Electrochemistry Concepts PDF
Document Details
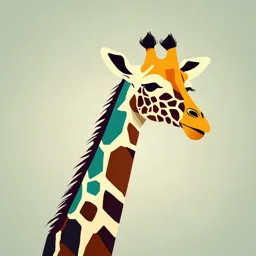
Uploaded by ComfortableNephrite297
Lawrence Technological University
Harris & Papaiconomou
Tags
Summary
This document discusses electrochemistry concepts, including redox reactions, galvanic cells, and standard hydrogen electrodes. It provides examples of different scenarios and calculations involving cell potentials. The text is focused on the basics of the subject matter.
Full Transcript
1.08 Cell Potential SCH4U Harris & Papaiconomou Consider the following individual possible reduction half cells: Zn Fe Zn2+ Fe2+ Both metal ions are oxidizing agents, ho...
1.08 Cell Potential SCH4U Harris & Papaiconomou Consider the following individual possible reduction half cells: Zn Fe Zn2+ Fe2+ Both metal ions are oxidizing agents, however, Fe2+ is a stronger oxidizing agent than Zn2+. Therefore, there would be a greater tendency for Fe2+ to gain e- (get reduced) than Zn2+. If we have a number of half cells we would like to rank, we need to introduce a standard half cell for the purpose of comparison. This is called the STANDARD HYDROGEN ELECTRODE (S.H.E) Standard Hydrogen Electrode red (SHE) ox /25°C This is a half cell which is used as a reference electrode. Since hydrogen is a gas (and not a metal) which is non-conducting, we need an inert electrode. Platinum wire is used for this purpose. How the SHE Works S.H.E. is set up as left-hand cell. Oxidation SHOULD occur here (convention). Two observations are made: 1. Voltmeter reading 2. Direction of e- flow (+/-/0) The value is recorded as a Standard Reduction Potential, Eor written as reduction in forward direction (see Appendix C p. 805) Scenario 1: SHE/SHE V H2(g) Pt(s) H+(aq) Neither OA is stronger /more competitive for electrons. No electron flow. Scenario 2: SHE/Ag V H2(g) Pt(s) Ag(s) WRA cathode anode (+) (--) H+(aq) Ag+(aq) magnitude high = SOA high e- flow More +ve, the more the OA is strong Scenario 3: SHE/Cu V H2(g) Pt(s) Cu(s) WRA cathode anode (+) (--) H+(aq) Cu2+(aq) magnitude lower = SOA lower e- flow Scenario 4: SHE/Mg V H2(g) Pt(s) Mg(s) cathode SRA anode (+) (--) H+(aq) Mg2+(aq) magnitude high WOA = high e- flow More -ve, the more the RA is strong Calculating Standard Cell Potential The Standard Cell Potential, ΔEo, is the difference between the reduction potentials of two half-cells. It is also shown as E◦cell If ΔEo is positive, the reaction is spontaneous as written (ie, cell notation follows convention). If it is negative, the reaction will not occur as written (ie, cell notation is backwards). NOTE: Eo values on your redox table are all reported as reduction potentials (shown as Eor) Method 1: E◦cell = E◦r cathode – E◦r anode Method 2: E◦cell = E◦red + E◦ox Example: Calculate the cell potential for the following galvanic cell: Cu ⏐Cu2+⏐⏐ Ag+ ⏐Ag anode cathode changed sign since oxidation; table only has reduction values Method 1: Method 2: E◦cell = E◦cathode – E◦anode E◦cell = E◦red + E◦ox E◦cell = 0.80 V – (+0.34 V) E◦cell = 0.80 V+ (– 0.34 V) = 0.46 V = 0.46 V Galvanic Cells SCH4U Harris & Papaiconomou 1 Spontaneity SOA WRA Consider a reaction between a solution of silver nitrate and copper metal. Reaction: Net ionic equation: Will this reaction be spontaneous? Yes! Silver ions are strong oxidizing agents while copper metal is a strong reducing agent. 2 WOA SRA Electrochemistry Concepts 1. Redox reactions involve the transfer of electrons from one reactant to another 2. Electric current is a flow of electrons in a circuit Electrochemistry - study of processes involved in converting chemical energy into electrical energy But how can we use these moving electrons to do work? We need to construct a mechanism to prevent direct contact of reactants of redox reactions. We must have electrons flow through an external circuit. 3 Consider the single displacement reaction between copper metal and silver nitrate set up this way: Observations: 1. Copper rod loses some of its mass 0.460 V (as copper metal is converted to copper (11) ions) Cu(s) → Cu2+ (aq) +2𝑒 oxidation half reaction 2. Silver rod gains some mass (silver ions in solution are converted to silver metal) 2 Ag + (aq) +2𝑒 →2 Ag (s) reduction half reaction 3. The voltmeter connected to the wire shows that electricity is flowing. Electrons flow from the copper rod towards the silver rod 4 Cu(s)→ Cu2+ (aq) +2𝑒 2 Ag + (aq) +2𝑒 →2 Ag (s) Oxidation Half Cell Reduction Half Cell Anode (-) Cathode (+) Cu(s) SRA Ag+(s) SOA AN OX ATE RED CAT 5 SOA WRA Now, consider a reaction between a solution of iron (III) nitrate and copper metal. Reaction: Net ionic equation: Will this reaction be spontaneous? No! 6 WOA SRA Now, consider the single displacement reaction between copper metal and iron (III) nitrate in this set up: Observations: 0.01 V 1. Iron rod loses some of its mass (as iron metal is converted to iron (1I1) ions) Fe(s) → Fe3+ (aq) +3𝑒 oxidation half reaction 2. Copper rod gains some mass (copper (II) ions in solution are converted to copper metal) Cu (aq) +2𝑒 →Cu(s) reduction half reaction 3. The voltmeter connected to the wire shows that electricity is flowing. Electrons flow from the iron rod towards the copper rod 7 Cu (aq) +2𝑒 →Cu(s) Fe(s) → Fe3+ (aq) +3𝑒 Reduction Half Cell Oxidation Half Cell Cathode (+) Anode (-) Cu2+(aq) SOA Fe(s) SRA AN OX ATE RED CAT 8 Explanation of Observations In each of the examples taken in the previous slides, two possible oxidizing agents and two possible reducing agents are present. RA RA OA OA The set up allows the strongest oxidizing agent to undergo reduction and also the strongest reducing agent can undergo oxidation. The two processes take place at the metal rods. 9 This set up is a Galvanic Cell. A galvanic cell is one where a chemical reaction is used to produce an electric current. Also known as the electrochemical cell. Each beaker containing the solution in contact with its metal is called a half cell since a half reaction of the redox reaction occurs therein. One is oxidation and the other is reduction. The solution itself is called the electrolyte. The two metal rods can be called electrodes. Cathode is the electrode where reduction occurs. Ions come up to the cathode and accept electrons. This may involve metal deposition on the cathode. As electrons are removed from the cathode, it carries a (+) charge. Anode is the electrode where oxidation occurs. Ions leave their electrons behind on this electrode and enter the solution. Since electrons are released at the anode, it carries a (-) charge. 