Bioenergetics and Integrated Metabolism (BIOC202) PDF
Document Details
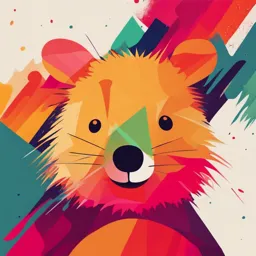
Uploaded by FriendlyTrust
University of KwaZulu-Natal - Westville
Tags
Summary
This document presents a collection of lecture notes about bioenergetics and integrated metabolism (BIOC202), encompassing various metabolic pathways. It discusses glucose metabolism, the role of red blood cells, and biochemical processes.
Full Transcript
BIOENERGETICS AND INTEGRATED METABOLISM (BIOC202) How do other monossacharides enter the glycolytic pathway? GALACTOSEMIA – clinical implication Galactose enter glycolysis as per below reaction Deficiency of Galactokinase or Galactose-1-phosphat...
BIOENERGETICS AND INTEGRATED METABOLISM (BIOC202) How do other monossacharides enter the glycolytic pathway? GALACTOSEMIA – clinical implication Galactose enter glycolysis as per below reaction Deficiency of Galactokinase or Galactose-1-phosphate uridyltransferase GLYCOLYSIS GALACTOSEMIA A deficiency of galactose-1-phosphate uridyltransferase manifests itself in the newborn as intolerance of breast or cow’s milk which contains lactose. Galactose-1-phosphate rises in the blood and causes damage to the liver, kidneys, brain, intestine and spleen. Lactose Intolerance - implications maltase Maltose + H2O D-Glucose + D-Glucose lactase Lactose + H2O D-Galactose + D-Glucose Sucrose + H2O sucrase D-Fructose + D-Glucose Galactose Glucose-6-phosphate Mannose Fructose-6-phosphate Fructose (muscle) Fructose-6-phosphate Fructose (liver) Glyceraldehyde-3-phosphate GALACTOSE METABOLISM AND GALACTOSEMIA When galactose accumulates, the reaction catalyzed by aldose reductase becomes very active: Aldose reductase which has a high Km for aldoses Km is the Michaelis constant and represents the substrate concentration at which the reaction rate is half the maximum rate of the reaction i.e. high levels of aldoses (galactose) are required for the enzyme to become active therefore aldose reductase is not normally active The accumulation of galactitol and depletion of NADPH in the lens of the eye, leads to damage of the tissue and to cataract. FRUCTOSE METABOLISM Fructose enters the glycolytic pathway by one of two routes. In muscle: Hexokinase, which phosphorylates various monosaccharides, can also act on fructose to produce fructose 6-phosphate. But this is not the major pathway. Hexokinase has low affinity (high Km) for fructose. As affinity of the enzyme hexokinase for fructose is very low, hence this is a minor pathway FRUCTOSE METABOLISM In the liver, we have a major pathway for phosphorylation of fructose – catalysed by the enzyme fructokinase ( also been identified in kidney and intestine) Fructose is mostly phosphorylated by fructokinase to fructose 1-phosphate – this enzyme phosphorylates fructose only and will not phosphorylate glucose. Fructose 1-phosphate is cleaved to glyceraldehyde and dihydroxyacetone phosphate (DHAP) by aldolase B. FRUCTOSE INTOLERANCE A metabolic defect can occur which involves fructose-1-phosphate aldolase B and leads to fructose (sucrose) intolerance in the newborn. Fructose-1-phosphate accumulates in the liver and damages it, resulting in jaundice. High concentrations of fructose-1-phosphate also inhibit: aldolase of the glycolytic pathway (reaction 4) thus inhibiting ATP generation & glycogen phosphorylase leading to hypoglycemia. SUCROSE INTOLERANCE BIOENERGETICS AND INTEGRATED METABOLISM (BIOC202) GLYCOLYSIS AND RED BLOOD CELLS (RBC) Glucose metabolism role in RBC functions: 1) RBCs rely solely on glycolysis to generate adenosine triphosphate (ATP); 2) approximately 25% of glucose in RBCs is used to produce the RBC specific metabolite 2,3-bisphosphoglycerate (2,3-BPG) for haemoglobin O2 affinity modulation; 3) RBCs depend on the oxidative branch of the pentose phosphate pathway (PPP) to generate