DNA Replication - Molecular Biology I (BOI131) - Fall 2023-2024 - PDF
Document Details
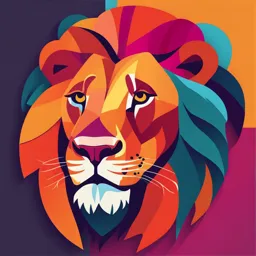
Uploaded by SelfSufficiencyPearTree4414
Galala University
Heba Hamdy Abouseada
Tags
Summary
This document provides an overview of DNA replication, covering fundamental concepts. Key details such as the Watson-Crick model, components, and the process of replication are explained.
Full Transcript
DNA Replication Molecular Biotechnology Program Molecular Biology I (BOI131) Fall 2023-2024 The Future Starts Here GALALA UNIVERSITY THE FUTURE STARTS HERE Faculty of Basic Science Molecular Biotechnology Program Molecular Biology (BIO321)...
DNA Replication Molecular Biotechnology Program Molecular Biology I (BOI131) Fall 2023-2024 The Future Starts Here GALALA UNIVERSITY THE FUTURE STARTS HERE Faculty of Basic Science Molecular Biotechnology Program Molecular Biology (BIO321) Lecture 1 DNA Replication Assoc. Prof. Dr. Heba Hamdy Abouseada [email protected] 2 Gene: is specific sequence of DNA that generally encodes for a certain protein (the DNA sequence must be transcribed in mRNA, which is then translated into the protein). DNA: is genetic material, existing as a double helix of paired complementary base pairs of adenine, thymine, cytosine, and guanine (A-T, C-G) with a sugar-phosphate backbone and a nucleotide center. Chromatin: is a complex of DNA and the proteins necessary for chromosomal organization Nucleosome: The most basic unit of chromatin packaging, which the double stranded DNA wrapped twice around a histone core connected to a neighboring nucleosome via linker (hence, it looks like a bead on a string). Chromosomes: A packaged and organized thread-like structure of tightly coiled DNA around histone proteins (nucleosomes) found in the nucleus of a living cell. Chromatid – homologous chromosomes?? So, the chromatin is a lower order of DNA organization, while chromosomes are the higher order of DNA organization. An organism’s genetic content is counted in terms of the chromosome pairs present. e.g. humans have 23 pairs of chromosomes. Watson and Crick model of DNA DNA stands for deoxy ribonucleotide nucleic acid. The currently accepted model according to the double helix structure proposed by Watson and Crick in which: 1. The two strands that made up the double helix are complementary and anti-parallel in nature. 2. Deoxyribose sugars and phosphates form the backbone of the structure, 3. The nitrogenous bases are stacked inside. 4. The diameter of the double helix, 2 nm, is uniform throughout. 5. A purine always pairs with a pyrimidine; A pairs with T, and G pairs with C. 6. One turn of the helix has ten base pairs. The chemical structure of a polynucleotide chain The difference in chemical structure between DNA and RNA Each nucleotide is composed of a nitrogen- containing nucleobaseeither cytosine (C), guanine (G), adenine (A), or thymine (T), as well as a monosaccharide sugar called deoxyribose and a phosphate group. The nucleotides are joined to one another in a chain by covalent bonds between the sugar of one nucleotide and the phosphate of the next, resulting in an alternating sugar- phosphate backbone. According to base pairing rules (A with T, and C with G), hydrogen bonds bind the nitrogenous bases of the two separate polynucleotide strands to make double-stranded DNA. The two strands of DNA run in opposite directions to each other and are therefore anti-parallel. Attached to each sugar is one of four types of nucleobases (informally, bases). It is the sequence of these four nucleobases along the backbone that encodes biological information. Under the genetic code, RNA strands are translated to specify the sequence of amino acids within proteins. These RNA strands are initially created using DNA strands as a template in a process called transcription. Within cells, DNA is organized into long structures called chromosomes. During cell division these chromosomes are duplicated in the process ofDNA replication, providing each cell its own complete set of chromosomes Eukaryotic organisms (animals, plants, fungi, and protists) store most of their DNA inside the cell nucleus and some of their DNA in organelles, as mitochondria or chloroplasts. In contrast, prokaryotes(bacteria and archaea) store their DNA only in the cytoplasm. DNA Replication Alternative models of DNA replication DNA replication is semi-conservative and occurs during the S-phase of interphase Four components are required: 1. dNTPs: dATP, dTTP, dGTP, dCTP (deoxyribonucleoside 5 ’ - triphosphates) (sugar-base + 3 phosphates) 2. DNA template 3. DNA polymerase (Kornberg enzyme) 4. Mg 2+ (optimizes DNA polymerase activity) DNA Replication Main Features DNA replication involves three main steps: 1-Initiation 2-Elongation 3- Termination The DNA must be accessible to the proteins and enzymes involved in the replication process. Replication starts at specific chromosomal locations called origins of replication (Ori C). DNA replication is semi-conservative and bidirectional. Direction of synthesis is 5’ to 3’ as All DNA polymerases make DNA in 5’- 3’ direction Segments of single-stranded DNA are called template strands. Step 1: Initiation 1.Gyrase enzyme: (a type of topoisomerase) relaxes the supercoiled DNA, to make it accessible to the proteins and enzymes involved in the replication process. 2.Initiator proteins: recognizes the Ori C and binds to its DNA to “recruit” the other proteins. 