10 Salt Bridge As the cell functions, more are more iron atoms leave their electrons behind on the rod and are released into the solution as iron (III) ions. Thus the positive charge builds up in the right hand half cell. Similarly, as more and more copper ions accept electrons on the copper rod and are converted into copper metal. Thus, the negative ions (NO3-) in the half cell are now in larger concentration. Function of Salt Bridge What makes a Salt Bridge? 1. Complete the electrical circuit Glass tube filled with an electrolyte, like without introducing any more potassium nitrate solution. metal into the system. Ends are “stoppered” by cotton wool. This 2. Maintain electrical neutrality of prevents too much mixing of the contents each half cell. The ions migrate of the salt bridge with that of the beaker. into and out of the salt bridge in Electrolyte in the salt bridge is chosen so order to do so. that it doesn’t react with the contents of either beaker. 11 Summary of Galvanic Cell SRA: SOA: Oxidation Reduction Cu(s) → Cu2+ (aq) +2𝑒 2 Ag + (aq) +2𝑒 →2 Ag (s) 12 Cell Notation allows for a short-hand way to represent a galvanic cell | phase boundary (solid aqueous) || porous barrier or salt bridge , comma between ions if no phase boundary between two ions occurs in the following order anode | cation of anode || cation of cathode | cathode reducing agent oxidizing agent Spectator ions are usually omitted Zn | Zn2+ || Cu2+ | Cu Standard Cell Both electrolyte solution concentrations are 1 mol/L Temperature is 25°C 13 Inert Electrodes electrode made from a material that is neither a reactant nor a product of cell redox reaction allows for redox reaction of gasses or dissolved electrolytes oxidation: Pb Pb2+ + 2e- reduction: Fe3+ + e- Fe2+ e.g. Pb | Pb2+ || Fe3+ , Fe2+ | Pt inert electrode 14 Disposable Batteries 1. Wet Cell Battery - liquid with electrolytes 2. Dry Cell Battery Zn | Zn2+ || Mn4+, Mn3+ | C - paste with electrolytes - portable, cheap (A, AA, C, D) (a) Disposable Battery (Primary) - stop producing electricity when reactants are used up (b) Rechargeable Battery (Secondary) - regenerate reactants Anode: Zn container OX: Zn Zn2+ + 2e- Paste: MnO2, ZnCl2, NH4Cl, C Cathode: C graphite (inert cathode) RED: MnO2 + H2O + 2e- Mn2O3 + 2OH- 15 Disposable Batteries (cont’d) 3. Alkaline Cell Battery Zn | Zn2+ || Mn4+, Mn3+ | C - improved, longer lasting version of dry cell Anode: Zn container OX: Zn + OH- ZnO + H2O + 2e- Paste: MnO2, C, KOH Cathode: C graphite (inert cathode) RED: MnO2 + 2H2O + 2e- Mn(OH)2 + 2OH- 4. Button Cell Battery Zn | Zn2+ || Hg2+, Hg | Steel Zn | Zn2+ || Ag+, Ag | Steel - smaller than alkaline battery Anode: Zn container OX: Zn + OH- ZnO + H2O + 2e- Paste: MnO2, C, KOH and either (a) HgO or (b) Ag2O Cathode: Stainless steel (inert cathode) RED: (a) HgO + H2O + 2e- Hg + 2OH- RED: (b) Ag2O + H2O + 2e- 2Ag + 2OH- 16 Summary of Galvanic Cell e- e- e- e- Anode Cathode e- e- Reducing Oxidizing Agent Agent 17 Summary of Galvanic Cells The ANODE... The CATHODE... supplies electrons to the external accepts electrons from the external circuit (wire) circuit (wire) is the negative pole of the battery is the positive pole of the battery is the site of OXIDATION is the site of REDUCTION is written on the left hand side if the is written on the right hand side if the convention is followed convention is followed is the half-cell with the lowest is the half-cell with the highest electrode electrode potential potential may18decrease in mass may increase in mass Lesson 1.05 Predicting Redox Reactions & Spontaneity Rule SCH4U Harris & Papaiconomou Predicting Redox Reactions Activity Series Zn Pb Developing a Redox Table We can compare different metal ions and rank them by their strength as oxidizing agents. Consider the following table: strongest weakest oxidizing oxidizing agent agent SOA WOA Similarly, we can compare different metals and rank them by their strength as reducing agents. Consider the following table: strongest weakest reducing reducing agent agent SRA WRA We can use this data to create a table in which we write the forward and reverse reduction/oxidation reactions as equilibria (done above) that shows their rankings. By convention, the reactions are written as reductions in the forward direction, and the strongest OA are at the top of the table. SOA WRA (most reactive metal ion) SRA WOA (most reactive metal) Spontaneity Rule A redox reaction (in this case, a single displacement reaction) occurs only if a STRONG OXIDIZING AGENT reacts with a STRONG REDUCING AGENT. (i.e. if something from the TOP LEFT of the table above reacts with something from the BOTTOM RIGHT of the table) 🗷 🗷 SCH4U Harris & Papaiconomou Redox reactions are two half reactions oxidation half-reaction reduction half-reaction The half-reaction method balances redox reactions by separately considering each half-reaction, balancing each to ensure that the number of electrons lost in the oxidation half-reaction is equal to the number of electrons gained in the reduction half-reaction. For a complete redox reaction, you have to combine an oxidation half-reaction and a reduction half-reaction after balancing each individually No electrons appear in the overall redox reaction Step 1. Write unbalanced half reactions showing reactants/products Step 1. Write unbalanced half reactions showing reactants/products Step 2. Balance atoms (excluding O & H) Step 1. Write unbalanced half reactions showing reactants/products Step 2. Balance atoms (excluding O & H) Step 3. Balance oxygen by adding H2O Step 1. Write unbalanced half reactions showing reactants/products Step 2. Balance atoms (excluding O & H) Step 3. Balance oxygen by adding H2O Step 4. Balance hydrogen by adding H+ Step 1. Write unbalanced half reactions showing reactants/products Step 2. Balance atoms (excluding O & H) Step 3. Balance oxygen by adding H2O Step 4. Balance hydrogen by adding H+ Reactant: (-1) + 8(+1) = +7 Reactant side has 5 more +, Product: (+2) + (0) = +2 so it needs 5 e- Step 5. Balance charge by adding electrons. Step 1. Write unbalanced half reactions showing reactants/products Step 2. Balance atoms (excluding O & H) Step 3. Balance oxygen by adding H2O Step 4. Balance hydrogen by adding H+ Reactant: (-1) + 8(+1) = +7 Reactant side has 5 more +, Product: (+2) + (0) = +2 so it needs 5 e- Step 5. Balance charge by adding electrons. Step 1. Write unbalanced half 𝑆 𝑂 ⟶ 𝑆𝑂 reactions showing reactants/products Step 1. Write unbalanced half 𝑆 𝑂 ⟶ 𝑆𝑂 reactions showing reactants/products Step 2. Balance atoms (excluding O 𝑆 