reducing equivalents (NADPH) to preserve glutathione homeostasis and counteract oxidative stress.” (Sun et al., 2017) IMPORTANCE OF GLYCOLYSIS IN RBCs 2- O OPO 3 C Bisphosphoglycerate mutase H C OH 2- CH2 OPO3 1,3-Bisphosphoglycerate (1,3-BPG) 2ADP - COO 7 Phosphoglycerate 2- kinase H C OPO3 ( PGK) 2ATP 2- CH2 OPO3 2,3-Bisphosphoglycerate (2,3-BPG) 3-Phosphoglycerate (3PG) - COO H2O 2,3-Bisphosphoglycerate H C OH p hosphatase 2- CH2 OPO3 Pi IMPORTANCE OF GLYCOLYSIS Production of 2,3-bisphosphoglycerate (2,3-BPG), an allosteric effector that promotes the release of oxygen from hemoglobin. 2,3-bisphosphoglycerate interacts with the subunits of hemoglobin thereby decreasing its affinity for oxygen. Red blood cells contain the enzyme bisphosphoglycerate mutase that catalyses the transfer of a phosphoryl group from C-1 to C-2 of 1,3- bisphosphoglycerate, to form 2,3-BPG. IMPORTANCE OF GLYCOLYSIS The enzyme 2,3-bisphosphoglycerate phosphatase then converts 2,3-BPG to 3-phosphoglycerate which can re-enter the glycolytic pathway and be converted to pyruvate. These steps bypass step 7 of the glycolytic pathway during which ATP is generated. Thus, in exchange for decreased ATP production, there is a regulated supply of 2,3-BPG that is crucial for release of O2 from hemoglobin. ARESENATE POISONING Arsenic and phosphorous is in Group V of the periodic table Arsenate is an analogue of inorganic phosphate and can replace a phosphate group in 1,3-bisphospholycerate to give arseno-3- phosphoglycerate Arseno-3-phosphoglycerate can be rapidly hydrolyzed in water to produce 3- phosphoglycerate Step 7 of glycolysis is by-passed – no net yield of ATP ARSENATE POISONING O 2- O OPO3 O O As O C C O H C OH H C OH 2- 2- CH2 OPO3 CH2 OPO3 1,3-Bisphosphoglycerate (1,3-BPG) 1-Arseno-3-phosphoglycerate 2ADP H 2O 7 Phosphoglycerate non-enzymatic kinase 2ATP (PGK) AsO4 3- 3-Phosphoglycerate (3PG) 3-Phosphoglycerate (3PG) - - COOH COOH H C OH H C OH 2- 2- CH2 OPO3 CH2 OPO3 REGULATION OF GLYCOLYSIS First step of regulation - glucose transport into the cells via glucose transporters Intestinal and kidney cells have Na+-dependent co-transporters called SGLT1 – glucose moves in by passive transport via facilitated diffusion The GLUT family makes up the other hexose transporters: GLUT 1 & GLUT 3 – present in nearly all mammalian cells, continually transports glucose at a constant rate GLUT 2 – present in liver cells GLUT 4 – present in skeletal muscle cells and adipocytes. Insulin promotes rapid uptake of glucose by increasing the number of GLUT 4 receptors on the cell membrane GLUT 5 – transports glucose in the small intestine GLUT 7 – transports glucose-6-phosphate from the cytosol to the ER. REGULATION OF GLYCOLYSIS Three enzymes i. Hexokinase – inhibited by glucose-6- phosphate ii. Phosphofructokinase (PFK) - inhibited by [ATP] and citrate - activated by [AMP] and fructose-2.6- bisphosphate. (When present in high amount, Fructose-6- phosphate can be converted to fructose-2,6- bisphosphate) iii. Pyruvate kinase - inhibited by [ATP] - activated by fructose-2,6-bisphosphate REGULATION OF GLYCOLYSIS i. Hexokinase – inhibited by glucose-6-phosphate REGULATION OF GLYCOLYSIS ii. Phosphofructokinase (PFK) - inhibited by [ATP] and citrate - activated by [AMP] and fructose-2.6- bisphosphate. When present in high amount, fructose-6- phosphate can be convertedto fructose-2,6- bisphosphate) ii. Phosphofructokinase (PFK) (contd) Excess Fructose-6-phosphate is converted to fructose-2,6-bisphosphate using the enzyme Phosphofructokinase-2 (PFK-2) Thus presence of fructose-2,6-bisphosphate indicates that lots of fructose-6- phosphate is present and thus glycolysis must occur FBPase-2 = Fructose-2,6-bisphosphatase-2 REGULATION OF GLYCOLYSIS iii. Pyruvate kinase - inhibited by [ATP] - activated by fructose-2,6-bisphosphate BIOENERGETICS AND INTEGRATED