3.DNA helicase: binds to the DNA double helix at the Ori C and untwist (denature) it using energy derived from ATP (adenosine triphosphate). As the DNA opens up, Y-shaped structures called replication forks are formed. Because two helicases bind, two replication forks are formed at the origin of replication; these are extended in both directions (bidirectional) as replication proceeds creating a replication bubble. 4. SSB (Single-strand binding proteins): stabilize the exposed single stranded DNA to protect it from breakage and prevent it from winding (renaturing) again. 5.Primase enzyme (RNA polymerase): binds to the DNA and synthesizes a short RNA primer (10-12 nucleotide) Step 1: Initiation Step 2: Elongation 6. DNA polymerase III: extends the RNA primer made by primase Leading strand synthesis On the template strand with 3’-5’ orientation, one primer is synthesized and new DNA is made continuously in 5’-3’ direction towards the replication fork. The new strand that is continuously synthesized in 5’-3’ direction is the leading strand. Step 2: Elongation Lagging strand synthesis On the template strand with 5’-3’ orientation, multiple primers are synthesized at specific sites by primase (primosome complex) and DNA pol III synthesizes short pieces of new DNA (about 1000 nucleotides long) new DNA is in 5’-3’ direction. These small DNA fragments that are discontinuously synthesized are called Okazaki fragments. DNA polymerase III synthesizes DNA for both leading and lagging strands. Step 2: Elongation Supercoiled DNA relaxed by gyrase & unwound by helicase + proteins: 5’ SSB Proteins Okazaki Fragments 1 ATP Polymerase III 2 Helicase Lagging strand 3 + Initiator Proteins 3’ primase base pairs Polymerase III 5’ RNA primer replaced by polymerase I & gap is sealed by ligase Leading strand RNA Primer 3’ Step 2: Elongation 7. DNA Polymerase I: uses its exonuclease activity to remove the RNA primer and fills the gaps with new DNA (After DNA synthesis by DNA pol III, DNA ). 8. Finally DNA ligase: joins the ends of the DNA fragments together. DNA ligase seals the gaps between Okazaki fragments with a phosphodiester bond Step 3: Termination 9. The two replication forks meet ~180 degree opposite to Ori C, as DNA is circular in prokaryotes. 10.Around this region there are several terminator sites which arrest the movement of forks by binding to the tus (Terminus Utilization Substance) gene product (an inhibitor of helicase). 11.Once replication is complete, the two double stranded circular DNA molecules (daughter strands) remain interlinked. Topoisomerase II makes double stranded cuts to unlink these molecules. Step 3: Termination Model of DNA replication Model of DNA replication Concepts and terms to understand: Why are gyrase and helicase required? The difference between a template and a primer? The difference between primase and polymerase? What is a replication fork and how many are there? Why are single-stranded binding (SSB) proteins required? How does synthesis differ on leading strand and lagging strand? Which is continuous and semi-discontinuous? What are Okazaki fragments? How do polymerase I and III differ? Assignment The mechanism of DNA replication in eukaryotes is same as that of prokaryotes with some differences, state them. To be handled out and brought with a dead line 17-10-2023. Molecular biology 1 Polymerase chain reaction Course instructor: Dr. Aya Helal (Ph.D) Lecturer of Clinical Biochemistry and Molecular Biology The polymerase chain reaction (PCR) It is a laboratory nucleic acid amplification technique used to denature and renature short segments of deoxyribonucleic acid (DNA) or ribonucleic acid (RNA) sequences using DNA polymerase I enzyme Components of PCR reaction Master Mix Components of PCR reaction Components of PCR reaction Difference between polymerases used in PCR Pfu and vent DNA polymerase has superior thermostability and proofreading properties compared with Taq DNA polymerase. Unlike Taq DNA polymerase, Pfu DNA polymerase possesses 3' to 5' exonuclease proofreading activity, meaning that as the DNA is assembled from the 5' end to 3' end, the exonuclease activity immediately removes nucleotides mis incorporated at the 3' end of the growing DNA strand. Consequently, Pfu DNA polymerase-generated PCR fragments will have fewer errors than Taq-generated PCR inserts. Primers Steps of PCR reaction Steps of PCR reaction Steps of PCR reaction 1 cycle lasts from 45 seconds to 3 minutes 25-30 cycles of amplification are recommended After 20-40 cycles millions of copies of genes are formed Steps of PCR reaction Steps of PCR reaction Conventional PCR Manual PCR, also referred to as conventional PCR, is time consuming and requires manual manipulation. Each step of the protocol involves multiple transfers of sample, reagent, and consumables i.e. pipette tips, tubes, gloves, etc. Once the PCR product is obtained, secondary analysis, such as a gel electrophoresis, is needed for detection. Definitive identification often requires genomic sequence analysis or other alternative methods. Thus, conventional PCR is not ideal for rapid diagnostic use in most clinical laboratories. Real-time PCR (q-PCR) Real-time PCR, also called quantitative PCR (qPCR), is an automated technique that allows for detection and quantification of the target material. Results are generated at the same time that the assay is being run, hence the designation "Real-time." The key feature in RT-PCR is that amplification of DNA is detected in “real time” as the PCR is in progress. The assessment of the PCR progression is performed using