𝑂 ⟶ 2𝑆𝑂 & H) Step 1. Write unbalanced half 𝑆 𝑂 ⟶ 𝑆𝑂 reactions showing reactants/products Step 2. Balance atoms (excluding O 𝑆 𝑂 ⟶ 2𝑆𝑂 & H) Step 3. Balance oxygen by adding 𝑆 𝑂 + 3𝐻 𝑂 ⟶ 2𝑆𝑂 H2 O Step 1. Write unbalanced half 𝑆 𝑂 ⟶ 𝑆𝑂 reactions showing reactants/products Step 2. Balance atoms (excluding O 𝑆 𝑂 ⟶ 2𝑆𝑂 & H) Step 3. Balance oxygen by adding 𝑆 𝑂 + 3𝐻 𝑂 ⟶ 2𝑆𝑂 H2 O Step 4. Balance hydrogen by adding 𝑆 𝑂 + 3𝐻 𝑂 ⟶ 2𝑆𝑂 + 6𝐻 H+ Step 1. Write unbalanced half 𝑆 𝑂 ⟶ 𝑆𝑂 reactions showing reactants/products Step 2. Balance atoms (excluding O 𝑆 𝑂 ⟶ 2𝑆𝑂 & H) Step 3. Balance oxygen by adding 𝑆 𝑂 + 3𝐻 𝑂 ⟶ 2𝑆𝑂 H2 O Step 4. Balance hydrogen by adding 𝑆 𝑂 + 3𝐻 𝑂 ⟶ 2𝑆𝑂 + 6𝐻 H+ Step 5. Adjust for basic conditions 𝑆 𝑂 + 3𝐻 𝑂 + 6𝑂𝐻 ⟶ 2𝑆𝑂 + 6𝐻 + 6𝑂𝐻 by adding as many OH- to both sides as the number of H+ Step 1. Write unbalanced half 𝑆 𝑂 ⟶ 𝑆𝑂 reactions showing reactants/products Step 2. Balance atoms (excluding O 𝑆 𝑂 ⟶ 2𝑆𝑂 & H) Step 3. Balance oxygen by adding 𝑆 𝑂 + 3𝐻 𝑂 ⟶ 2𝑆𝑂 H2 O Step 4. Balance hydrogen by adding 𝑆 𝑂 + 3𝐻 𝑂 ⟶ 2𝑆𝑂 + 6𝐻 H+ Step 5. Adjust for basic conditions 𝑆 𝑂 + 3𝐻 𝑂 + 6𝑂𝐻 ⟶ 2𝑆𝑂 + 6𝐻 + 6𝑂𝐻 by adding as many OH- to both sides as the number of H+ Step 6. Simplify by combining H+ 𝑆 𝑂 + 3𝐻 𝑂 + 6𝑂𝐻 ⟶ 2𝑆𝑂 + 6𝐻 𝑂 and OH- to form H2O Step 1. Write unbalanced half 𝑆 𝑂 ⟶ 𝑆𝑂 reactions showing reactants/products Step 2. Balance atoms (excluding O 𝑆 𝑂 ⟶ 2𝑆𝑂 & H) Step 3. Balance oxygen by adding 𝑆 𝑂 + 3𝐻 𝑂 ⟶ 2𝑆𝑂 H2 O Step 4. Balance hydrogen by adding 𝑆 𝑂 + 3𝐻 𝑂 ⟶ 2𝑆𝑂 + 6𝐻 H+ Step 5. Adjust for basic conditions 𝑆 𝑂 + 3𝐻 𝑂 + 6𝑂𝐻 ⟶ 2𝑆𝑂 + 6𝐻 + 6𝑂𝐻 by adding as many OH- to both sides as the number of H+ Step 6. Simplify by combining H+ 𝑆 𝑂 + 3𝐻 𝑂 + 6𝑂𝐻 ⟶ 2𝑆𝑂 + 6𝐻 𝑂 and OH- to form H2O Step 7. Simplify H2O molecules 𝑆 𝑂 + 6𝑂𝐻 ⟶ 2𝑆𝑂 + 3𝐻 𝑂 Step 1. Write unbalanced half 𝑆 𝑂 ⟶ 𝑆𝑂 reactions showing reactants/products Step 2. Balance atoms (excluding O 𝑆 𝑂 ⟶ 2𝑆𝑂 & H) Step 3. Balance oxygen by adding 𝑆 𝑂 + 3𝐻 𝑂 ⟶ 2𝑆𝑂 H2 O Step 4. Balance hydrogen by adding 𝑆 𝑂 + 3𝐻 𝑂 ⟶ 2𝑆𝑂 + 6𝐻 H+ Step 5. Adjust for basic conditions 𝑆 𝑂 + 3𝐻 𝑂 + 6𝑂𝐻 ⟶ 2𝑆𝑂 + 6𝐻 + 6𝑂𝐻 by adding as many OH- to both sides as the number of H+ Step 6. Simplify by combining H+ 𝑆 𝑂 + 3𝐻 𝑂 + 6𝑂𝐻 ⟶ 2𝑆𝑂 + 6𝐻 𝑂 and OH- to form H2O Step 7. Simplify H2O molecules 𝑆 𝑂 + 6𝑂𝐻 ⟶ 2𝑆𝑂 + 3𝐻 𝑂 Step 8. Balance charge by adding Reactant: (-2) + 6(-1) = -8 Reactant side has 4 more negative charges, electrons. Product: 2(-2) + (0) = -4 so product side needs 4 electrons Step 1. Write unbalanced half 𝑆 𝑂 ⟶ 𝑆𝑂 reactions showing reactants/products Step 2. Balance atoms (excluding O 𝑆 𝑂 ⟶ 2𝑆𝑂 & H) Step 3. Balance oxygen by adding 𝑆 𝑂 + 3𝐻 𝑂 ⟶ 2𝑆𝑂 H2 O Step 4. Balance hydrogen by adding 𝑆 𝑂 + 3𝐻 𝑂 ⟶ 2𝑆𝑂 + 6𝐻 H+ Step 5. Adjust for basic conditions 𝑆 𝑂 + 3𝐻 𝑂 + 6𝑂𝐻 ⟶ 2𝑆𝑂 + 6𝐻 + 6𝑂𝐻 by adding as many OH- to both sides as the number of H+ Step 6. Simplify by combining H+ 𝑆 𝑂 + 3𝐻 𝑂 + 6𝑂𝐻 ⟶ 2𝑆𝑂 + 6𝐻 𝑂 and OH- to form H2O Step 7. Simplify H2O molecules 𝑆 𝑂 + 6𝑂𝐻 ⟶ 2𝑆𝑂 + 3𝐻 𝑂 Step 8. Balance charge by adding Reactant: (-2) + 6(-1) = -8 Reactant side has 4 more negative charges, electrons. Product: 2(-2) + (0) = -4 so product side needs 4 electrons 𝑆 𝑂 + 6𝑂𝐻 ⟶ 2𝑆𝑂 + 3𝐻 𝑂 + 4𝑒 Balancing a Redox Reaction using the Half-Reaction Method Step 1. Write unbalanced net ionic 𝑀𝑔 + 𝐴𝑙 𝑁𝑂 ⟶? equation Balancing a Redox Reaction using the Half-Reaction Method Step 1. Write unbalanced net ionic 𝑀𝑔 + 𝐴𝑙 𝑁𝑂 ⟶? equation Step 2. Divide into oxidation half-reaction and reduction half-reaction. 𝑀𝑔 → 𝑀𝑔 𝐴𝑙 → 𝐴𝑙 Balancing a Redox Reaction using the Half-Reaction Method Step 1. Write unbalanced net ionic 𝑀𝑔 + 𝐴𝑙 𝑁𝑂 ⟶? equation Step 2. Divide into oxidation half-reaction and reduction half-reaction. 𝑀𝑔 → 𝑀𝑔 𝐴𝑙 → 𝐴𝑙 Step 3. Independently balance oxidation & reduction half-reaction. 𝑀𝑔 → 𝑀𝑔 + 2𝑒 𝐴𝑙 + 3𝑒 → 𝐴𝑙 Balancing a Redox Reaction using the Half-Reaction Method Step 1. Write unbalanced net ionic 𝑀𝑔 + 𝐴𝑙 𝑁𝑂 ⟶? equation Step 2. Divide into oxidation half-reaction and reduction half-reaction. 𝑀𝑔 → 𝑀𝑔 𝐴𝑙 → 𝐴𝑙 Step 3. Independently balance oxidation & reduction half-reaction. 𝑀𝑔 → 𝑀𝑔 + 2𝑒 𝐴𝑙 + 3𝑒 → 𝐴𝑙 Step 4. Find LCM (lowest common multiple) of electrons in oxidation & reduction half- 𝐿𝐶𝑀 𝑜𝑓 2 & 3 𝑖𝑠 6 reactions Balancing a Redox Reaction using the Half-Reaction Method Step 1. Write unbalanced net ionic 𝑀𝑔 + 𝐴𝑙 𝑁𝑂 ⟶? equation Step 2. Divide into oxidation half-reaction and reduction half-reaction. 𝑀𝑔 → 𝑀𝑔 𝐴𝑙 → 𝐴𝑙 Step 3. Independently balance oxidation & reduction half-reaction. 𝑀𝑔 → 𝑀𝑔 + 2𝑒 𝐴𝑙 + 3𝑒 → 𝐴𝑙 Step 4. Find LCM (lowest common multiple) of electrons in oxidation & reduction half- 𝐿𝐶𝑀 𝑜𝑓 2 & 3 𝑖𝑠 6 reactions Step 5. Use LCM to determine coefficient for both half-reactions. 3𝑀𝑔 → 3𝑀𝑔 + 6𝑒 2𝐴𝑙 + 6𝑒 → 2𝐴𝑙 Balancing a Redox Reaction using the Half-Reaction Method Step 1. Write unbalanced net ionic 𝑀𝑔 + 𝐴𝑙 𝑁𝑂 ⟶? equation Step 2. Divide into oxidation half-reaction and reduction half-reaction. 𝑀𝑔 → 𝑀𝑔 𝐴𝑙 → 𝐴𝑙 Step 3. Independently balance oxidation & reduction half-reaction. 𝑀𝑔 → 𝑀𝑔 + 2𝑒 𝐴𝑙 + 3𝑒 → 𝐴𝑙 Step 4. Find LCM (lowest common multiple) of electrons in oxidation & reduction half- 𝐿𝐶𝑀 𝑜𝑓 2 & 3 𝑖𝑠 6 reactions Step 5. Use LCM to determine coefficient for both half-reactions. 3𝑀𝑔 → 3𝑀𝑔 + 6𝑒 2𝐴𝑙 + 6𝑒 → 2𝐴𝑙 Step 6. Add half-reactions with equal number of electrons. 3𝑀𝑔 + 2𝐴𝑙 + 6𝑒 → 3𝑀𝑔 + 6𝑒 + 2𝐴𝑙 Balancing a Redox Reaction using the Half-Reaction Method Step 1. Write unbalanced net ionic 𝑀𝑔 + 𝐴𝑙 𝑁𝑂 ⟶? equation Step 2. Divide into oxidation half-reaction and reduction half-reaction. 𝑀𝑔 → 𝑀𝑔 𝐴𝑙 → 𝐴𝑙 Step 3. Independently balance oxidation & reduction half-reaction. 𝑀𝑔 → 𝑀𝑔 + 2𝑒 𝐴𝑙 + 3𝑒 → 𝐴𝑙 Step 4. Find LCM (lowest common multiple) of electrons in oxidation & reduction half- 𝐿𝐶𝑀 𝑜𝑓 2 & 3 𝑖𝑠 6 reactions Step 5. Use LCM to determine coefficient for both half-reactions. 3𝑀𝑔 → 3𝑀𝑔 + 6𝑒 2𝐴𝑙 + 6𝑒 → 2𝐴𝑙 Step 6. Add half-reactions with equal number of electrons. 3𝑀𝑔 + 2𝐴𝑙 + 6𝑒 → 3𝑀𝑔 + 6𝑒 + 2𝐴𝑙 Step 7. Simplify electrons. 3𝑀𝑔 + 2𝐴𝑙 → 3𝑀𝑔 + 2𝐴𝑙 Balancing a Redox Reaction using the Half-Reaction Method Step 1. Write unbalanced net ionic 𝑀𝑔 + 𝐴𝑙 𝑁𝑂 ⟶? equation Step 2. Divide into oxidation half-reaction and reduction half-reaction. 𝑀𝑔 → 𝑀𝑔 𝐴𝑙 → 𝐴𝑙 Step 3. Independently balance oxidation & reduction half-reaction. 𝑀𝑔 → 𝑀𝑔 + 2𝑒 𝐴𝑙 + 3𝑒 → 𝐴𝑙 Step 4. Find LCM (lowest common multiple) of electrons in oxidation & reduction half- 𝐿𝐶𝑀 𝑜𝑓 2 & 3 𝑖𝑠 6 reactions Step 5. Use LCM to determine coefficient for both half-reactions. 3𝑀𝑔 → 3𝑀𝑔 + 6𝑒 2𝐴𝑙 + 6𝑒 → 2𝐴𝑙 Step 6. Add half-reactions with equal number of electrons. 3𝑀𝑔 + 2𝐴𝑙 + 6𝑒 → 3𝑀𝑔 + 6𝑒 + 2𝐴𝑙 Step 7. Simplify electrons. 