METABOLISM (BIOC202) Ms. SR Mokhosi (Module Coordinator & Lecturer: [email protected]) AIM OF MODULE: To introduce students to integrated biochemical pathways. CONTENT TOPIC Carbohydrate Metabolism Lipid Metabolism Amino Acid Metabolism Nucleotide Metabolism & 1-Carbon Fragments TEXTBOOK Author(s) Title Year ISBN Publisher Moran LA, Horton Principles of Biochemistry, RA, Scrimgeour G, 2011 978-0321795793 Pearson 5th edition Perry M & Rawn D Voet D, Voet JG and Principles of Biochemistry: John Wiley & 2008 978-0470233962 Pratt CW Life at the Molecular Level Sons INTRODUCTION In this module, you will learn about the pathways of: Carbohydrates, Amino acids, Lipids, Nucleic acid, and One carbon fragment metabolism. Even though the pathways are discussed individually, metabolism within an organism is an integrated process. OVERVIEW OF CARBOHYDRATE METABOLISM : Dietary carbs Glycolysis Transition step (pyruvate → acetyl CoA) TCA (Krebs) cycle Anaplerotic reactions The Pentose Phosphate Pathway Glycogenesis Glycogenolysis Gluconeogenesis OVERVIEW OF CARBOHYDRATE METABOLISM Dietary carbs Glycolysis Transition step (pyruvate → acetyl CoA) TCA (Krebs) cycle Anaplerotic reactions The Pentose phosphate pathway Glycogenesis Glycogenolysis Gluconeogenesis HOW ARE THE PATHWAYS ELUCIDATED? Pathways are elucidated by complex processes and may involve use of chemical speculation, inhibitors, radiochemical tracers, gene expression based studies using bacterial mutants, knock-down animals, gene silencers, etc. Techniques used may include various forms of chromatography: TLC – thin layer chromatography, HPLC – high-performance liquid chromatography, NMR– nuclear magnetic resonance, MS – mass spectrometry, X-ray crystallography WHERE ARE THE METABOLIC PATHWAYS LOCATED? Metabolic pathways are located in different cell compartments or organelles thus each cell organelle is defined by specific metabolic activities that take place within it. This compartmentalization of metabolic activities can assist in the regulation of cellular metabolism. WHERE ARE THE METABOLIC PATHWAYS LOCATED? Table 1: Metabolic functions of Eukaryotic Organelles BIOENERGETICS AND INTEGRATED METABOLISM (BIOC202) Lecture aims Discuss the transition step (conversion of pyruvate to acetyl CoA) TRANSITION STEP (CONVERSION OF PYRUVATE TO ACETYL COA) Occurs in the mitochondria Links Glycolysis and the TCA cycle TRANSITION STEP (CONVERSION OF PYRUVATE TO ACETYL COA) Catalysed by the pyruvate dehydrogenase (PDH) complex that consists of the following three enzymes: Pyruvate dehydrogenase Dihidrolipoyl transacetylase Dihydrolipoyl dehydrogenase CONVERSION OF PYRUVATE TO ACETYL COA The following co-factors are required: o Mg2+, o Thiamine pyrophosphate, o FAD, o Lipoic acid, o CoASH, and o NAD+ The products of this reaction from 1 pyruvate are: i. 1x CO2 ii. 1x NADH iii. 1x Acetyl CoA CONVERSION OF PYRUVATE TO ACETYL COA PDH has lipoamide sulphydryl groups to which arsenic and organic arsenicals can bind and thus these compounds can inhibit PDH The enzyme α-ketoglutarate dehydrogenase which acts in the TCA cycle can also be inhibited by arsenic since it resembles the PDH complex REGULATION OF THE PDH COMPLEX Regulated by phosphorylation: o Phosphorylated = active o Dephosphorylated = inactive REGULATION OF THE PDH COMPLEX The three ezymes can also be individually regulated: i. Pyruvate dehydrogenase inhibited by high [ATP] and [GTP]; activated by high [AMP], [Ca2+] and [pyruvate] ii. Dihidrolipoyl transacetylase inhibited by high [acetyl CoA]; activated by high [CoA-SH] iii. Dihydrolipoyl dehydrogenase inhibited by high [NADH]; activated by high [NAD+] TRICARBOXYLIC ACID (TCA) CYCLE Also referred to as the Citric Acid or Kreb’s Cycle Occurs in the mitochondria The high energy thiol ester, Acetyl CoA, enters the TCA cycle where it undergoes further oxidation