. melting point is 327° and boiling point is 1600°C. SUPER ALLOYS Three Groups of Superalloys 1. Iron-based alloys - in some cases iron is less than 50% of total composition 2. Nickel-based alloys - better high temperature strength than alloy steels 3. Cobalt-based alloys - ~ 40% Co and ~ 20% chromium SUPER ALLOYS Importance: Room temperature strength properties are good in comparison to other metals, but not outstanding High temperature performance is excellent – tensile strength, hot hardness, creep resistance, and corrosion resistance at very elevated temperatures Operating temperatures often in the vicinity of 1100°C (2000°F) Have many applications Example is that it is used in systems in which operating efficiency increases with higher temperatures e.g., gas turbines, jet and rocket engines, steam turbines, and nuclear power plants SUPER ALLOYS Importance: Room temperature strength properties are good in comparison to other metals, but not outstanding High temperature performance is excellent – tensile strength, hot hardness, creep resistance, and corrosion resistance at very elevated temperatures Operating temperatures often in the vicinity of 1100°C (2000°F) Have many applications Example is that it is used in systems in which operating efficiency increases with higher temperatures e.g., gas turbines, jet and rocket engines, steam turbines, and nuclear power plants METAL PROCESSING processing of metals should be carried out carefully Affects the mechanical properties of metals Grain Size Effect Grains in metals tend to grow larger as the metal is heated metals with small grains are stronger but they are less ductile METAL PROCESSING Quenching and Hardening Most steels may be hardened by heating and cooling rapidly (quenching) metals are quenched in water or oil Produce very hard but brittle metal Annealing a softening process in which metals are heated and then allowed to cool slowly Tempering heating a hardened metal and allowing it to cool slowly produce a metal that is still hard and less brittle results formation of small Fe3C (iron carbide or cementite) precipitates in the steel, which provides strength METAL PROCESSING Cold Working refers to the process of strengthening a metal by changing its shape without the use of heat also known as plastic deformation or work hardening, involves strengthening a metal by changing its shape. the metal is subjected to mechanical stress so as to cause a permanent change to the metal's crystalline structure METAL PROCESSING Cold Working METAL MANUFACTURING: PRODUCTION CASTING Melting and molding - molten metal is poured into a mold cavity where, the metal take on the shape of the cavity once it cools For small intricate parts (Dies, jewelry, plaques, and machine components ) SHOULD NOT be used for products that require high strength, high ductility, or tight tolerances a. Expendable Mold Casting - the mold must be destroyed in order to remove the part b. Permanent Mold Casting - the mold is fabricated out of a ductile material and can be used repeatedly. METAL MANUFACTURING: PRODUCTION Powder Processing treats powdered metals with pressure (pressing) and heat (sintering) to form different shapes Powdered metallurgy (processing of powdered metals) is known for its precision and output quality – it keeps tight tolerances and often requires no secondary fabrications metal powder is compacted into the desired shape and heated to cause the particles to bond into a rigid mass. incredibly costly and generally only used for small, complex parts Powder processing is NOT appropriate for high-strength applications. METAL MANUFACTURING: PRODUCTION Powder Processing treats powdered metals with pressure (pressing) and heat (sintering) to form different shapes Powdered metallurgy (processing of powdered metals) is known for its precision and output quality – it keeps tight tolerances and often requires no secondary fabrications metal powder is compacted into the desired shape and heated to cause the particles to bond into a rigid mass. incredibly costly and generally only used for small, complex parts Powder processing is NOT appropriate for high-strength applications. METAL MANUFACTURING: PRODUCTION Forming raw metal (usually in sheet metal form) is mechanically manipulated into a desired shape Unlike casting, metal forming allows for higher strength, ductility, and workability for additional fabrications METAL MANUFACTURING: FABRICATION Deformation bending, rolling, forging, and drawing Deformation processes include metal forming and sheet metalworking Application of stresses to the piece which exceed the yield stress of the metal There are two types of deformation processes: a. Bulk Processes - characterized by large deformations and shape changes surface area to volume ratio is relatively small include rolling, forging, extrusion and wire and bar drawing. b. Sheet Metalworking - performed on metal sheets, strips and coils having a high surface area to volume ratio. use a punch and die to form the workpiece Bending, drawing and shearing METAL MANUFACTURING: FABRICATION Machining any fabrication method that removes a section of the metal also known as material removal processing Cutting, shearing, punching, and stamping are all common types of machining fabrication. a. Machining Operations - These are cutting operations using cutting tools that are harder than the metal of the product. They include turning, drilling, milling, shaping, planning, broaching and sawing. b. Abrasive Machining - In these methods material is removed by abrasive particles that normally form a bonded wheel. Grinding, honing and lapping are included in this category. c. Nontraditional Processes - These methods use