a “fluorescent reporter” which measures the fluorescent signal to directly assess the number of amplified DNA molecules. Real-time PCR (q-PCR) SYBR Green This is a dye that emits prominent fluorescent signal when it binds at the minor groove of DNA, nonspecifically. Other fluorescent dyes like Ethidium Bromide or Acridine Orange can also be used but SYBR Green is better used for its higher signal intensity. SYBR Green can provide information about each cycle of amplification Real-time PCR (q-PCR) Threshold: The level that the signal reflect statistically significant increase over baseline signal CT: the number of cycles required for amplification to reach threshold RT-PCR Reverse transcription polymerase chain reaction (RT-PCR) is a laboratory technique combining reverse transcription of RNA into DNA (in this context called complementary DNA or cDNA) and amplification of specific DNA targets using polymerase chain reaction (PCR) It is primarily used to measure the amount of a specific RNA. This is achieved by monitoring the amplification reaction using fluorescence, a technique called real-time PCR or quantitative PCR (qPCR). Advantages of PCR technology 1. Highly specific: PCR can distinguish DNA sequences by just one nucleotide, making it a very accurate technique. 2. Sensitive: PCR is a very useful technique when the amount of DNA sample is limited because it allows the detection of even a single copy of a specific DNA template. 3. Versatile: The PCR technique can be used for various applications like genetic testing, criminal investigations, and paternity tests. 4. Rapid and efficient: PCR can efficiently and rapidly amplify a small amount of DNA sample to million copies in just a few hours. Disadvantages of PCR technology 1. Contamination: The PCR technique is very susceptible to contamination from other sources of DNA or RNA or the environment. This can mislead data interpretation. 2. Cost and complexity: PCR can be expensive and requires expert knowledge for high-throughput projects. 3. Lack of novel information: Since PCR can only amplify and target specific DNA sequences targeted by the primers, PCR provides limited information and cannot detect novel DNA sequences. 4. Inhibition from sample content: The whole PCR cycle can be disrupted by inhibitors that co-purify with DNA, such as heme from blood samples, reducing the sensitivity of the process. 5. Errors in amplification: Base substitutions, indels, and other alterations in DNA sequences can lead to inaccurate amplification and hence, false results. Transcription in Prokaryotes Prokaryotes gene structure 1. Promoter: a sequence upstream of the gene transcription start site +1 with which the RNA interacts to define the direction for transcription and thus dictates to the enzyme which DNA strand is the template and where transcription is to begin. Two consensus sequences are said to be found in most of the prokaryotic genes promoters -35 box and -10 box. 2. RNA-coding sequence : the DNA that is transcriped into RNA 3. Terminator: a sequence downstream of the gene, specifies where transcription stops Prokaryotic RNA polymerases ❑In E. coli, the RNA polymerase holoenzyme is composed of: 1. Core enzyme (Four subunits) = 2α + β + β’ 2. Sigma factor One subunit =s Initiation. 1. At the start of initiation, the RNA polymerase holoenzyme binds loosely to the DNA. 2. It then scans along the DNA, until it encounters a promoter 3. When it does, the sigma factor recognizes both the –35 and –10 regions. 4. A region within the sigma factor that contains a helix-turn-helix structure then interacts strongly with the promoter, causing RNA polymerase to “tighten its grip” on the DNA. 5. The tight binding of the RNA polymerase to the promoter forms what is called the closed complex 6. Then, the open complex is formed when RNA polymerase denatures the double-stranded DNA in the AT-rich Pribnow (-10) Box 7. Next, the RNA polymerase makes a short RNA strand copy of the template strand within the denatured region The sigma factor is released at this point This marks the end of initiation Note that RNA polymerase, unlike DNA polymerase, is a “smart enzyme”! It can start an RNA strand all on its own. Initiation Elongation Similar to the synthesis of DNA via DNA polymerase Termination Termination is the end of RNA synthesis. It occurs when the short RNA-DNA hybrid of the open complex is forced to separate. This releases the newly made RNA as well as the RNA polymerase. E. coli has two different mechanisms for termination 1. Rho-dependent termination *Requires a protein known as r (rho) 2. Rho-independent termination *Does not require r (rho) Termination The termination of bacterial gene transcription is signaled by terminator sequences. In E. coli, the protein Rho ( r ) plays a role in the termination of transcription of some genes. The terminators of such genes are called Rho dependent terminators (also, type II terminators). For other genes, the core RNA polymerase terminates transcription; terminators for those genes are called Rho independent terminators (also, type I terminators). Termination 1. Rho-independent termination Rho-independent terminators consist of an inverted repeat sequence that is about 16 to 20 base pairs upstream of the transcription termination point, followed by a string of about 4 to 8 A–T base pairs. The RNA polymerase transcribes the terminator sequence, which is part of the initial RNA coding sequence of the gene. Because of the inverted repeat arrangement, the RNA folds into