3𝑀𝑔 + 2𝐴𝑙 → 3𝑀𝑔 + 2𝐴𝑙 Step 8. Include spectator ions to balance. 3𝑀𝑔 + 2𝐴𝑙(𝑁𝑂 ) → 3𝑀𝑔(𝑁𝑂 ) + 2𝐴𝑙 ** may be skipped ** Balancing a Redox Reaction using the Half-Reaction Method Step 1. Write unbalanced net ionic 𝑀𝑔 + 𝐴𝑙 𝑁𝑂 ⟶? equation Step 2. Divide into oxidation half-reaction and reduction half-reaction. 𝑀𝑔 → 𝑀𝑔 𝐴𝑙 → 𝐴𝑙 Step 3. Independently balance oxidation & reduction half-reaction. 𝑀𝑔 → 𝑀𝑔 + 2𝑒 𝐴𝑙 + 3𝑒 → 𝐴𝑙 Step 4. Find LCM (lowest common multiple) of electrons in oxidation & reduction half- 𝐿𝐶𝑀 𝑜𝑓 2 & 3 𝑖𝑠 6 reactions Step 5. Use LCM to determine coefficient for both half-reactions. 3𝑀𝑔 → 3𝑀𝑔 + 6𝑒 2𝐴𝑙 + 6𝑒 → 2𝐴𝑙 Step 6. Add half-reactions with equal number of electrons. 3𝑀𝑔 + 2𝐴𝑙 + 6𝑒 → 3𝑀𝑔 + 6𝑒 + 2𝐴𝑙 Step 7. Simplify electrons. 3𝑀𝑔 + 2𝐴𝑙 → 3𝑀𝑔 + 2𝐴𝑙 Step 8. Include spectator ions to balance. 3𝑀𝑔 + 2𝐴𝑙(𝑁𝑂 ) → 3𝑀𝑔(𝑁𝑂 ) + 2𝐴𝑙 ** may be skipped ** Step 9. Include states 3𝑀𝑔( ) + 2𝐴𝑙(𝑁𝑂 ) ( ) → 3𝑀𝑔(𝑁𝑂 ) ( ) + 2𝐴𝑙( ) Oxidation number (ON) method balances redox equations by ensuring that the total increase in the oxidation numbers of the oxidized element(s) equals the total decrease in the oxidation numbers of the reduced element(s). Recall: Oxidation – loss of electrons oxidation number increases Reduction – gain of electrons oxidation number decreases Balancing a Redox Reaction using the Oxidation Number Method Step 1. Write unbalanced equation. 𝑁𝐻 + 𝑂 ⟶ 𝑁𝑂 + 𝐻 𝑂 Balancing a Redox Reaction using the Oxidation Number Method Step 1. Write unbalanced equation. 𝑁𝐻 + 𝑂 ⟶ 𝑁𝑂 + 𝐻 𝑂 Step 2. Determine whether the reaction is a redox reaction by -3 +1 0 +4 -2 +1 -2 assigning an ON to each element wherever it appears in the equation. Balancing a Redox Reaction using the Oxidation Number Method Step 1. Write unbalanced equation. 𝑁𝐻 + 𝑂 ⟶ 𝑁𝑂 + 𝐻 𝑂 Step 2. Determine whether the reaction is a redox reaction by -3 +1 0 +4 -2 +1 -2 assigning an ON to each element wherever it appears in the equation. Step 3. If a redox reaction, identify element(s) with increase Increase in ON: N in ON and element(s) with decrease in ON. Decrease in ON: O Balancing a Redox Reaction using the Oxidation Number Method Step 1. Write unbalanced equation. 𝑁𝐻 + 𝑂 ⟶ 𝑁𝑂 + 𝐻 𝑂 Step 2. Determine whether the reaction is a redox reaction by -3 +1 0 +4 -2 +1 -2 assigning an ON to each element wherever it appears in the equation. Step 3. If a redox reaction, identify element(s) with increase Increase in ON: N in ON and element(s) with decrease in ON. Decrease in ON: O Step 4. Find numerical values of the increase and decrease of N: -3 +4, up by 7 ON. O: 0 -2, down by 2 Balancing a Redox Reaction using the Oxidation Number Method Step 1. Write unbalanced equation. 𝑁𝐻 + 𝑂 ⟶ 𝑁𝑂 + 𝐻 𝑂 Step 2. Determine whether the reaction is a redox reaction by -3 +1 0 +4 -2 +1 -2 assigning an ON to each element wherever it appears in the equation. Step 3. If a redox reaction, identify element(s) with increase Increase in ON: N in ON and element(s) with decrease in ON. Decrease in ON: O Step 4. Find numerical values of the increase and decrease of N: -3 +4, up by 7 ON. O: 0 -2, down by 2 Step 5. Find the LCM of the oxidized and reduced elements, so the total increase in ON equals the total decrease in ON. LCM of 7 and 2 is 14. Balancing a Redox Reaction using the Oxidation Number Method Step 1. Write unbalanced equation. 𝑁𝐻 + 𝑂 ⟶ 𝑁𝑂 + 𝐻 𝑂 Step 2. Determine whether the reaction is a redox reaction by -3 +1 0 +4 -2 +1 -2 assigning an ON to each element wherever it appears in the equation. Step 3. If a redox reaction, identify element(s) with increase Increase in ON: N in ON and element(s) with decrease in ON. Decrease in ON: O Step 4. Find numerical values of the increase and decrease of N: -3 +4, up by 7 ON. O: 0 -2, down by 2 Step 5. Find the LCM of the oxidized and reduced elements, so the total increase in ON equals the total decrease in ON. LCM of 7 and 2 is 14. Step 6. Use the LCM to balance the numbers of atoms of the 7 2𝑁𝐻 + 𝑂 ⟶ 𝑁𝑂 + 𝐻 𝑂 element(s) oxidized and reduced. 2 Balancing a Redox Reaction using the Oxidation Number Method Step 1. Write unbalanced equation. 𝑁𝐻 + 𝑂 ⟶ 𝑁𝑂 + 𝐻 𝑂 Step 2. Determine whether the reaction is a redox reaction by -3 +1 0 +4 -2 +1 -2 assigning an ON to each element wherever it appears in the equation. Step 3. If a redox reaction, identify element(s) with increase Increase in ON: N in ON and element(s) with decrease in ON. Decrease in ON: O Step 4. Find numerical values of the increase and decrease of N: -3 +4, up by 7 ON. O: 0 -2, down by 2 Step 5. Find the LCM of the oxidized and reduced elements, so the total increase in ON equals the total decrease in ON. LCM of 7 and 2 is 14. Step 6. Use the LCM to balance the numbers of atoms of the 7 2𝑁𝐻 + 𝑂 ⟶ 𝑁𝑂 + 𝐻 𝑂 element(s) oxidized and reduced. 2 7 2𝑁𝐻 + 𝑂 ⟶ 2𝑁𝑂 + 3𝐻 𝑂 2 Step 7. Balance the other elements by inspection. Balancing a Redox Reaction using the Oxidation Number Method Step 1. Write unbalanced equation. 𝑁𝐻 + 𝑂 ⟶ 𝑁𝑂 + 𝐻 𝑂 Step 2. Determine whether the reaction is a redox reaction by -3 +1 0 +4 -2 +1 -2 assigning an ON to each element wherever it appears in the equation. Step 3. If a redox reaction, identify element(s) with increase Increase in ON: N in ON and element(s) with decrease in ON. Decrease in ON: O Step 4. Find numerical values of the increase and decrease of N: -3 +4, up by 7 ON. O: 0 -2, down by 2 Step 5. Find the LCM of the oxidized and reduced elements, so the total increase in ON equals the total decrease in ON. LCM of 7 and 2 is 14. Step 6. Use the LCM to balance the numbers of atoms of the 7 2𝑁𝐻 + 𝑂 ⟶ 𝑁𝑂 + 𝐻 𝑂 element(s) oxidized and reduced. 2 7 2𝑁𝐻 + 𝑂 ⟶ 2𝑁𝑂 + 3𝐻 𝑂 2 Step 7. Balance the other elements by inspection. 