lasers, electron beams, chemical erosion, electric discharge and electrochemical energy instead of traditional cutting and grinding tools When planning for machining in your supply chain, hardening processes should happen AFTER machining processes. Hardened metals have a high shear strength and are more difficult to cut. METAL MANUFACTURING: FABRICATION Joining Joining, or assembly, is one of the last steps of the metal manufacturing process includes welding, brazing, bolting, and adhesives. Assembly can be done by machine or by hand, where multiple parts are connected either permanently or semi-permanently to form a new entity. Finishing includes everything from galvanization to powder coating, and can take place throughout the manufacturing process MECHANICAL PROPERTIES OF MATERIALS Strength It is the capacity of the material to withstand the breaking, bowing, or deforming under the action of mechanical loads on it. Elasticity It is the property of a material to come back to its original size and shape even after the load stops acting on it. Plasticity It is the property of a material that makes it to be in the deformed size and shape even after the load stops acting on it. Ductility It is the property of a material that allows it to deform or make into thin wires under the action of tensile loads plastically. Tensile strength It is the property of a material that allows it to deform under tensile loading without breaking under the action of a load STRENGTH OF MATERIALS STRESS NORMAL STRESS the strength of a material per unit area or unit either tensile stress or compressive stress strength Tension or tensile force applied results to the force on a member divided by area, which Tensile Stress (increase in length) carries the force Compression or compressive force applied maximum stress in tension or compression results to Compressive Stress (decrease in occurring over a section normal or length) perpEndiculat to the load expressed in psi, N/mm2 or Mpa 𝑭 (𝒇𝒐𝒓𝒄𝒆) 𝝈= 𝑨 (𝒂𝒓𝒆𝒂) STRENGTH OF MATERIALS SHEARING STRESS BEARING STRESS results from forces applied parallel to the area of the contact pressure between the separate bodies resisting force differs from compressive stress, as it is an internal also known as tangential stress stress caused by compressive forces 𝑽 (𝒔𝒉𝒆𝒂𝒓𝒊𝒏𝒈 𝒇𝒐𝒓𝒄𝒆) 𝑷𝒃 𝝉= 𝝈𝒃 = 𝑨 (𝒂𝒓𝒆𝒂 𝒃𝒆𝒊𝒏𝒈 𝒔𝒉𝒆𝒂𝒓𝒆𝒅) 𝑨𝒃 STRENGTH OF MATERIALS SHEARING STRESS BEARING STRESS results from forces applied parallel to the area of the contact pressure between the separate bodies resisting force differs from compressive stress, as it is an internal also known as tangential stress stress caused by compressive forces 𝑽 (𝒔𝒉𝒆𝒂𝒓𝒊𝒏𝒈 𝒇𝒐𝒓𝒄𝒆) 𝑷𝒃 𝝉= 𝝈𝒃 = 𝑨 (𝒂𝒓𝒆𝒂 𝒃𝒆𝒊𝒏𝒈 𝒔𝒉𝒆𝒂𝒓𝒆𝒅) 𝑨𝒃 STRENGTH OF MATERIALS SIMPLE STRAIN Also known as unit deformation the ratio of the change in length caused by the applied force, to the original length Dimensionless 𝜹 (𝒄𝒉𝒂𝒏𝒈𝒆 𝒊𝒏 𝒍𝒆𝒏𝒈𝒕𝒉) 𝜺= 𝑳 (𝒊𝒏𝒊𝒕𝒊𝒂𝒍 𝒍𝒆𝒏𝒈𝒕𝒉 STRENGTH OF MATERIALS STRESS-STRAIN DIAGRAM the graph of quantities with the stress σ along the y-axis and the strain ε differs in form for various materials Metallic engineering materials are classified as either ductile or brittle Ductile material is one having relatively large tensile strains up to the point of rupture like structural steel and aluminum Brittle materials have a relatively small strain up to the point of rupture like cast iron and concrete. STRENGTH OF MATERIALS STRESS-STRAIN DIAGRAM Proportional Limit (Hooke's Law) “within the proportional limit, the stress is directly proportional to strain” 𝝈 𝜶 𝜺 𝒐𝒓 𝝈 = 𝒌𝜺 The constant of proportionality k is called the Modulus of Elasticity E or Young's Modulus and is equal to the slope of the stress-strain diagram from O to P. Then, 𝝈 = 𝑬𝜺 STRENGTH OF MATERIALS STRESS-STRAIN DIAGRAM Elastic Limit (E) limit beyond which the material will no longer go back to its original shape when the load is removed Elastic and Plastic Ranges The region in stress-strain diagram from O to P is called the elastic range. The region from P to R is called the plastic range Yield Point Yield point is the point at which the material will have an appreciable elongation or yielding without any increase in load STRENGTH OF MATERIALS STRESS-STRAIN DIAGRAM Ultimate Strength The maximum ordinate in the stress-strain diagram is the ultimate strength or tensile strength Rapture Strength Rapture strength is the strength of the material at rupture. This is also known as the breaking strength POLYMERS POLYMERS Polymers are engineering materials of very high molecular weight Created by joining together single units called monomer to make a single large monomer molecule One of the common examples of polymers is polyethylene, composed of repeating ethylene molecules (C2H4) extensively used in food packaging, clothing, home furnishing, transportation, medical devices, information technology, and other multifarious applications Natural polymers: silk, wool and cotton POLYMERS Polymerization is a chemical reaction in which monomer units combine to form larger molecules that contain repeating structural units Proper polymer names are derived from the individual structural unit. For example: Ethylene polymerizes to polyethylene Propylene polymerizes to polypropylene Styrene polymerizes to polystyrene Vinyl Chloride polymerizes to polyvinyl chloride Types of Polymerization a. Chain-reaction Polymerization or Addition Polymerization b. Step-Reaction Polymerization or Condensation Polymerization POLYMERS Chain-reaction Polymerization or Addition Polymerization In this reaction, monomers simply add to one another to form the polymer. Examples of this are LDPE, HDPE, PVC, PP, and PS POLYMERS Step-Reaction Polymerization or Condensation Polymerization