a hairpin loop structure. The hairpin structure causes the RNA polymerase to slow and then pause in its catalysis of RNA synthesis. The string of U nucleotides downstream of the hairpin destabilizes the pairing between the new RNA chain and the DNA template strand, and RNA polymerase dissociates from the template; transcription has terminated. Termination 1. Rho-independent termination Termination 1. Rho-independent termination Termination 2. Rho-dependent termination Rho-dependent terminators are C-rich, G-poor sequences that have no hairpin structures like those of rho-independent terminators. Termination at these terminators is as follows: Rho binds to the C- rich terminator sequence in the transcript upstream of the transcription termination site. Rho then moves along the transcript until it reaches the RNA polymerase, where the most recently synthesized RNA is base paired with the template DNA. Rho is a helicase enzyme, meaning that it can unwind double- stranded nucleic acids. Termination 2. Rho-dependent termination When Rho reaches the RNA polymerase, helicase unwinds the helix formed between the RNA and the DNA template strand, using ATP hydrolysis to provide the needed energy. The new RNA strand is then released, the DNA double helix reforms, and the RNA polymerase and Rho dissociate from the DNA; transcription has terminated. Molecular biology 1 (Gene transcription and processing) Course instructor: Dr. Aya Helal (Ph.D) Lecturer of Clinical Biochemistry and Molecular Biology RNA RNA plays a central role in the life of the cell. We are mostly going to look at its role in protein synthesis, but RNA does many other things as well. RNA can both store information (like DNA) and catalyze chemical reactions (like proteins). RNA/protein hybrid structures are involved in protein synthesis (ribosome) and splicing of messenger RNA. Major players in transcription mRNA- type of RNA that encodes information for the synthesis of proteins and carries it to a ribosome from the nucleus Reading the DNA code Every 3 DNA bases pairs with 3 mRNA bases Every group of 3 mRNA bases encodes a single amino acid Codon- coding triplet of mRNA bases Transfer RNA (tRNA). Small RNA molecules that act as adapters between the codons of messenger RNA and the amino acids they code for. Ribosomal RNA (rRNA). Four different RNA molecules that make up part of the structure of the ribosome. They perform the actual catalysis of adding an amino acid to a growing peptide chain. What is transcription and translation? Transcription vs. Translation Transcription Translation Process by which genetic Process by which information encoded in information encoded in DNA is copied onto mRNA is used to messenger RNA assemble a protein at a Occurs in the nucleus ribosome DNA mRNA Occurs on a Ribosome mRNA protein Transcription Transcription is the process by which an RNA sequence is produced from a DNA template: RNA polymerase is the enzyme responsible for making mRNA copies of genes. DNA unzips at the site of the gene that is needed. Initiation RNA polymerase complex of enzymes with 2 functions: ⚫ Unwind DNA sequence ⚫ Produce primary transcript by stringing together the chain of RNA nucleotides Initiation A promoter is a region of DNA upstream of a gene where relevant proteins (such as RNA polymerase and transcription factors) bind to initiate transcription of that gene. The resulting transcription produces an RNA molecule (such as mRNA). Initiation of Transcription in Eukaryotes Unlike the prokaryotic RNA polymerase that can bind to a DNA template on its own, eukaryotes require several other proteins, called transcription factors, to first bind to the promoter region and then help recruit the appropriate polymerase. The completed assembly of transcription factors and RNA polymerase bind to the promoter, forming a transcription pre- initiation complex (PIC). Transcription factor (TF) Protein that controls the rate of transcription of genetic information from DNA to messenger RNA, by binding to a specific DNA sequence. The function of TFs is to regulate—turn on and off— genes in order to make sure that they are expressed in the desired cells at the right time and in the right amount throughout the life of the cell and the organism. Transcription factor (TF) The most-extensively studied core promoter element in eukaryotes is a short DNA sequence known as a TATA box, found 25-30 base pairs upstream from the start site of transcription. The TATA box, as a core promoter element, is the binding site for a transcription factor known as TATA-binding protein (TBP), which is itself a subunit of another transcription factor: Transcription Factor II D (TFIID). After TFIID binds to the TATA box via the TBP, five more transcription factors and RNA polymerase combine around the TATA box in a series of stages to form a pre-initiation complex. One transcription factor, Transcription Factor II H (TFIIH), is involved in separating opposing strands of double-stranded DNA to provide the RNA Polymerase access to a single-stranded DNA template. Eukaryotic Transcription Initiation Three Eukaryotic RNA Polymerases (RNAPs) The features of eukaryotic mRNA synthesis are markedly more complex those of prokaryotes. Instead of a single polymerase comprising five subunits, the eukaryotes have three polymerases that are each made up of 10 subunits or more. Each eukaryotic polymerase also requires a distinct set of transcription factors to bring it to the DNA template. Three Eukaryotic RNA Polymerases (RNAPs) RNA