TRICARBOXYLIC ACID (TCA) CYCLE TO NOTE: The energy for the synthesis of citrate in reaction 1 comes from hydrolysis of the thiol ester In reaction 2, a symmetrical compound (achiral) is converted into an asymmetrical (chiral) compound GTP is equivalent to ATP since it can phosphorylate ADP to yield ATP and GDP in a reaction catalysed by nucleotide diphosphate kinase By reaction 4, all six carbons from glucose have been converted to CO2 TRICARBOXYLIC ACID (TCA) CYCLE The products from 1 glucose and 2 resulting acetyl CoA molecule are : i. 4 x CO2 ii. 6 x NADH iii. 2 x FADH2 iv. 2 x GTP The TCA cycle is amphibolic i.e. it is involved in catabolic and anabolic reactions Most catabolic pathways end with TCA cycle intermediates while most anabolic pathways start from the cycle’s intermediates REGULATION OF THE TCA CYCLE 1. Isocitrate dehydrogenase inhibited by high [NADH]; activated by high [Ca2+] and [ADP] 2. α-Ketoglutarate dehydrogenase inhibited by high [NADH] and [succinyl CoA]; activated by high [Ca2+] Acetyl CoA (2x 2C) Glycolysis: H 2O Glucose (6C) → 2 Pyruvates (2x 3C) CoASH NADH + H+ Oxaloacetate Citrate 1 Citrate 8 synthase NAD+ M alate dehydrogenase - Oxidation L-Malate 2 Transition Step: 7 Aconitase 2 Pyruvates H 2O Fumarase - Hydration Fumarate 2x Acetyl CoA (2x 2C) TCA Cycle Isocitrate 2x CO2 (2x 1C) FADH2 6 2x NADH Succinate 3 I socitrate NAD+ dehydrogenase - dehydrogenase Oxidation FAD Oxidative decarboxylation Succinate NADH + H+ CoASH 4 α -Ketoglutarate GTP 5 dehydrogenase - Succinyl-CoA synthetase Oxidative (2x 1C) decarboxylation CO2 GDP + Pi CO2 CoASH (2x 1C) Succinyl CoA α-Ketoglutarate NADH + H+ NAD+ BIOENERGETICS AND INTEGRATED METABOLISM (BIOC202) THE PENTOSE PHOSPHATE PATHWAY All reactions are cytosolic. This pathway has four main functions: i. Generation of NADPH which is involved in reductive biosynthetic pathways. ii. Source of pentoses from hexoses, in particular ribose-5-phosphate which is required in large amounts in nucleic acid and co-enzyme synthesis. iii. Allows for the oxidation of pentose carbon by channeling it into glycolysis. iv. It is a source of erythrose which is required for the biosynthesis of aromatic amino acids. THE PENTOSE PHOSPHATE PATHWAY This pathway has three distinct phases: 1) Oxidation, 2) Isomerization and 3) Sugar rearrangement The products of the pentose phosphate pathway when 3x glucose-6-phosphate are used are: 1) 3 x CO2 2) 6 x NADPH 3) 2x Fructose-6-phosphate 4) Glyceraldehyde This pathway is active in tissues that synthesis fatty acids or sterols since large amounts of NADPH are required. There is little activity of the pentose phosphate pathway in muscle and brain. THE PENTOSE PHOSPHATE PATHWAY The path of carbon in the pentose phosphate pathway. In the oxidative stage, three molecules of a six-carbon (total of 18 C) compound are converted to three molecules of a five-carbon sugar (ribulose 5-phosphate) (total of 15 C), CO2 is released (total of 3C). In the nonoxidative stage, three molecules of five-carbon sugars (total of 15 C) are interconverted to produce two molecules of a six-carbon sugar (fructose 6- phosphate) (total of 12 C) and one molecule of a three-carbon compound (glyceraldehyde 3-phosphate). 3x = 18C THE IMPORTANCE OF NADPH One of the functions of NADPH produced by PPP is to keep –SH groups in proteins in a reduced state. Another function is to reduce oxidized glutathione. Glutathione (λ-L-glutamyl-L-cysteinylglycine) eliminates Reactive Oxygen Species (ROS) such as H2O2, by reducing them and in the process becomes oxidized itself. If left unchecked, the peroxides cause premature cell lysis as they cleave C- C bonds in phospholipid tails of membranes and also damage hemoglobin irreversibly. IMPORTANCE OF NADPH 6-phospho gluconolactone Oxidised Reduced form Glutathione form Eliminates ROS (e.g. H2O2) By Reducing it Damage caused