Two monomers add in a condensation reaction under the release of water or another small molecule like hydrogen chloride Examples of this are PET, PU and PA POLYMERS Polymer Branching Polymer may have similar molecular weights, but can have different properties, which mainly depends on the degree of branching Branching can be distinguished by density of the polymer High-density polyethylene (HDPE) is resulted when highly linear polyethylene molecules can be closely packed Low-density polyethylene (LDPE) are highly branched molecular chains which cannot be packed closely together Linear-low density polyethylene (LLDPE) which has short controlled side branches POLYMERS Polymer Linking Homopolymer made by linking only one type of small molecule or monomer Copolymer two different types of monomers are joined in the same polymer chain Terpolymer Three different types of polymer are joined in one polymer chain Purpose of copolymerization: combine properties of different monomers to enhance and improve the property of the final product or material POLYMERS Polymer Linking Terpolymer Homopolymer Copolymer POLYMERS Polarity and Material Properties Polarity is the way in which atoms bond with each other. one of the atoms exerts a stronger attractive force on the electrons in the bond, resulting to unequal sharing of electrons A polymer is polar if it has positive and negative poles. If non, then it is nonpolar. Polar Materials Nonpolar Materials POLYMERS Polarity and Material Properties Factors Affecting Polymer Properties Polarity influences material properties such as: 1. Elemental Composition 1. Melting point 2. Polarity 2. Solubility 3. Size or Molecular Weight 3. Barrier 4. Shape of Molecule 4. Coefficient of Friction of Stickiness 5. Thermal History 6. Mechanical History POLYMERS MOLECULAR STRUCTURE AND PROPERTIES 1. Crystallinity Highly ordered molecular arrangements -“crystalline” Completely random arrangements - “amorphous A polymer with lower crystallinity will have better clarity 2. Molecular Weight Distribution Polymers don’t have a specific molecular weight The greater the molecular weight distribution, the easier to melt the plastic. 3. Viscoelasticity materials exhibiting both viscous and elastic characteristics also known as anelasticity, which is present in systems when undergoing deformation. Accordingly, rapid application of a load to plastic will cause it to bend, and will return to its original shape once the load is released. NANOMATERIALS NANOMATERIALS Nanotechnology is science, engineering, and technology conducted at the nanoscale, which is about 1 to 100 nanometers. Nanomaterials are nano-scale particles, tubes, rods, or fibres, used in healthcare, electronics, cosmetics, textiles, information technology and environmental protection Concept: “There’s Plenty of Room at the Bottom” by physicist Richard Feynman at an American Physical Society meeting at the California Institute of Technology (CalTech) on December 29, 1959 NANOMATERIALS PROPERTIES OF NANOMATERIALS 2. Chemical properties 1. Physical Properties Molecular structure Their size, shape, specific surface area, and ratio of Composition, including purity, and known impurities width and height or additives Whether they stick together Whether it is held in a solid, liquid or gas Size distribution Surface chemistry How smooth or bumpy their surface is Attraction to water molecules or oils and fats Structure, including crystal structure and any crystal defects How well they dissolve NANOMATERIALS HOW TO MANUFACTURE MATERIALS 1. Top-down - reduces large pieces of materials all the way down to the nanoscale, like someone carving a model airplane out of a block of wood. This approach requires larger amounts of materials and can lead to waste if excess material is discarded. 1. Bottom-up - creates products by building them up from atomic- and molecular-scale components, which can be time-consuming. NANOMATERIALS HOW TO MANUFACTURE MATERIALS: NEW PROCESSES Chemical vapor deposition - a process in which chemicals react to produce very pure, high- performance films Molecular beam epitaxy - one method for depositing highly controlled thin films Atomic layer epitaxy - a process for depositing one-atom-thick layers on a surface Dip pen lithography - a process in which the tip of an atomic force microscope is "dipped" into a chemical fluid and then used to "write" on a surface, like an old fashioned ink pen onto paper Nanoimprint lithography - a process for creating nanoscale features by "stamping" or "printing" them onto a surface Roll-to-roll processing - a high-volume process to produce nanoscale devices on a roll of ultrathin plastic or metal Self-assembly - describes the process in which a group of components come together to form an ordered structure without outside direction NANOMATERIALS POTENTIAL EFFECTS OF NANOMATERIALS In health. There is experimental evidence of a range of possible interactions with biological systems and health effects of manufactured nanoparticles. In experimental systems in the laboratory they can affect the formation of the fibrous protein tangles which are similar to those seen in some diseases, including brain diseases. Airborne particles might cause effects in the lungs but also on the heart and blood circulation similar to those already known for particulate air pollution. There is some evidence that nanoparticles might lead to genetic damage, either directly or by causing inflammation. In environment.Wider use of nanomaterials will lead to increases in environmental exposure. Since they are minute, little is known about how they may then behave in air, water or soil. As a result of their diversity, nanomaterials may have a wide range