polymerase I is located in the nucleolus, a specialized nuclear substructure in which ribosomal RNA (rRNA) is transcribed, processed, and assembled into ribosomes. The rRNA molecules are considered structural RNAs because they have a cellular role but are not translated into protein Three Eukaryotic RNA Polymerases (RNAPs) RNA polymerase II is located in the nucleus and synthesizes all protein-coding nuclear pre-mRNAs. Eukaryotic pre-mRNAs undergo extensive processing after transcription, but before translation. RNA polymerase II is responsible for transcribing the overwhelming majority of eukaryotic genes, including all of the protein-encoding genes which ultimately are translated into proteins and genes for several types of regulatory RNAs, including microRNAs (miRNAs). Three Eukaryotic RNA Polymerases (RNAPs) RNA polymerase III is also located in the nucleus. This polymerase transcribes a variety of structural RNAs that includes the 5S pre-rRNA, transfer pre-RNAs (pre-tRNAs), and small nuclear pre-RNAs. The tRNAs have a critical role in translation: they serve as the adaptor molecules between the mRNA template and the growing polypeptide chain. Small nuclear RNAs have a variety of functions, including “splicing” pre-mRNAs and regulating transcription factors. Not all miRNAs are transcribed by RNA Polymerase II, RNA Polymerase III transcribes some of them. Elongation Notice that the RNA is made from 5’ end to 3’ end, so the coding strand is actually read from 3’ to 5’. RNA polymerase proceeds down the DNA, synthesizing the RNA copy. Elongation The enzyme RNA polymerase binds to a site on the DNA at the start of a gene (The sequence of DNA that is transcribed into RNA is called a gene). RNA polymerase separates the DNA strands and synthesises a complementary RNA copy from the antisense DNA strand It does this by covalently bonding ribonucleoside triphosphates by using the energy Termination Transcription stops when the RNA polymerase reaches a specific termination signal on the DNA. This signal instructs the polymerase to release the newly formed RNA molecule. The DNA double helix reforms, and the RNA product is freed. Once the RNA sequence has been synthesized: - RNA polymerase will detach from the DNA molecule - RNA detaches from the DNA - the double helix reforms Transcription occurs in the nucleus (where the DNA is) and, once made, the mRNA moves to the cytoplasm (where translation can occur) RNA splicing RNA splicing is a process where a newly-made precursor messenger RNA (pre- mRNA) transcript is transformed into a mature messenger RNA (mRNA). RNA splicing RNA splicing is a process where a newly-made precursor messenger RNA (pre- mRNA) transcript is transformed into a mature messenger RNA (mRNA). Introns are regions within a gene that don’t code for protein and don’t appear in the final mRNA molecule. Protein-coding sections of a gene (called exons) are interrupted by introns. RNA splicing It works by removing all the introns (non-coding regions of RNA) and splicing back together exons (coding regions). For eukaryotic genes , splicing occurs in the nucleus either during or immediately after transcription to create an mRNA molecule that can be translated into protein. Introns splicing occurs in a series of reactions which are catalyzed by the spliceosome, a complex of small nuclear ribonucleoproteins (snRNPs) Steps of RNA processing In eukaryotes, RNA polymerase produces a “primary transcript”, an exact RNA copy of the gene. A cap is put on the 5’ end (7-methyl guanine). All introns are spliced out. The RNA is terminated and poly-A tail is added to the 3’ end (AAAAAAA). At this point, the RNA can be called messenger RNA. It is then transported out of the nucleus into the cytoplasm, where it is translated.. Molecular biology 1 (Gene transcription and processing) Course instructor: Dr. Aya Helal (Ph.D) Lecturer of Clinical Biochemistry and Molecular Biology RNA RNA plays a central role in the life of the cell. We are mostly going to look at its role in protein synthesis, but RNA does many other things as well. RNA can both store information (like DNA) and catalyze chemical reactions (like proteins). RNA/protein hybrid structures are involved in protein synthesis (ribosome) and splicing of messenger RNA. Major players in transcription mRNA- type of RNA that encodes information for the synthesis of proteins and carries it to a ribosome from the nucleus Reading the DNA code Every 3 DNA bases pairs with 3 mRNA bases Every group of 3 mRNA bases encodes a single amino acid Codon- coding triplet of mRNA bases Transfer RNA (tRNA). Small RNA molecules that act as adapters between the codons of messenger RNA and the amino acids they code for. Ribosomal RNA (rRNA). Four different RNA molecules that make up part of the structure of the ribosome. They perform the actual catalysis of adding an amino acid to a growing peptide chain. What is transcription and translation? Transcription vs. Translation Transcription Translation Process by which genetic Process by which information encoded in information encoded in DNA is copied onto mRNA is used to messenger RNA assemble a protein at a Occurs in the nucleus ribosome DNA mRNA Occurs on a Ribosome mRNA protein Transcription Transcription is the process by which an RNA sequence is produced from a DNA template: RNA polymerase is the enzyme responsible for making mRNA copies of genes. DNA unzips at the site of the gene that is needed. Initiation RNA polymerase complex of enzymes with 2 functions: ⚫ Unwind DNA