by peroxides Clinical Implication: Glucose-6-phosphate Dehydrogenase Deficiency In patients taking the anti-malaria drug, primaquine, hemolytic anemia can result if they have deficiency of G-6-PDH. This is because the drug promotes peroxide formation. NADPH is insufficient to reduce Glutathione. Glutathione (reduced form) levels are too low to reduce all the peroxide produced and this results in damage to the red blood cell membrane. GLYCOGEN SYNTHESIS AND BREAKDOWN Glycogen is a storage carbohydrate with a relative mass of x106 Daltons Storage sites: 1. Liver, approximately 6% by weight after a high carbohydrate diet 2. In muscle, rarely above 1% Functions: Liver glycogen maintains blood glucose level within normal limits. Muscle glycogen is the source of hexose units (glucose) for glycolysis in this tissue. GLYCOGEN SYNTHESIS – aka Glycogenesis The first three reactions of glycogenesis involves the conversion of glucose to UDP-glucose. GLYCOGENESIS Glycogen synthase then adds glucose residues from UDP-glucose to a primer of seven glucose residues. This seven glucose residue molecule is synthesized by glycogenin and linkages between glucose units are α(1→4). A minimum of six residues from a chain that is at least 11 residues in length are transferred to a neighbouring chain to form an α(1→6) branch in a reaction catalyzed by amylo-(1-4→1-6)transglycosylase (branching enzyme). The new branch must be at least four residues from other branch points. Glucose 1 Hexokinase OR Glucokinase- Phosphorylation Glucose-6-phosphate (G6P) UTP 2 Phosphoglucomutase - Isomerization I norganic pyrophosphatase PPi 2 Pi Glucose-1-phosphate (G1P) 3 UDP-Glucose pyrophosphorylase - Phosphoanhydride exchange UDP-Glucose The first three reactions of glycogenesis Addition of glucose residues by glycogen synthase. The reaction involves a glucosyl oxonium ion intermediate. GLYCOGENOLYSIS Glycogen breakdown involves two main enzymes: 1) Phosphorylase – breaks α(1→4) linkages and removes outer chains until four residues are left on the α(1→6) chain chain. 2) α(1→4)→ α(1→4)-glucantransferase – transfers three of the four residues on the α(1→6) chain, exposing the α(1→6) residue which is then cleaved by amylo-1,6- glucosidase. Thereafter, G-1-P is converted to G-6-P by phosphoglucomutase which in turn is converted to glucose by glucose phosphatase. GLYCOGENOLYSIS Phosphorylase (Cleaves α(1-6) glycosidic bond) BIOENERGETICS AND INTEGRATED METABOLISM (BIOC202) GLUCONEOGENESIS Gluconeogenesis is a pathway for the synthesis of glucose from precursors such as pyruvate, lactate and amino acids. It is a reversal of glycolysis, except for reactions 1, 3 and 10 which involve large free energy changes and are not directly reversible. These reactions are bypassed. 1) Bypass of pyruvate kinase reaction 2) Bypass of phosphofructokinase reaction 3) Bypass of hexokinase reaction 1. Bypass of pyruvate kinase reaction: First, pyruvate is carboxylated to oxaloacetate. This occurs inside the mitochondrion, is catalysed by pyruvate carboxylase (PC) and requires biotin as CO2 carrier. Eating raw eggs may lead to biotin deficiency since they contain avidin, a protein that binds to biotin very strongly and hence prevents its intestinal absorption. Thereafter, oxaloacetate is converted to phosphoenolpyruvate by phosphoenolpyruvate carboxykinase (PEPCK) in a reaction that requires GTP. 2. Bypass of phosphofructokinase reaction Fructose-1,6-bisphosphate is converted to fructose-6-phosphate by fructose bisphosphatase (FBPase). 