of effects. Some kill bacteria or viruses. Experiments so far have also shown possible harmful effects on invertebrates and fish, including effects on behavior, reproduction and development. There is less research to date on soil systems and terrestrial species, and it is not clear whether laboratory results relate to what may happen out in the real world. CHEMISTRY AND THE ENVIRONMENT COURSE CONTENT INTRODUCTION CHEMISTRY OF THE ATMOSPHERE CHEMISTRY OF WATER SOIL CHEMISTRY INTRODUCTION The environment is composed of the air we breathe, the land we live on, the water we drink and the climate around us. It is important that to ensure that the developments do not adversely affect our environment, while also ensuring mitigation of the damage that has already occurred INTRODUCTION Chemistry can help us to understand, monitor, protect and improve the environment around us. Tools and techniques are developed to enable us to measure air and water pollution. Software are used to model the trend of changes in climate from the past, to the present and for the future. CHEMISTRY OF THE ATMOSPHERE Formation of the Atmosphere 1. Primitive Atmosphere 4.5 Million years ago, the atmosphere was composed of Hydrogen, Helium, Ammonia and Methane, similar to the atmosphere of Saturn and Jupiter today 2. Secondary Atmosphere Volcanic activity from the Earth’s solid crust released gases such as water vapor, carbon dioxide and ammonia, similar to the atmosphere of Mars and Venus today As the Earth grew cooler, water vapor formed into clouds creating rain, which resulted to formation of the ocean Nitrogen was formed from the breakdown of ammonia due to sunlight Other gases formed were hydrogen, methane, and carbon monoxide in trace amounts, with hydrogen sulfide (H2S), sulfur dioxide (SO2), and chlorine CHEMISTRY OF THE ATMOSPHERE Formation of the Atmosphere 3. Oxidizing Atmosphere Oxygen was produced from a. dissociation of water vapor by strong ultraviolet radiation b. Photosynthesis of cyanobacteria c. Oxidation of metals present in the Earth’s surface Oxygen molecules started absorbing UV radiations resulting to the formation of ozone (O3) Formation of O3 made life on Earth possible CHEMISTRY OF THE ATMOSPHERE Atmosphere thick mixture of gases surrounding the Earth’s surface serves as a shield used to protect life, making our planet unique mixture of gases is forced to remain near the Earth’s surface due to gravity decrease in atmospheric material is observed with increase in altitude, until it gradually reaches outer space Karman Line an imaginary line which identifies the boundary between the atmosphere and outer space CHEMISTRY OF THE ATMOSPHERE History Important Discoveries of Atmospheric Elements Year of Discovery Element/Compound Discovered by 1750s Carbon dioxide Joseph Black 1766 Hydrogen Henry Cavendish 1772 Nitrogen Daniel Rutherford 1774 Oxygen Joseph Priestly and 1772 (published in 1777) Carl Wilhem Scheele 1840 Ozone Christian Friedrich Schonbein 1894 Argon Lord Rayleigh and William Ramsay CHEMISTRY OF THE ATMOSPHERE History Important Milestones of Atmospheric Chemistry Year Scientist Discovery Developed a spectrophotometer and started measurements of total- 1924 Gordon Dobson column ozone 1930 Sydney Chapman Described the theory that explains the existence of the ozone layer 1960 Arie Jan Haagen-Smit Described the emergence of the photochemical smog 1973 James Lovelock First detected chlorofluorocarbons (CFCs) in the Atmosphere Paul Crutzen Awarded jointly the Nobel Prize in Chemistry for their work in 1995 Mario Molina atmospheric chemistry, particularly concerning the formation and Frank Sherwood Rowland decomposition of ozone CHEMISTRY OF THE ATMOSPHERE Atmospheric Composition Atmospheric gases are generally classified by their amount and residence time. Residence Time the average amount of time that a gas spends in the atmosphere It can be estimated as the amount of the compound in the atmosphere divided by the rate at which this compound is removed from the atmosphere CHEMISTRY OF THE ATMOSPHERE Atmospheric Composition Classification of Atmospheric Gases Main components Trace gases Classification of Gases Constant Gases Nitrogen, oxygen and Other noble gases (neon, Based on the Amount argon helium, krypton, xenon) a. Major Components Variable Gases Carbon dioxide Other long-lived trace b. Trace Gases gases (methane, hydrogen, nitrous oxide) Based on the Residence Time Highly Variable Water vapor Other short-lived trace a. Constant Gases Gases gases (carbon monoxide, ozone, nitrogen dioxide, b. Variable Gases ammonia, sulfur dioxide, c. Highly Variable Gases hydrogen sulfide) CHEMISTRY OF THE ATMOSPHERE Atmospheric Gases Composition of the Atmosphere as Percent by Volume of Gases Nitrogen 78.08% Oxygen 20.95% Argon 0.934% Carbon 0.035% Dioxide Trace Gases 0.001% CHEMISTRY OF THE ATMOSPHERE Atmospheric Gases NITROGEN an inert gas fundamental to all living systems Removed from the atmosphere through a process called nitrogen fixation wherein atmospheric N2 is reduced to form ammonia. can be “fixed” or removed by soil bacteria or by lightning during precipitation. can be returned to the atmosphere by biomass combustion and denitrification. Denitrification – the reduction of nitrates to gaseous nitrogen by microorganisms in a series of biochemical reactions. CHEMISTRY OF THE ATMOSPHERE Atmospheric Gases OXYGEN Oxygen exchange between the atmosphere and biosphere is realized by photosynthesis and respiration. ARGON third most abundant gas in the atmosphere. Most of atmospheric argon is radiogenic 40Ar isotope derived from decay in 40K (potassium) in the Earth’s crust. CHEMISTRY OF THE ATMOSPHERE Atmospheric Gases WATER VAPOR is