4𝑁𝐻 + 7𝑂 ⟶ 4𝑁𝑂 + 6𝐻 𝑂 Step 8. For reactions that occur in acidic or basic solutions, include H2O, H+, or OH- as needed to balance the equation. Lesson 1.02 SCH4U Harris & Papaiconomou Representing Redox Reactions Consider: Mg (s) + 2 HCl (aq) MgCl2 (aq) + H2 (g) This is the overall equation for the redox reaction. Notice: - No electrons appear in the overall equation for redox reactions - It is not initially obvious that this is a redox reaction nor what is being reduced or oxidized. - Not all chemical species are involved in the redox reaction and thus can be eliminated by writing the net ionic equation. Overall Equation: Mg (s) + 2 HCl (aq) MgCl2 (aq) + H2 (g) Total Ionic Equation: Mg (s) + 2 H+ (aq) + 2 Cl- (aq) Mg2+ (aq) + 2 Cl- (aq) + H2 (g) Net Ionic Equation: Mg (s) + 2 H+ (aq) Mg2+ (aq) + H2 (g) Mg lost 2 e- to form Mg2+ Mg was oxidized 2 H+ each gained 1 e- to form H2, a net gain of 2 e- H+ was reduced Half Reactions - show oxidation and reduction independently Mg Mg2+ + 2 e- 2 H+ + 2 e- H2 Oxidation half reaction Reduction half reaction of magnesium. of hydrogen ions. Electrons appear on the Electrons appear on the right hand side of left hand side of oxidation half reactions. reduction half reactions. Some typical redox reactions: 1. Reaction between metal and non-metal (ionic substance formed) 2 Mg (s) + O2 (g) 2 MgO (s) 2. Reaction between a metal and dilute acids (ionic salt and hydrogen are formed) Mg(s) + 2HCl(aq) → MgCl2(aq) + H2(aq) 3. Single displacement reaction between reactive metal and the salt of a less reactive metal Example: Overall Equation: Zn(s) + CuSO4(aq) → ZnSO4(aq) + Cu(s) Ionic equation: Zn(s) + Cu2+(aq) → Zn2+(aq) + Cu(s) ½ rxn for oxidation: Zn(s) → Zn2+(aq) + 2e ½ rxn for reduction: Cu2+(aq) + 2e → Cu(s) Disproportionation reaction is a redox reaction where the same substance is simultaneously reduced and oxidized. Example: Word equation: copper (I) iodide → copper (II) iodide + copper Overall equation: 2CuI (aq) → CuI2 (aq) + Cu(s) Net Ionic equation: 2Cu+ (aq) → Cu2+ (aq) + Cu (s) ½ rxn for oxidation: Cu+ (aq) → Cu2+ (aq) + e ½ rxn for reduction: Cu+ (aq) + e → Cu (s) Can redox reactions be observed in covalent substances (where electrons are shared between 2 nuclei of atoms)? Consider : 2 H2(g) + O2(g) → 2 H2O(l) Both reactants have bonding that is pure covalent due to equal electron sharing (∆EN=0) However, the product has bonding that is polar covalent due to unequal electron sharing between H and O. Thus during the formation of water, Each of the four hydrogen atoms lost partial control of each one’s lone valence electrons Each of the two oxygen atoms gained partial control of two electrons which were previously not its own. Thus hydrogen has been oxidized and oxygen has been reduced. The ‘partial’ movement of electrons within covalent bonds can be shown more easily by using oxidation numbers Oxidation numbers are assigned to each atom within a molecule The magnitude of the oxidation number shows the number of electrons an atom may lose or gain control of in polar covalent bonding. Oxidation numbers can be positive or negative or zero Positive Sign Negative Sign Zero Atom LOSES control Atom GAINS Equal electron of e-. control of e-. sharing. Using oxidation numbers to predict oxidation and reduction: When the oxidation When the oxidation number of an atom number of an atom INCREASES, it is REDUCES, it is undergoing OXIDATION undergoing REDUCTION. The oxidation number The oxidation number of on carbon increases each oxygen reduces from 0 to +4 from 0 to –2 (OXIDATION) (REDUCTION) Determination of Oxidation Numbers: The oxidation of atoms in elements is zero. The oxidation numbers on cations and anions is the same as the charge on the ions. The oxidation number of hydrogen atom is +1 (except in hydrides where it is –1). The oxidation number on oxygen atom is taken to be –2 (except in hydrogen peroxide, H2O2, where it is –1 & OF2 where it is +2). Oxidation number of group I elements is +1, of group II elements is +2 and of aluminum is +3. Oxidation number of halogen atoms is –1. So what about all the other elements?? It can be deduced since… The total of all oxidation numbers within a neutral molecule is zero. The total of all oxidation numbers within a polyatomic ion is the same as the charge on the ion. ** Note, for oxidation number the sign comes BEFORE the number, as opposed to ion charge when the sign comes after the number. ** Rules Oxidation Number Examples Pure Element 0 Na(s) : 0 Element in Monatomic Ion Charge of ion Na+(aq) : +1 Hydrogen - most compounds +1 H2S : +1 - metal hydrides -1 NaH : -1 Oxygen - most compounds -2 Li2O : -2 - peroxides -1 H2O2 : -1 - OF2 +2 OF2 : +2 Covalent compound without More EN element’s ON equals PCl3 : P +3, Cl -1 H or O its usual ionic charge CS2 : C +4, S -2 Sum of ON in a compound CF4 : F -1, so C +4 =0 Sum of ON of polyatomic ion NO2— : O -2, N (+3) = charge of ion (+3) + 2(-2) = -1 𝑍𝑛 ⟶ 𝑍𝑛 + 2𝑒 lose 2 e- ON: 0 +2 Zn undergoes oxidation: increase in ON 𝐶𝑢 + 2𝑒 ⟶ 𝐶𝑢 gain 2 e- ON: +2 0 Cu2+ undergoes reduction: decrease in ON gain 2 e- ( ) ( ) () ON: 0 0 +1 -2 lose 1 e- twice So, H undergoes oxidation, O undergoes reduction Lesson 1.01 SCH4U - Electrochemistry Harris & Papaiconomou A redox reaction is the name given to a reaction which involves movement of electrons between the REACTANTS. One reactant is the electron donor. The other reactant is the electron acceptor. Redox consists of two sub-steps: REDuction is the process involving gain of electrons. OXidation is the process involving loss of electrons. Mnemonics LEO the lion says GER OIL RIG Consider the reaction between magnesium and oxygen. 2 Mg (s) + O2 (g) 2 MgO (s) * Note MgO is ionic and is a crystal lattice of alternating ions Mg2+& O2- * Each magnesium Each atom of oxygen atom loses two in O2 molecule gains electrons. two electrons. Magnesium atom (Mg) Oxygen atoms (O) undergoes oxidation undergo reduction to form to form Magnesium ions Oxygen ions (O2-) (Mg2+) 2 Mg (s) + O2 (g) 2 MgO (s) Magnesium provides Oxygen helps to oxygen with remove electrons electrons. from magnesium. Mg helps O2 reduce / O2 helps Mg oxidize / Mg acts as a O2 acts as an oxidizing reducing agent. agent. Reducing agents are Oxidizing agents are electron donors. electron acceptors. 2 Mg (s) + O2 (g) 2 MgO (s) Magnesium atoms Oxygen atoms themselves themselves undergo oxidation undergo reduction and and act as reducing agents act as oxidizing agents Oxidizing agents are reduced while reducing agents are oxidized. Redox reactions can, therefore, be described as a reaction where a flow of electrons is observed from a reducing agent to an oxidizing agent. These processes need to run concurrently. If one reactant is oxidized then another reactant is reduced. Note: In a redox reaction, the reducing agent and oxidizing agent are always located amongst the reactants. CLASSIFICATION OF SOLIDS SCH4U – STRUCTURE & PROPERTIES OF MATTER HARRIS & PAPAICONOMOU CLASSIFICATION OF SOLIDS When describing physical properties of a pure substance (many of which are solids under ordinary room conditions) we are not referring to a single particle, but to an aggregate of particles held together by attractive forces. When classifying such solids, we consider: ❑ Structural units (particles) that may up the aggregates (collections) ❑ Type of bond or force between the units ❑ Regularity/irregularity of the organization of the units. We can then use this information to