3. Bypass of hexokinase reaction: Glucose-6-phosphate is converted to glucose by glucose-6-phosphatase. Glucose-6-phosphatase is unique to the liver and kidney hence these are the only gluconeogenic tissues. Regulation of Gluconeogenesis 1. During low blood glucose, increased glucagon secretion leads to increased enzyme phosphorylation that results in inactivation of phosphofructokinase-2 (PFK-2) and activation of fructose-2,6-bisphosphatase-2 (FBase-2). Note that these two enzymes are different forms of the same enzyme molecule. The net result of their actions is reduced concentration of fructose-2,6-bisphosphate. i) PFK (the glycolytic enzyme) is thus inhibited ii) Fructose-2,6-bisphosphatase (FBPase, the enzyme that bypasses PFK during gluconeogenesis) is activated due to low fructose-2,6-bisphosphate. iii) Glycolysis slows down while gluconeogenesis is accelerated. Note that in muscle which is a non-gluconeogenic tissue, fructose-2,6-bisphosphate though present, is under a different control mechanism. The skeletal muscle enzyme does not even have a phosphorylation site hence it is not under cAMP-dependent phosphorylation. Low blood [glucose] cAMP activates an enzyme called protein kinase A Increased glucagon secretion Protein kinase A phosphorylates enzymes involved in glycolysis, gluconeogenesis, glycogenesis and glycogenolysis Increased [cAMP] Increased enzyme phosphorylation activation of FBPase-2 and inactivation of PFK-2 Decreased [F2,6P] Inhibition of PFK and activation of FBPase Increased gluconeogenesis Regulation of gluconeogenesis in the liver in response to low blood glucose levels Regulation of Gluconeogenesis 1. Increased phosphorylation also results in: i) Activation of phosphorylase which breaks down glycogen, inactivation of glycogen synthase. These events increase blood glucose. 2. During high blood glucose i) Increased insulin secretion leads to enzyme dephosphorylation ii) Activation of phosphofructokinase-2 (PFK-2); inactivation of fructose-2,6-bisphosphatase- 2(FBase-2) iii) Fructose-2,6-bisP activates PFK-1 - Glycolysis is Activated, and Gluconeogenesis is inhibited Table 2: Regulators of Gluconeogenic Enzyme Activity Enzyme Allosteric Allosteric Enzyme Protein inhibitors activators phosphorylation synthesis PFK ATP, Citrate AMP, F2,6P FBPase AMP, F2,6P PK Alanine, ATP, F1,6P, AMP Inactivates Acetyl CoA PC Acetyl CoA PEPCK Stimulated by glucagon PFK-2 Citrate AMP, F6P, Pi Inactivates FBPase-2 F6P Glycerol-3-P Activates CONTROL OF GLYCOGENESIS AND GLYCOGENOLYSIS To prevent wastage of energy resources, glycogen synthesis and breakdown should be reciprocally regulated. Reciprocal regulation occurs through phosphorylation and dephosphorylation of key enzymes. The phosphorylation of glycogen phosphorylase and glycogen synthase is under hormonal regulation through cAMP dependent kinases. Hormones like glucagon and adrenalin promote phosphorylation of these enzymes whereas insulin has the opposite effect. Dephosphorylation can occur by specific phosphatases. CONTROL OF GLYCOGENESIS AND GLYCOGENOLYSIS Phosphorylation: - activates glycogen phosphorylase (promotes glycogen breakdown) - inactivates glycogen synthase (stops glycogen synthesis) Dephosphorylation - inactivates glycogen phosphorylase - activates glycogen synthase Thus, glycogenesis and glycogenolysis do not occur together EFFECTS OF GLUCAGON ON GLYCOGEN METABOLISM GLUCAGON Adenylyl cyclase Glucagon receptor ATP cAMP + PPi cAMP cAMP PKA PKA inactive active ATP ADP P Phosphorylase Phosphorylase kinase kinase GLYCOGENESIS INHIBITED ATP ADP ATP ADP Glycogen (n+1) PP P Glycogen Glycogen Glycogen Glycogen phosphorylase b phosphorylase a synthase a synthase b G1P Glycogen (n) UDP-glucose Pi GLYCOGENOLYSIS Pi ACTIVATED EFFECT OF INSULIN ON GLYCOGEN METABOLISM GLYCOGENOLYSIS GLYCOGENESIS INHIBITED ACTIVATED ATP ADP ATP ADP P P Glycogen (n+1) Glycogen Glycogen Glycogen Glycogen phosphorylase b phosphorylase a synthase a synthase b G1P Glycogen ( n ) UDP-glucose Pi Pi Phosphoprotein INSULIN phosphatase-1 Hormonal regulation of glycogen metabolism BIOENERGETICS AND INTEGRATED METABOLISM (BIOC202) The energy released from glucose oxidation is captured in the