mostly concentrated on the lower atmosphere (about 90% of total atmospheric water vapor found in the lower 5 km atmospheric layer, and 99% of it found in the troposphere Saturation Level the capacity of air to hold water vapor dependent solely of air temperature. The higher the temperature, the greater amount of water vapor that can be held without condensation. important for radiation and energy budgets of the atmosphere, and in the formation of clouds and precipitation absorbs about 70% of the incoming shortwave solar radiation, and about 60% of outgoing long-wave radiations, making it the most significant greenhouse gas. Energy/Heat Budget – describes the net flow of energy into the Earth which is mainly in the form of shortwave radiation and infrared longwave radiation. CHEMISTRY OF THE ATMOSPHERE Atmospheric Gases CARBON DIOXIDE an important greenhouse gas since it has a strong absorption capacity of infrared and near-infrared radiation it is continually exchanged between the atmosphere and biosphere through photosynthesis and respiration Some of atmospheric CO2 are dissolved by the seas and oceans. increase in CO2 levels has been observed due to growing industries and human activities, like burning of fossil fuels, deforestation, and other forms of land-use change. Man-made activities like these contributed to global warming with the increase of the greenhouse effect. CHEMISTRY OF WATER WATER a chemical compound of hydrogen and oxygen contains strong covalent bonds that hold two hydrogen atoms and one oxygen atom together bonded as H-O-H with a bond angle of 105° between the two hydrogen atoms in liquid water and a large angle of 109° 6’ for ice Three isotopes of hydrogen and three of oxygen exist in nature, therefore 18 varieties of water molecules are possible CHEMISTRY OF WATER WATER electrons are unequally shared between the oxygen and hydrogen atoms hydrogen atoms are slightly positive in charge while the oxygen atom is slightly negative in charge, forming hydrogen bonds Hydrogen Bond – a weak bond between polar compounds where hydrogen atom of one molecule is attracted to an electronegative atom of another molecule. Water can form up to 4 hydrogen bonds. CHEMISTRY OF WATER WATER expected to be gaseous at room temperature yet due to the many hydrogen bonds it contains, it is liquid. Liquid water has higher coordination number than ice Coordination Number – the average number of nearest neighbor atoms with respect to a central atom. CHEMISTRY OF WATER WATER Specific Heat - 1 cal/g/°C Latent Heat of Fusion – the energy required to convert 1 gram of ice to water at 0 °C, estimated at 80 calories Latent Heat of Vaporization – the energy required to convert 1 gram of liquid water into vapor at 100 °C, estimated at540 calories CHEMISTRY OF WATER PROPERTIES OF WATER SOURCES OF WATER Excellent Solvent Surface Water Cohesion 1/3 of the rainfall or precipitation High Specific Heat Capacity Expansion Groundwater Osmosis and Capillary Action CHEMISTRY OF WATER WATER POLLUTION a. Physical Parameters b. Physical Parameters c. Biological Parameters Color Total Solids composed of: Algae Odor Carbonates Fungi Turbidity Sulphates Viruses Taste Chlorides Protozoa Temperature Fluorides Bacteria E:ectrical Conductivity Nitrates Metal ions CHEMISTRY OF WATER Water Pollutants Substances which can induce physical, chemical, or biological changes to a body of water SURFACE WATER POLLUTION Point Sources Non-Point Sources Sources that emit pollutants which sources of water pollution that indirectly affect directly influences different bodies of bodies of water through environmental changes water example would be contaminated water that examples are domestic and industrial runs off from farms, mines, and construction wastes for these wastes are directly sites, affecting bodies of water dumped into bodies of water more difficult to deal with than point sources CHEMISTRY OF WATER SURFACE WATER POLLUTION Natural Sources Anthropogenic Sources an increase in the concentration of naturally pollution due to human activities or man- occurring substances made sources of water pollution Siltation occurs when continuous Domestic wastes, such as sewage and waste deforestation makes soil loose and run-off water, industrial wastes, and agricultural water during precipitation bring silt and dirt wastes are common example from mountain into bodies of water CHEMISTRY OF WATER GROUNDWATER POLLUTION Results from polluted water seeping into the ground and enters an aquifer Polluted water such as sewage, nitrogenous fertilizer, and toxic waste POLLUTANTS SOURCES OF POLLUTANTS EFFECTS AND SIGNIFICANCE 1. PATHOGENS SEWAGE, HUMAN AND DEPLETION OF DISSOLVED ANIMAL WASTES, NATURAL OXYGEN IN WATER (FOUL AND URBAN RUNOFF FROM ODOR), HEALTH EFFECTS LAND, INDUSTRIAL WASTE (OUTBREAKS OF WATER BORNE DISEASES) 2. ORGANIC POLLUTANTS AUTOMOBILE AND MACHINE DISRUPTION OF MARINE LIFE OIL AND WASTE, TANKER SPILLS, AESTHETIC DAMAGE GREASE OFFSHORE OIL LEAKAGE, TOXIC EFFECTS TO AQUATIC PESTICIDES AGRICULTURAL CHEMICALS, LIFE PLASTICS DETERGENTS INDUSTRIAL AND HOUSEHOLD WASTE GENETIC DEFECTS AND CANCER FISH KILLS CHEMISTRY EUTROPHICATION 3. INORGANC POLLUTANTS FERTILIZERS AGRICULTURAL RUNOFF ALGAL BLOOM AND EUTROPHICATION, NITRATES CAUSE METHEMOGLOBENEMIA OF WATER (PHOSPHATES & NITRATES) ACIDS & MINE DRAINAGE, INDUSTRIAL KILLS FRESH WATER ALKALIS WASTES, NATURAL AND ORGANISMS, UNFIT FOR URBAN RUNOFF DRINKING, IRRIGATION AND INDUSTRIAL USE 4. RADIOACTIVE NATURAL SOURCES, URANIUM CANCER AND GENETIC DEFECTS MATERIALS MINING AND PROCESSING, HOSPITALS, LABORATORIES USING RADIOISOTOPES 5. HEAT COOLING WATER FOR DECREASE SOLUBILITY OF INDUSTRIAL, NUCLEAR AND OXYGEN IN WATER, DISRUPTS