sequence ⚫ Produce primary transcript by stringing together the chain of RNA nucleotides Initiation A promoter is a region of DNA upstream of a gene where relevant proteins (such as RNA polymerase and transcription factors) bind to initiate transcription of that gene. The resulting transcription produces an RNA molecule (such as mRNA). Initiation of Transcription in Eukaryotes Unlike the prokaryotic RNA polymerase that can bind to a DNA template on its own, eukaryotes require several other proteins, called transcription factors, to first bind to the promoter region and then help recruit the appropriate polymerase. The completed assembly of transcription factors and RNA polymerase bind to the promoter, forming a transcription pre- initiation complex (PIC). Transcription factor (TF) Protein that controls the rate of transcription of genetic information from DNA to messenger RNA, by binding to a specific DNA sequence. The function of TFs is to regulate—turn on and off— genes in order to make sure that they are expressed in the desired cells at the right time and in the right amount throughout the life of the cell and the organism. Transcription factor (TF) The most-extensively studied core promoter element in eukaryotes is a short DNA sequence known as a TATA box, found 25-30 base pairs upstream from the start site of transcription. The TATA box, as a core promoter element, is the binding site for a transcription factor known as TATA-binding protein (TBP), which is itself a subunit of another transcription factor: Transcription Factor II D (TFIID). After TFIID binds to the TATA box via the TBP, five more transcription factors and RNA polymerase combine around the TATA box in a series of stages to form a pre-initiation complex. One transcription factor, Transcription Factor II H (TFIIH), is involved in separating opposing strands of double-stranded DNA to provide the RNA Polymerase access to a single-stranded DNA template. Eukaryotic Transcription Initiation Three Eukaryotic RNA Polymerases (RNAPs) The features of eukaryotic mRNA synthesis are markedly more complex those of prokaryotes. Instead of a single polymerase comprising five subunits, the eukaryotes have three polymerases that are each made up of 10 subunits or more. Each eukaryotic polymerase also requires a distinct set of transcription factors to bring it to the DNA template. Three Eukaryotic RNA Polymerases (RNAPs) RNA polymerase I is located in the nucleolus, a specialized nuclear substructure in which ribosomal RNA (rRNA) is transcribed, processed, and assembled into ribosomes. The rRNA molecules are considered structural RNAs because they have a cellular role but are not translated into protein Three Eukaryotic RNA Polymerases (RNAPs) RNA polymerase II is located in the nucleus and synthesizes all protein-coding nuclear pre-mRNAs. Eukaryotic pre-mRNAs undergo extensive processing after transcription, but before translation. RNA polymerase II is responsible for transcribing the overwhelming majority of eukaryotic genes, including all of the protein-encoding genes which ultimately are translated into proteins and genes for several types of regulatory RNAs, including microRNAs (miRNAs). Three Eukaryotic RNA Polymerases (RNAPs) RNA polymerase III is also located in the nucleus. This polymerase transcribes a variety of structural RNAs that includes the 5S pre-rRNA, transfer pre-RNAs (pre-tRNAs), and small nuclear pre-RNAs. The tRNAs have a critical role in translation: they serve as the adaptor molecules between the mRNA template and the growing polypeptide chain. Small nuclear RNAs have a variety of functions, including “splicing” pre-mRNAs and regulating transcription factors. Not all miRNAs are transcribed by RNA Polymerase II, RNA Polymerase III transcribes some of them. Elongation Notice that the RNA is made from 5’ end to 3’ end, so the coding strand is actually read from 3’ to 5’. RNA polymerase proceeds down the DNA, synthesizing the RNA copy. Elongation The enzyme RNA polymerase binds to a site on the DNA at the start of a gene (The sequence of DNA that is transcribed into RNA is called a gene). RNA polymerase separates the DNA strands and synthesises a complementary RNA copy from the antisense DNA strand It does this by covalently bonding ribonucleoside triphosphates by using the energy Termination Transcription stops when the RNA polymerase reaches a specific termination signal on the DNA. This signal instructs the polymerase to release the newly formed RNA molecule. The DNA double helix reforms, and the RNA product is freed. Once the RNA sequence has been synthesized: - RNA polymerase will detach from the DNA molecule - RNA detaches from the DNA - the double helix reforms Transcription occurs in the nucleus (where the DNA is) and, once made, the mRNA moves to the cytoplasm (where translation can occur) RNA splicing RNA splicing is a process where a newly-made precursor messenger RNA (pre- mRNA) transcript is transformed into a mature messenger RNA (mRNA). RNA splicing RNA splicing is a process where a newly-made precursor messenger RNA (pre- mRNA) transcript is transformed into a mature messenger RNA (mRNA). Introns are regions within a gene that don’t code for protein and don’t appear in the final mRNA molecule. Protein-coding sections of a gene (called exons) are interrupted by introns. RNA splicing It works by removing all the introns (non-coding regions of RNA) and splicing back together exons (coding regions). For eukaryotic genes , splicing occurs in the nucleus either during