form of NADH and FADH2 They can then be oxidized by the electron transport chain through a series of redox reactions ending with O2 as the final acceptor of their electrons. This results in release of large quantities of energy that is harnessed for the synthesis of ATP in a process referred to as oxidative phosphorylation ELECTRON TRANSPORT CHAIN (ETC) An electron transport chain is a series of protein complexes and other molecules that transfer electrons from electron donors to electron acceptors via redox reactions (both reduction and oxidation occurring simultaneously) There is coupling of this electron transfer with the transfer of protons (H+ ions) across a membrane. The electrons that transferred from NADH and FADH2 Many of the enzymes in the electron transport chain are membrane-bound. ELECTRON TRANSPORT CHAIN Complexes of the ETC are arranged in order of decreasing electronegativity of standard redox potentials When NADH is the electron donor, there are three points in the ETC where there is large enough change in standard redox potential and sufficient energy to drive the synthesis of an ATP molecule NADH → CoQ, CoQ → cytc, and cytc → O2 When FADH2 is the donor, there are only two points, which are the latter two CoQ → cytc, and cytc → O2 Intermembrane space of mitochondria H+ ATP Protein H+ H+ synthase complex H+ of electron Cyt c carriers IV Q I III II 2 H+ + ½ O2 H2O FADH2 FAD NADH NAD+ ADP + P i ATP (carrying electrons from food) H+ 1 Electron transport chain 2 Chemiosmosis Oxidative phosphorylation Matrix of mitochondria © 2018 Pearson Education Ltd. The Electron Transport Chain El H+ Stator INTERMEMBRANE SPACE Rotor Internal rod Catalytic knob ADP + Pi ATP MITOCHONDRIAL MATRIX © 2018 Pearson Education Ltd. Chemiosmosis: The Energy-Coupling Mechanism The energy released when electrons are passed down the electron transport chain, is used to pump H+ from the mitochondrial matrix to the inter-membrane space H+ then moves down its concentration gradient back across the membrane, passing through the protein complex ATP synthase H+ moves into binding sites on the rotor of ATP synthase, causing it to spin in a way that catalyses phosphorylation of ADP to ATP This is an example of chemiosmosis, the use of energy in a H+ gradient to drive cellular work © 2018 Pearson Education Ltd. Chemiosmosis: The Energy-Coupling Mechanism Certain electron carriers in the electron transport chain accept and release H+ along with the electrons In this way, the energy stored in a H+ gradient across a membrane couples the redox reactions of the electron transport chain to ATP synthesis The H+ gradient is referred to as a proton-motive force, emphasizing its capacity to do work Net production of ATP from 1 Glucose molecule NADH produced in the cytoplasm produces two ATP. NADH produced in the mitochondria produces three ATP. FADH2 produces two ATP. Pathway Products Total ATP Glycolysis 2 ATP, 2 Pyruvate 2 NADH = 4 ATP 6 Transition step 2 Acetyl CoA, 2 CO2 2 NADH = 6 ATP 6 TCA cycle 2 GTP, 4 CO2 6 NADH = 18 ATP 24 2 FADH2 = 4 ATP TOTAL 36 Anaplerotic Reactions of the TCA cycle Since the TCA cycle is involved in anabolism, this will tend to deplete the intermediates of the cycle, especially oxaloacetate on which the cycle depends. In Greek, ana means “up” and plerotikos means “to fill”. Anaplerotic Reactions of the TCA cycle The following four topping-up reactions are used to augment levels of TCA cycle intermediates: Pyruvate carboxylase i) Pyruvate + HCO 3- + ATP Oxaloacetate + ADP + Pi In Liver/Kidney PEP carboxylase ii) Phosphoenolpyruvate + HCO 3- Oxaloacetate + Pi In Higher plants, Yeast, Bacteria PEP carboxykinase iii) Phosphoenolpyruvate + CO 2 + GDP Oxaloacetate + GTP In Heart/Skeletal muscle M alic enzyme iv) Pyruvate + HCO 3- + NAD(P)H Malate + NAD(P) + Widely distributed in Eukaryotes and Prokaryotes