THERMAL PLANTS AQUATIC ECOSYSTEMS 6. SEDIMENTS NATURAL EROSION, RUNOFF AFFECTS WATER QUALITY, FROM AGRICULTURAL LAND REDUCES FISH POPULATION AND CONSTRUCTION SITES CHEMISTRY OF WATER FACTORS THAT AFFECT WATER QUALITY 1. Temperature 2. Dissolved Oxygen 3. Alkalinity 4. Acidity 5. Hardness CHEMISTRY OF WATER TEMPERATURE Thermal Stratification Formation of distinct layers within non- flowing bodies of water due to temperature difference The two layers of a body of water do not mix, but they behave independently and have different chemical and biological properties CHEMISTRY OF WATER EPILIMNION – surface layer constantly exposed to solar radiation, resulting to higher temperature and lower density, making it float over the bottom THERMOCLINE - layer between the epilimnion and hypolimnion Heavy growth of algae due to exposure to atmosphere and sunlight Contains high levels of dissolved oxygen HYPOLIMNION – lowest layer Anaerobic due to consumption of oxygen by biodegradable organic material CHEMISTRY OF WATER Properties of Water that are affected by Changes in Water Temperature is temperature: caused by: 1. The amount of gas that can be dissolved 1. Air Temperature in the water. Cold water can dissolve 2. Thermal Pollution more oxygen than warm water. occurs when water in an entering 2. The rate of photosynthetic action of algae stream is warmer than the water and other aquatic plants. already present in a body of water 3. The metabolism of aquatic organisms Sources: nuclear power plants, which (respiration, digestion, etc.) discharge cooling water CHEMISTRY OF WATER DISSOLVED OXYGEN Needed by aquatic organisms for respiration and decomposition of organic matter Most fish kills result from low levels of dissolved oxygen ALKALINITY a measure of its capacity to neutralize acids, or a measure of buffer capacity (greatly used in waste water treatment) the alkalinity of waters can be attributed to the salts of weak acids Bicarbonates contribute the most to the alkalinity of water CHEMISTRY OF WATER ACIDITY CO2 Acidity is acidity due to carbon dioxide dissolved in bodies of water. This is the most common form of acidity in water. Acidic waters are of major concern because of their corrosive characteristics HARDNESS Divalent metallic cations cause the hardness of water principal hardness causing cations are calcium, magnesium, strontium, ferrous and manganous ions CHEMISTRY OF SOIL SOIL heterogeneous mixtures of air, water, inorganic and organic solids, and microorganisms (both plant and animal) Soil Chemistry is the branch of science that deals with the chemical composition, properties and reactions of soils Environmental Soil Chemistry is the study of chemical reactions between soils and environmentally important plant nutrients, radionuclides, metals, metalloids, and organic chemicals CHEMISTRY OF SOIL COMPOSITION OF SOIL Inorganic Components of Soil Peds are formed by the aggregation of sand, silt, and clay particles to form larger soil structure that result from the action of soil forming factors Profiles develop in the loose material on the earth’s surface and are composed of layers of varying texture, structure, color, bulk density and other properties. These layers are also of varying thickness Pedon is the smallest unit that can be considered “soil” and consists of all horizons extending from the soil surface to the underlying geologic strata. An area consisting of similar pedons is called a polypedon CHEMISTRY OF SOIL Inorganic Components of Soil The elements found in soils with the highest quantities are the following: Oxygen - most prevalent element. (47% of the Earth’s crust by weight and 90% by volume) Silicon Aluminum Iron Carbon Calcium Potassium Sodium Magnesium CHEMISTRY OF SOIL MINERAL a natural inorganic compound with definite physical, chemical and crystalline properties CHEMISTRY OF SOIL PRIMARY MINERAL a mineral that has not been chemically altered since its deposition from molten lava. Examples: quartz and feldspar, pyroxenes, micas, amphiboles and olivines primarily occur on sand and silt fractions of soils but may be found in slightly weathered clay-sized fractions CHEMISTRY OF SOIL SECONDARY MINERAL the result of the weathering of a primary mineral, either through alteration in the structure or from reprecipitation of the products of weathering (dissolution) of a primary mineral Examples aluminosilicate minerals such as kaolinite and montmorillonite, oxides such as gibbsite, goethite and birnessite, amorphous materials such as allophane, and in sulfur and carbonate materials. primarily found in the clay fraction of the soil but can also be found in the silt fraction CHEMISTRY OF SOIL COMPOSITION OF SOIL Organic Components of Soil Soil Organic Matter (SOM) also called humus, includes the total organic compounds in soils, excluding undecayed plant and animal tissues, their “partial decomposition” products, and soil biomass mixture of plant and animal residues in different stages of decomposition, substances synthesized microbiologically and/or chemically from the breakdown products, and the bodies of live and dead microorganisms and their decomposing remains CHEMISTRY OF SOIL Term Definition Organic residues Undecayed plant and animal tissues and their partially decomposed products Soil biomass Organic matter present as live microbial tissue A series of relatively high-molecular-weight, brown-to-black-colored substances formed Humic substances by secondary synthesis reactions. Compounds belonging to known classes of biochemistry, such as amino acids, Nonhumic substances carbohydrates, fats, waxes, resins, and organic acids Humin The alkali insoluble fraction of soil organic matter The dark-colored organic