or immediately after transcription to create an mRNA molecule that can be translated into protein. Introns splicing occurs in a series of reactions which are catalyzed by the spliceosome, a complex of small nuclear ribonucleoproteins (snRNPs) Steps of RNA processing In eukaryotes, RNA polymerase produces a “primary transcript”, an exact RNA copy of the gene. A cap is put on the 5’ end (7-methyl guanine). All introns are spliced out. The RNA is terminated and poly-A tail is added to the 3’ end (AAAAAAA). At this point, the RNA can be called messenger RNA. It is then transported out of the nucleus into the cytoplasm, where it is translated.. Regulation of Gene Expression ONE OF THE BEST STRATEGIES FOR AN organism’s survival is to be able to adapt quickly to changes in its environment. In fact, a fundamental property of living cells is their ability to turn their genes on and off in response to extracellular signals. This control of gene expression makes it possible for cells to produce specific kinds of proteins when and where they are needed. Regulated genes Genes whose activity is controlled in response to the needs of a cell or organism. Constitutive genes or housekeeping genes Genes whose products are essential to the normal functioning of a growing and dividing cell, no matter what the conditions are. These genes are always active in growing cells; examples include genes that code for the enzymes needed for protein synthesis and glucose metabolism. Regulation of Gene Expression in Bacteria Significantly, genes which encode proteins that work together in the cell typically are organized into OPERONS; that is, the genes are adjacent to each other and are transcribed together onto a polycistronic mRNA, so called because it contains the information from more than one gene. Regulation of the synthesis of this mRNA depends on interactions between regulatory proteins and regulatory sequences that are next to the gene array. Structure of the polycistronic mRNA encoded by the three clustered lac utilization genes in E. coli and its translation to produce β-galactosidase, permease, and transacetylase. When gene expression is turned on in a bacterium by adding a substance (such as lactose) to the medium, the genes involved are said to be ➔ INDUCIBLE. The regulatory substance that brings about this gene induction is called an ➔ INDUCER. And the phenomenon of producing a gene product in response to an inducer is called ➔ INDUCTION. The inducer is an example of a class of small molecules, called effectors or effector molecules, that help control the expression of many regulated genes. An inducible gene is transcribed in response to a regulatory event occurring at a specific regulatory DNA sequence adjacent to or near the protein-coding sequence. The regulatory event typically involves an inducer and a regulator protein; and when it occurs, RNA polymerase initiates transcription at the promoter (usually upstream of the regulatory sequence). The gene is turned on, mRNA is made, and the protein encoded by the gene is produced. The regulatory sequence itself does not code for any product The lac Operon of E. coli E. coli can grow in a simple medium containing salts(including a nitrogen source) and a carbon source such as glucose. The energy for biochemical reactions in the cell comes from glucose metabolism. The enzymes required for glucose metabolism are coded for by constitutive genes. If lactose is provided to E. coli as a carbon source instead of glucose, a number of enzymes that are required to metabolize lactose are rapidly synthesized. (A similar series of events, each involving a sugar-specific set of enzymes, is triggered by other sugars as well.) The lac Operon of E. coli (cont.) The enzymes are synthesized because the genes that code for them become actively transcribed in the presence of the sugar; the same genes are inactive if the sugar is absent. In other words, the genes are regulated genes whose products are needed only under certain conditions. Lactose is a disaccharide consisting of the monosaccharides D-galactose and D-glucose. The lac Operon of E. coli (cont.) When lactose is present as the sole carbon source in the growth medium, three proteins are synthesized: 1.β-Galactosidase It breaks down lactose into galactose and glucose. catalyzes the isomerization (conversion to a different form) of lactose to allolactose ( a compound that is important in regulating expression of the lac operon) (In the cell, the galactose is converted to glucose by enzymes encoded by a gene system specific to galactose catabolism. The glucose is then utilized by constitutively produced enzymes.) The lac Operon of E. coli (cont.) 2. Lactose permease It is found in the E. coli cytoplasmic membrane, actively transports lactose into the cell. 3. β-Galactoside transacetylase. This enzyme transfers an acetyl group from acetyl-CoA to β-galactosidase. The function of this enzyme in the lac operon is not understood. In wild-type E. coli growing in a medium containing glucose, only a low concentration of each of these three proteins is produced. For example, only an average of three molecules of β- galactosidase is present in the cell under these conditions. In the presence of lactose, and the absence of glucose, the amount of each enzyme increases coordinately (simultaneously) about a thousand fold (e.g., to about 3,000 molecules of β-- galactosidase), because the three essentially inactive genes are now actively transcribed. The process is called COORDINATE