predict/explain properties of such substance. IONIC SOLIDS (Ionic Crystals) Composed of: oppositely charged ions (cations/anions) Held together by: electrostatic attractions (ionic bonds – “directional”, rigid, fixed) In: regular crystal lattice structure e.g. NaCl, K2SO4, CoCl2·5H2O Properties: hard & brittle very high melting points poor conductor of electricity COVALENT NETWORK SOLIDS (Giant Molecule) Composed of: enormous network of atoms Held together by: covalent bonds In: regular crystal lattice structure e.g. diamond [C(dia)] graphite [C(gr)] quartz [SiO2] silicon Properties: very hard & strong often poor conductor of electricity very high melting points ALLOTROPES OF CARBON Covalent networks are remarkably diverse and useful. Carbon can create such diverse structures as: diamond (tetrahedral arrangement) graphite (planar arrangement) buckminsterfullerene (a soccer ball arrangement) “delocalized electrons” - conducts e- carbon nanotubes - lubricant (thin tubes used for optics and conduction experiments) graphene (one layer of graphite) Graphene: Buckyball & Nanotube: Watch video at 2:20 min https://www.youtube.com/watch?v=Mcg9_ML2mXY https://www.youtube.com/watch?v=ndJlgTkm0oQ MOLECULAR SOLIDS (Molecular Crystals) Composed of: polar or non-polar molecules Held together by: intermolecular forces In: regular crystal lattice structure e.g. S8, CH4, H2O, C12H22O11, I2, Cℓ2, CO2, NH3 Properties: vary based on which intermolecular forces present fairly soft poor conductors of electricity low to moderate melting points PROPERTIES OF MOLECULAR SOLIDS METALLIC SOLIDS Composed of: Properties: fixed positive metallic ions soft to very hard surrounded by a “sea” of delocalized electrons malleable & ductile excellent conductors of Held together by: electricity metallic bond (non-directional bond) low to very high melting points In: regular crystal lattice structure e.g. Na, Fe, Cu ATOMIC SOLIDS Composed of: Noble gas atoms Held together by: London dispersion forces In: regular crystal lattice structure e.g. He Properties: soft poor conductors of electricity very low melting points AMORPHOUS SOLIDS (Non-crystalline solids) Composed of: atoms Held together by: covalent bonds In: no fixed arrangement (irregular) e.g. glass, amber Properties: soft poor conductors of electricity low melting points GLASS ❑ When heated beyond it’s MP, then cooled quickly, SiO2 changes from a) “sand” to b) “glass” ❑ SiO2 glass can be strengthened by adding B2O3, used in lab and kitchen glassware (Pyrex) WHY STUDY Understanding the properties of solids allows use novel MATERIAL SCIENCE? solids in engineering. Source RECALL THESE CONCEPTS: electronegativity bonding continuum covalent bond non-polar bond polar bond ionic bond MOLECULAR POLARITY SCH4U – STRUCTURE AND PROPERTIES OF MATTER HARRIS & PAPAICONOMOU POLARITY OF MOLECULES Recall that bond polarity is determined by calculating the electronegativity (EN) difference between two atoms in a bond. e.g. H –– Cℓ ENH = 2.20 ENCℓ = 3.16 ΔENCℓ-H = 3.16 – 2.20 = 0.96 ∴The bond is polar. ▪ In this example, the polar bond results in a polar molecule. This is NOT always the case for more complex molecules. HOW CAN MOLECULAR POLARITY BE DETERMINED EXPERIMENTALLY? Subject molecules to an electric field and determine whether their orientation changes. No Electric Field Electric Field Non Polar Molecules Polar Molecules HOW CAN MOLECULAR POLARITY BE DETERMINED BY INSPECTION? Two things must be considered when attempting to determine molecular polarity: 1. The Presence/Absence of Polar Bonds 1. The Shape of Molecules (Draw Lewis Structures & Use VSEPR Theory) EXAMPLE 1: BeH2 I. Lewis Structure The central atom has 2 bonding electron pairs and no lone electron pairs II. Shape (VSEPR) ∴ The shape is linear. (AX2) III. Bond Polarity ΔENH-Be = ENH – ENBe = 2.20 – 1.57 = 0.63 ∴ The bonds are polar. “individual bond” dipole IV. Shape Diagrams with Bond Dipoles The bond dipoles are equal in strength and symmetrically V. Molecular Polarity arranged around the central atom and thus cancel out. ∴ The molecule is non-polar. EXAMPLE 2: PH3 I. Lewis Structure The central atom has 3 bonding electron pairs and 1 lone electron pair II. Shape (VSEPR) ∴ The shape is trigonal pyramidal. (AX3E) III. Bond Polarity ΔENH-P = ENH – ENP = 2.20 – 2.19 = 0.01 ∴ The bonds are non-polar. “cumulative”/”molecular” dipole IV. Shape Diagrams with Bond Dipoles V. Molecular Polarity This molecule has no bond dipoles, however, the presence of the lone electron pair on the central atom creates a molecular dipole.∴ The molecule is polar. EXAMPLE 3: CCℓ4 I. Lewis Structure The central atom has 4 bonding electron pairs and 0 lone electron pair II. Shape (VSEPR) ∴ The shape is tetrahedral. (AX4) ΔENCℓ-C = ENCℓ – ENC = 3.16 – 2.55 = 0.61 ∴ The bonds are polar. III. Bond Polarity IV. Shape Diagrams with Bond Dipoles V. Molecular Polarity This molecule has bond dipoles which are equal in strength but symmetrically- arranged around the central atom. These bond dipoles cancel out. ∴ The molecule is non-polar. EXAMPLE 4: CHCℓ3 I. Lewis Structure The central atom has 4 bonding electron pairs and 0 lone electron pair II. Shape (VSEPR) ∴ The shape is tetrahedral. (AX4) ΔENCℓ-C = ENCℓ – ENC = 3.16 – 2.55 = 0.61 ∴ The bonds are polar. III. Bond Polarity ΔENC-H = ENC – ENH = 2.55 – 2.20 = 0.35 ∴ The bonds are non-polar. IV. Shape Diagrams with Bond Dipoles V. Molecular Polarity This molecule has 3 bond dipoles which are equal in strength but are not symmetrically-arranged around the central atom. These bond dipoles add up to a cumulative molecular dipole. ∴ The molecule is polar. EXAMPLE 5: CFCℓ3 I. Lewis Structure The central atom has 4 bonding electron pairs and 0 lone electron pair II. Shape (VSEPR) ∴ The shape is tetrahedral. (AX4) ΔENCℓ-C = ENCℓ – ENC = 3.16 – 2.55 = 0.61 ∴ The bonds are polar. III. Bond Polarity ΔENF-C = ENF – ENC = 3.98 – 2.55 = 1.43 ∴ The bonds are VERY polar. IV. Shape Diagrams with Bond Dipoles V. Molecular Polarity This molecule has 3 bond dipoles which are equal in strength and another bond dipole that is greater in strength. Although they are symmetrically-arranged around the central atom, they do not cancel out but rather add up to a cumulative molecular dipole. ∴ The molecule is polar. EXAMPLE 6: NH3 I. Lewis Structure The central atom has 3 bonding electron pairs and 1 lone electron pair II. Shape (VSEPR) ∴ The shape is trigonal pyramidal. (AX3E) ΔENN-H = ENN – ENH = 3.04 – 2.20 = 0.84 ∴ The bonds are polar. III. Bond Polarity IV. Shape Diagrams with Bond Dipoles V. Molecular