material that can be extracted from soil by various reagents Humic acid and is insoluble in dilute acid Fulvic acid The colored material that remains in solution after removal of humic acid by acidification Hymatomelanic acid Alcohol soluble portion of humic acid CHEMISTRY OF SOIL Property Remarks Effect on soil Color The typical dark color of many soils is caused by organic matter May facilitate warming Water retention Organic matter can hold up to 20 times in weight in water Help prevent drying and drinkingMay significantly improve the moisture-retaining properties of sandy soils Combination with clay Cements soil particles into structural units called aggregates Permits exchange of gases. Stabilizes structure. Increases materials permeability. Chelation Forms stable complexes with copper, manganese, zinc and other May enhance the availability of micronutrients to higher plants polyvalent cations Solubility in water Insolubility of organic matter is because of its association with clay. Little organic matter lost by leaching. Also, salts of divalent and trivalent cations with organic matter are insoluble. Isolated organic matter is partly soluble in water. Buffer action Organic matter exhibits buffering in slightly acid, neutral, and alkaline Helps to maintain a uniform reaction in the soil. ranges. Cation exchange Total acidities of isolated fractions of humus range from 300 to 1400 May increase the CEC** of the soil. From 20 to 70% of the CEC cmol/kg of many soils is due to organic matter. Mineralization Decomposition of organic matter yields carbon dioxide and A source of nutrient elements for plant growth. ammonium, nitrate, phosphate, and sulfate ions Combines with organic Affects bioactivity, persistence and biodegradability of pesticides and Modifies application rate of pesticides for effective control. Chemicals other organic chemicals **CEC (Cation Exchange Capacity) of soil humus is the maximum number of moles of proton charge dissociable from unit mass of solid-phase humus under given conditions of temperature, pressure, and aqueous solution composition, including the humus concentration. CHEMISTRY OF SOIL SOIL DECONTAMINATION In Situ Techniques Non-In Situ Techniques a. Volatilization a. Land Treatment b. Biodegradation b. Thermal Treatment c. Phytoremediation c. Asphalt Incorporation d. Leaching d. Solidification/Stabilization e. Vitrification Chemical Safety Image source: https://i.ytimg.com/vi/1oHhhAlEsJc/maxresdefault.jpg Outline Introduction: Case Studies Hazard Communication Material Safety Data Sheet (MSDS) Globally Harmonized System (GHS) of Chemical Labeling Chemical Exposure Personal Protective Equipment (PPE) Chemical Storage Chemical Spill Response Waste Disposal General Safety Rules Introduction: A Case Study Small tubes of petroleum ether (PE) were stored in an ordinary domestic freezer The tubes were not sealed well, and the PE https://www.gasdetectortubes.com/kwikdraw/eu/44- tubes/tubes-detail-info/228-petroleum-ether-short-term- evaporated to a concentration exceeding gasdetector-tubes the lower explosive limit (about 1.0%) Flash point of PE is −50℃ A spark from an internal component of the freezer caused the PE to ignite, resulting in $500,000 in damage University of Missouri 2010 Explosion https://shoham.net.technion.ac.il/fa cilities/anaerobic-hood/ Lab personnel ignored a “warning system” designed to tell researchers when too much hydrogen enters the anaerobic hood and becomes flammable An explosion resulted when gas Anaerobic hood - an oxygen-free come into contact with an chamber used for ignition source working with 4 people were injured, one of bacteria that can’t https://encrypted- tbn0.gstatic.com/images?q=tbn%3AANd them critically survive in oxygen 9GcQHuMhrkNk5Vtv7WKQCkDt3fMjBEn- CMwj1qg&usqp=CAU Hazard Communication Allows workers to know the hazards and identities of the chemicals they are exposed to while working Describes measures workers can take to protect themselves Hazards are communicated by: Labels Material Safety Data Sheet (MSDS) Education and Training https://www.optimumsafetymanagement.com/blog/osha- safety-training-new-hazard-communication-standard/ Material Safety Data Sheet (MSDS) A document prepared by the chemical manufacturer that describes: Physical and chemical properties Physical and health hazards Routes of exposure Precautions for safe handling and use Emergency and first-aid procedures Control measures https://www.slideshare.net/VikashMishra53/material-safety-data-sheetor- safety-data-sheet Sample MSDS https://www.texasgateway.org/resource/material-safety-data-sheets Globally Harmonized System (GHS) of Classification and Labelling of Chemicals Defines and classifies the hazards of chemical products Communicates health and safety information on labels and safety data sheets A set of rules for classifying hazards, and the same format and content for labels and safety data sheets (SDS) adopted and used around the world A 'non-binding' system of hazard communication Developed by an international team of hazard communication experts https://www.pinterest.ph/pin/462322717972720733/ Toxicity and Risk Toxicity The ability of a chemical to cause harm, i.e. “hazard” in general safety terms Risk Likelihood of a material to cause harm under conditions of use With proper handling, even highly toxic chemicals can be used safely Less toxic chemicals can be extremely hazardous if handled improperly Routes Chemical Inhalation – breathing (powders, fumes) Exposure Ingestion – entry through mouth Injection – through skin by foreign body Absorption – skin or mucus membranes Classifications (based on duration) Acute exposure (short term) eye irritation, nausea, dizziness, skin