Polarity The bond dipoles are equal in strength but not arranged around the central atom symmetrically. These bond dipoles do not cancel out. ∴ The molecule is polar. Comparing and Ranking Intermolecular Forces Worksheet SCH4U – Gr12 Chemistry Ms. Harris & Ms. Papaiconomou BeH2 H2S H2O sp hybridized; 2 e- groups sp3 hybridized; 4 e- groups sp3 hybridized; 4 e- groups AX2 AX2E2 AX2E2 ∴ molecule is linear ∴ molecule is angular/bent ∴ molecule is angular/bent ΔEN = 0.63 ΔEN = 0.38 ΔEN = 1.24 (polar covalent bond) (non-polar covalent bond) (polar covalent bond) Bond dipoles symmetrical No bond dipoles but two lone Bond dipoles not around central atom and ∴ pairs on central atom, symmetrical around central cancel, ∴ molecule is polar atom and ∴do not cancel ∴ molecule is non-polar ∴ molecule is polar IMF is London forces IMF is dipole-dipole & IMF is hydrogen bonding London forces & London forces Rank (strongest 🡪 weakest): H2O, H2S, BeH2 HCℓ HBr not hybridized not hybridized 1 σ bond 1 σ bond ∴ molecule is linear ∴ molecule is linear ΔEN = 0.96 ΔEN = 0.76 (polar covalent bond) (polar covalent bond) 1 dipole 1 dipole ∴ molecule is polar ∴ molecule is polar IMF is dipole-dipole & London forces IMF is dipole-dipole & London forces * stronger DD force, since greater ΔEN *stronger LF since more e- Rank (strongest 🡪 weakest): HBr (bp = -66°C), HCℓ(bp=-85°C) PH3 AsCℓ3 sp3 hybridized; 4 e- groups sp3 hybridized; 4 e- groups AX3E1 AX3E1 ∴ molecule is trigonal pyramidal ∴ molecule is trigonal pyramidal ΔEN = 0.01 (non-polar covalent bond) ΔEN = 0.98 (polar covalent bond) total # e- = 18 e- total # e- = 84 e- No bond dipoles, but lone pair on the central dipoles not symmetrical around central atom atom ∴ do not cancel ∴ molecule is polar ∴ molecule is polar IMF is dipole-dipole & London forces IMF is dipole-dipole & London forces * Stronger IMF since stronger LF, higher #e- s Rank (strongest 🡪 weakest): AsCℓ3, PH3 SiF4 SiCℓ4 SiBr4 sp3 hybridized; 4 e- groups sp3 hybridized; 4 e- groups sp3 hybridized; 4 e- groups AX4 AX4 AX4 ∴ molecule is tetrahedral ∴ molecule is tetrahedral ∴ molecule is tetrahedral ΔEN = 2.08 ΔEN = 1.26 ΔEN = 1.06 (very polar covalent bond) (polar covalent bond) (polar covalent bond) dipoles symmetrical around dipoles symmetrical around dipoles symmetrical around central atom and ∴cancel central atom and ∴cancel central atom and ∴cancel ∴ molecule is non-polar ∴ molecule is non-polar ∴ molecule is non-polar IMF is London forces IMF is London forces IMF is London forces total #e-s = 50 e- total #e-s = 82 e- total #e-s = 154 e- Rank (strongest 🡪 weakest): SiBr4, SiCl4, SiF4 VSEPR Molecule Intermolecular Compound Bond Polarity shape Polarity Force H2 linear ΔENH-H = 0.00 non-polar non-polar London Ne - (atom) - - London ΔENC-H = 0.45 polar polar London CH2Cℓ2 tetrahedral (unsymmetrical ΔENC-Cl = 0.61 polar bond dipoles) dipole-dipole ΔENN-H = 0.94 polar polar trigonal London NH3 & 1 lone pair e-s on N (unsymmetrical pyramidal bond dipoles) hydrogen bonding & N-H bond present polar London HCℓ linear ΔENH-Cl = 1.06 polar (unsymmetrical bond dipoles) dipole-dipole ΔENO-H = 1.34 polar polar angular/ London H2O & 2 lone pair e-s on O (unsymmetrical bent bond dipoles) hydrogen bonding & O-H bond present trigonal ΔENN-F = 0.94 polar polar London NF3 (unsymmetrical pyramidal & 1 lone pair e-s on N bond dipoles) dipole-dipole BONDING AND THE MODERN ATOM & VSEPR THEORY SCH4U – STRUCTURE AND PROPERTIES OF MATTER HARRIS & PAPAICONOMOU Hybridization of CH4 Explained Example with Diagram Showing Changes in Diagram of Hybridized Type of Hybridization Orbital Diagram of Orbital Configuration Orbitals Central Atom ground C excited C 4 x sp3 hybrid electron promotion orbitals ↑ ↑ ↑ ↑ ↑ ↑ ↑ ↑ ↑ sp3 ↑↓ ↑ hybridization Hybrid Orbitals 109.5◦ ↑↓ ↑↓ What about the hybridization of ammonia? Example with Diagram Showing Changes in Diagram of Hybridized Type of Hybridization Orbital Diagram of Orbital Configuration Orbitals Central Atom ground N hybridization without 4 x sp3 electron promotion hybrid orbitals ↑ ↑ ↑ ↑ ↑ ↑ ↑↓ ↑ ↑ ↑ sp3 ↑↓ ↑↓ 107.3◦ hybridization Hybrid Orbitals ↑↓ ↑↓ What about the hybridization of water? Example with Diagram Showing Changes in Diagram of Hybridized Type of Hybridization Orbital Diagram of Orbital Configuration Orbitals Central Atom ground O hybridization without 4 x sp3 electron promotion hybrid orbitals sp3 ↑↓ ↑ ↑ ↑↓ ↑ ↑ ↑↓ ↑↓ ↑ ↑ hybridization ↑↓ ↑↓ Hybrid Orbitals ↑↓ ↑↓ 104.5◦ Methane, CH4 Ammonia, NH3 Water, H2O Yes No No Does hybridization involve (central atom only had two (central atom already had the (central atom already had the electron promotion? unpaired electrons while four necessary three unpaired necessary two unpaired was needed) electrons) electrons) Forming four equivalent hybrid orbitals allows them Why do hybrids form? to assume angles which maximizes their distance apart and therefore minimizes the repulsion between the bonding electrons. Diagram of molecule showing hybrid orbitals and sigma bonds to pure s orbital of hydrogen Molecular Shape and Bond tetrahedral trigonal pyramidal bent Angles 109.5◦ 107.3◦ 104.5◦ ETHENE HYBRIDIZATION IN ETHENE Electron promotion and hybridization in Carbon EXPLANATION OF THE HYBRIDIZATION OF ETHENE ◼ In ethene molecule two such carbon atoms lie side by side. ◼ One of the hybrid orbitals are involved in a sigma bond with the second carbon atom. ◼ The other two hybrid orbitals are involved in sigma bonds with two hydrogen atoms. Note that all atoms lie on the same plane. ◼ The pure 2p in each carbon undergoes sideways overlap to form a π (pi) bond. Summary of bonding in ethene: ETHYNE HYBRIDIZATION IN ETHYNE Electron promotion and hybridization in Carbon EXPLANATION OF THE HYBRIDIZATION OF ETHYNE ◼ In ethyne, two such carbon atoms lie side by side. ◼ One of the hybrid orbitals are involved in a sigma bond with the second carbon atom. ◼ The other hybrid orbital is involved in a sigma bond with a hydrogen atom. Note that all atoms are linearly arranged. ◼ The 2 pure 2p in each carbon undergo sideways overlap to form a 2 π (pi) bonds. Summary of bonding in ethyne: PCℓ5 Hybridization of PCℓ5 Explained Example with Diagram Showing Changes in Diagram of Hybridized Type of Hybridization Orbital Diagram of Orbital Configuration Orbitals Central Atom ground P excited P ↑ ↑ ↑ ↑ ↑ ↑ 5 x sp3d hybrid orbitals electron promotion sp3d hybridization SF6 Hybridization of SF6 Explained Example with Diagram Showing Changes in Diagram of Hybridized Type of Hybridization Orbital Diagram of Orbital Configuration Orbitals Central Atom ground S excited S ↑ ↑ ↑ ↑ ↑ ↑ ↑ ↑ ↑ 6 x sp3d2 hybrid orbitals electron promotion sp3d2 hybridization VSEPR ◼ Valence Shell Electron Pair Repulsion Theory ◼ Used to predict shapes and bond angles of simple molecules ◼ Electron pairs (both bonding and lone pairs) are distributed equally about a central atom in such a way as to minimize repulsion GEOMETRIES FOR SPECIES WITH TWO TO SIX ELECTRON GROUPS Groups are placed around the central atom in a way that produces a molecular structure with the lowest energy. That is, the one that minimizes repulsions. CALCULATING THE NUMBER OF ELECTRON PAIRS/GROUPS ON THE CENTRAL ATOM ◼ ELECTRON PAIR GEOMETRY: LINEAR Molecular Geometry: Linear Bonding Pairs: 2 Lone Pairs: 0 AXmEn designation: AX2 Image ELECTRON PAIR GEOMETRY: TRIGONAL PLANAR Molecular Geometry: Trigonal Planar Bent / Angular Bonding Pairs: 3 2 Lone Pairs: 0 1 AXmEn designation: AX3 AX2E Image ELECTRON PAIR GEOMETRY: TETRAHEDRAL Molecular Trigonal Tetrahedral Bent / Angular Geometry: Pyramidal Bonding Pairs: 4 3 2 Lone Pairs: 0 1 2 AXmEn designation: AX4 AX3E AX2E2 Image ELECTRON PAIR GEOMETRY: TRIGONAL BIPYRAMIDAL Molecular Trigonal Seesaw T-shaped Linear Geometry: Bipyramidal Bonding Pairs: 5 4 3 2 Lone Pairs: 0 1 2 3 AXmEn designation: AX5 AX4E AX3E2 AX2E3 Image ELECTRON PAIR GEOMETRY: OCTAHEDRAL Molecular Square Square Octahedral Geometry: Pyramidal Planar Bonding Pairs: 6 5 4 Lone Pairs: 0 1 2 AXmEn designation: AX6 AX5E AX4E2 Image VSEPR SUMMARY LEWIS THEORY OF BONDING SCH4U – STRUCTURE & PROPERTIES OF MATTER HARRIS & PAPAICONOMOU LEWIS THEORY OF BONDING Gilbert Lewis – used knowledge of chemical formulas, the octet rule, the concept of valence and the electron-shell model of the atom to explain chemical bonding Lewis Theory: Noble gas electron configurations are stable (“stable octet”) Electrons are most stable as pairs Atoms form chemical bonds to achieve stability Stable octets may be achieved by electron transfer (M→NM / ionic) or electron sharing (NM-NM / covalent) IONIC BOND: The electrostatic attraction between cations (+) and anions (-) in a crystal lattice. COVALENT BOND: The mutual attraction of shared valence electrons between atomic nuclei in a molecule or complex ion. LEWIS THEORY & QUANTUM MECHANICS Consider the following elements: Na Al P Cl Family 1 13 15 17 Number of Valence Electrons 1 3 5 7 #ve- Therefore, for representative elements, the number of valence electrons equals the one’s digit of the family member. LINK BETWEEN ELECTRON DOT DIAGRAMS AND ORBITAL DIAGRAMS Na Al P Cl...... Electron Aℓ..P.. Cℓ.. dot diagram Na.... Orbital Diagram REPRESENTING MOLECULES In addition to representing individual atoms, ELECTRON DOT DIAGRAMS can also be used to illustrate the covalent bonding between atoms in molecules. To do this, we follow the OCTET RULE: Bonded, non-metallic atoms possess or share a total of eight valence electrons. Electron pairs drawn between element symbols are called SHARED PAIRS or BONDING PAIRS. One shared pair = “single bond” eg. H-H Two shared pairs = “double bond” eg. O=O _ Three shared pairs = “triple bond” eg. N=N Electron pairs drawn next to only one symbol are called LONE PAIRS or NON- BONDING PAIRS. DRAWING ELECTRON DOT DIAGRAMS FOR MOLECULES METHOD 1: BONDING CAPACITY BONDING CAPACITY, or VALENCE is the number of electrons an atom is capable of losing, gaining, or sharing. It is the number of bonds that an atom is able to form. Number of Element Valence Bonding Capacity Electrons O 6 2 C 4 4 N 5 3 Cℓ 7 1 H 1 1 Knowing the information above can help you determine how the atoms are connected, and how they share electrons to achieve a stable octet. DRAWING ELECTRON DOT DIAGRAMS FOR MOLECULES METHOD 1: BONDING CAPACITY Example 1: Example 2: Example 3: CCℓ4 NH3 H2 O BC: 4 1 BC: 3 1 BC: 1 2 * * * central atom DRAWING ELECTRON DOT DIAGRAMS FOR MOLECULES METHOD 2: SYSTEMATIC METHOD 1. How many of each atom is present in the molecule? use molecular formula 2. How are the atoms connected? use bonding capacity 3. How many dots should appear in the diagram? total valence e- count 4. Where should the dots be placed on the diagram? place bonding pairs first, then place lone pairs for octets DRAWING ELECTRON DOT DIAGRAMS FOR MOLECULES METHOD 2: SYSTEMATIC METHOD Example 1: Ammonium Ion, NH4 + 1. How many of each atom is present in the molecule? use molecular formula: 1 N, 4 H 2. How are the atoms connected? use bonding capacity N: BC3, H: BC1 * central atom =N 3. How many electrons should appear in the diagram? total valence e- count ve- = 5 + 4(1) – 1 = 8e- 4. Where should the dots be placed on the diagram? coordinate place bonding pairs first, then place lone pairs for covalent octets bond DRAWING ELECTRON DOT DIAGRAMS FOR MOLECULES METHOD 2: SYSTEMATIC METHOD Example 2: Oxygen gas, O2 1. How many of each atom is present in the molecule? use molecular formula: 2 O 2. How are the atoms connected? use bonding capacity O: BC2 3. How many electrons should appear in the diagram? total valence e- count ve- = 2(6) = 12e- 4. Where should the dots be placed on the diagram? place bonding pairs first, then place lone pairs for octets DRAWING ELECTRON DOT DIAGRAMS FOR MOLECULES METHOD 2: SYSTEMATIC METHOD Example 2: Nitrogen gas, N2 1. How many of each atom is present in the molecule? use molecular formula: 2 N 2. How are the atoms connected? use bonding capacity N: BC3 3. How many electrons should appear in the diagram? total valence e- count ve- = 2(5) = 10e- 4. Where should the dots be placed on the diagram? place bonding pairs first, then place lone pairs for octets TYPES OF REPRESENTATIONS OF MOLECULES ELECTRON DOT DIAGRAMS: For convenience, bonding electron pairs can be represented by lines instead of dots in a LEWIS STRUCTURE. If lone e- pairs are omitted from a Lewis structure, we have drawn the STRUCTURAL FORMULA. CONSIDER THE LEWIS STRUCTURE OF THE PHOSPHATE POLYATOMIC ION # ve = 5 + 4(6) + 3 = 32 Predicted: Actual: RESONANCE: PHOSPHATE POLYATOMIC ION Orbital Diagrams & Electron Configurations Harris & Papaiconomou SCH4U – Structure & Properties Orbital Diagrams or “Energy Level Diagrams” An orbital diagram is a representation that shows the relative energies of electrons in orbitals under normal conditions (i.e. ground state). All four quantum numbers are represented. They give important clues about chemical properties and patterns in the periodic table. (p. 187 Figure 2) Aufbau Diagram (p.188, Figure 5) Electrons fill the lowest energy levels first, and then fill energy levels higher up. as n, E s