rash, burns, headache, etc. Chronic exposure (long term) long-term illnesses Protection Measures: Hierarchy of Risk Controls 1. Eliminate the hazard (ex. not using shelves) 2. Substitute other materials, processes or equipment (ex. toluene instead of benzene) 3. Have engineering controls (ex. fume hoods, engineered sharps, eye wash) 4. Use systems that increase awareness of potential hazards 5. Have administrative controls (training and procedures, instructions, scheduling) 6. Use personal protective equipment (PPE) – gloves (proper size and material), lab coats, eye protection, safety shoes, respirators, face shields, masks Personal Protective Equipment (PPE) worn to minimize exposure to hazards that cause serious workplace injuries and illnesses, which may result from contact with chemical, radiological, physical, electrical, mechanical, or other workplace hazards https://health.ucdavis.edu/medresearch/safety/Documents/Doc uments2020/4.3_Poster_for_PPE_and_Lab_Attire.pdf https://safety.nmsu.edu/lab-safety/chem-safety/lab-personnel-protective-equipment/ The Sheharbano “Sheri” Sangji Case (UCLA, 2008) The first criminal case resulting from an academic laboratory accident Sangji (23 y.o. R.A.) was working with a bottle https://losangeles.cbslocal.com/2014/06/20/sett lement-reached-in-fire-that-killed-ucla-chemist/ of t-butyl lithium dissolved in pentane While using a syringe to withdraw a quantity of the reagent, she accidentally pulled the plunger all the way out, introducing air and creating a flash fire Sangji was wearing nitrile gloves, safety glasses rather than goggles, a synthetic sweater, and no lab coat The fire ignited the gloves and sweater, and 40% of her body was burnt. She died 18 days later. https://ehs.unl.edu/safety- posters/chemical-safety-posters Glove Material Compatibility Generally Recommended Not Recommended Material for for Aromatics, hydrocarbons, Alcohols, caustics, Natural Rubber (NR) many solvents (especially ketones, many acids chlorinated and aromatic) Many acids, alcohols, Ketones, chlorinated Nitrile Buna Rubber (NBR) caustics, hydrocarbons hydrocarbons, strong acids Organic acids, caustics, Aromatic and chlorinated Neoprene alcohols, petroleum, solvents solvents, ketones The Karen Wetterhahn Case (Dartmouth College, 1997) Chemistry professor specializing in toxic metal exposure A few drops of dimethylmercury accidentally spilled on her hands, which were protected only by latex gloves Tests showed that DMM can rapidly permeate latex gloves and enter the skin within 15 seconds Single exposure to DMM raised Prof. Wetterhahn’s blood mercury level to 80 times the toxic threshold Delayed neurotoxic effects caused her to be hospitalized after 5 months, and died 10 months later at the age of 48 https://images.de nios.co.uk/produc ts_solutions/prod ucts/Storing_haza Chemical Storage rdous_materials/b egehbare- gefahrstofflager- 1a05.jpg Safe chemical handling requires routine inspections of chemical storage areas and maintenance of stringent inventory control. The inherent hazards of chemicals can be reduced by minimizing the quantity of chemicals on hand. However, when chemicals must be used, proper storage and handling can reduce or eliminate associated risks. All chemical storage areas and cabinets should be inspected at least annually and any unwanted or expired chemicals should be removed. Typical storage considerations: temperature, ignition control, ventilation, segregation and identification Proper segregation (physical barrier and/or distance) is necessary to prevent incompatible materials from inadvertently coming into contact Chemical Storage Guide White = compatible Red = incompatible https://its environm entalservi ces.com/r Chemical Spill Response esources/ chemical- spill- emergenc y/ WARNING: DO NOT RESPOND BEYOND YOUR TRAINING LEVEL! Stop. Think. IS IT A MAJOR SPILL? NO YES Remove contaminated Rescue clothing Avoid the chemical Use proper PPE Find the MSDS Contain spill Telephone for help Notify workers in the area Seek MSDS for advice Notify supervisor/security https:// www.pu rdue.ed u/uns/i Chemical Fire mages/2 018/pt- chem- show.jpg Stop. Think. CAN I EXTINGUISH THIS FIRE? YES NO Extinguish open flames Evacuate area Turn off gas/electricity immediately Notify workers in the area Call emergency number Ventilate work area Notify supervisor/security Hazardous Waste Minimize waste in the first place Do not pour chemical waste down the drain Know your chemical classification Use flame resistant container with label Don’t leave funnel on top of waste container Use proper mercury disposal (ex. broken thermometer) Call for pick up https://www.researchgate.net/profile/Sherly_Antony/publication/337704548/figure/fig2/ AS:896459574616067@1590744026580/Symbols-characteristics-of-Hazardous-Waste.png General Safety Rules: DOs and DON’Ts DO DON’T Store chemicals in their original Buy chemicals you do not need containers Eat, drink, smoke, chew gum, or Wear appropriate safety gear and apply cosmetics near chemicals work in a controlled environment Mouth pipette Dispose chemicals properly Use unlabeled containers Use care in handling contaminated glassware or needles Know chemical properties and toxicity https://guidancec orner.com/labora tory-safety-rules/ References Callaghan, J. (2012). WHMIS and OSHA labels. Canadian Center for Occupational Health and Safety. https://www.slideshare.net/CCOHS/whmis-and-osha-labels Sampson, S. (2014). Chemical Safety in the workplace. Safety Services Nova Scotia. https://www.slideshare.net/AWARE-NS/chemical-safety- in-the-workplace https://auspicesafety.com/2020/01/28/whmis-2015-and-ghs-what- you-need-to-know/ https://www.ccohs.ca/oshanswers/chemicals/ghs.html