INDUCTION. Allolactose, not lactose, is the inducer molecule directly responsible for the increased production of the three enzymes. Furthermore, the mRNAs for the enzymes have a short half- life, so the transcripts must be made continually in order for the enzymes to be produced. When lactose is no longer present, transcription of the three genes is stopped and any mRNAs already present are broken down, so no more of these proteins are made. Existing proteins are degraded and diluted out by cell growth and division. Experimental Evidence for the Regulation of lac Genes 1. Mutations in the Protein-Coding Genes. Mutations in the protein-coding (structural) genes were obtained after treatment of cells with chemical mutagens. The mutations were characterized to determine which enzyme activity was affected. The β-galactosidase gene was named lacZ. The permease gene was named lacY. The transacetylase gene was named lacA. The experiments showed that the three genes are tightly linked in the order lacZ–lacY–lacA. The three clustered genes were shown to be transcribed onto a single mRNA molecule—called a polycistronic mRNA—rather than onto three separate mRNAs. That is, RNA polymerase initiates transcription at a single promoter, and a polycistronic mRNA is synthesized with the gene transcripts in the order 5’ -lacZ –lacY –lacA –3’. In translation, a ribosome loads onto the polycistronic mRNA at the 5’ end and synthesizes β-galactosidase; it then reinitiates translation at the permease sequence and synthesizes permease, reinitiates at the transacetylase sequence and synthesizes transacetylase, and finally dissociates from the mRNA. Mutations Affecting the Regulation of Gene Expression. They identified two classes of constitutive mutations: 1. One class mapped to a small region just upstream of the lacZ gene they called the operator (lacO), 2. The other class mapped to a gene upstream of the operator they called the lacI gene or Lac repressor gene. The promoter, operator, and the three structural genes constitute the lac operon. Jacob and Monod’s Operon Model for the Regulation of lac Genes On the basis of their results, Jacob and Monod proposed their now-classic operon model. By definition, an operon is a cluster of genes, the expressions of which are regulated together by operator–repressor protein interactions, plus the operator region itself and the promoter. The order of the controlling elements and genes in the lac operon is promoter– operator–lacZ–lacY–lacA. The regulatory gene lacI is located close to the structural genes, just upstream of the promoter with its own promoter and terminator and encodes the Lac repressor. Negative control of the Lac Operon The promoter for the lacI gene is weak, so few repressor molecules are found in the cell. The Lac repressor binds to the operator (lacO), and covers the DNA sequence recognized by RNA polymerase. Therefore, RNA polymerase cannot bind to the promoter and transcription cannot occur—because of this, the lac operon is said to be under negative control. The low level of gene transcription that produces a few molecules of the enzymes, even in the absence of the inducer, occurs because repressors do not just bind and stay; they bind and dissociate. In the split second after one repressor unbinds and before another binds, an RNA polymerase could initiate transcription of the operon, even in the absence of the inducer. This “leaky” expression generates a few molecules of the three enzymes encoded by the lac operon, which is necessary to allow the initial transportation of lactose into the cell, and the initial conversion of lactose to allolactose when lactose is first added. Functional state of the lac operon in wild-type E. coli growing in the absence of lactose. When wild-type E. coli grows in the presence of lactose as the sole carbon source , some lactose is converted by β-galactosidase into allolactose. Allolactose binds to the Lac repressor and changes its shape; as a result, the repressor loses its affinity for the lac operator and dissociates from the site. Free repressor proteins are also altered by binding to allolactose so that they cannot bind to the operator. In this way, allolactose induces production of the lac operon-encoded enzymes. With no Lac repressor bound to the operator, RNA polymerase initiates synthesis of a single polycistronic mRNA molecule for the lacZ , lacY , and lacA genes. The polycistronic mRNA for the lac operon is translated by a string of ribosomes to produce the three enzymes specified by the operon. Functional state of the lac operon in wild-type E. coli growing in the presence of lactose as the sole carbon source. Positive control of the Lac Operon The Lac repressor protein exerts a negative effect on the expression of the lac operon by blocking RNA polymerase’s binding to the promoter if the inducer is absent. Several years J. and M. and their team have found a positive control system that also regulates the lac operon—a system that functions to turn on the expression of the operon. This system ensures that the lac operon will be expressed at high levels only if lactose is the sole carbon source and not if glucose is present as well. First, a protein called catabolite activator protein (CAP) binds with cAMP (cyclic AMP, or cyclic adenosine 3’, 5’-monophosphate) to form a CAP–cAMP complex ➔ the positive-regulator molecule. Next, the CAP–cAMP complex binds to the CAP site, which is upstream of the site where RNA polymerase binds to the promoter. CAP then recruits RNA polymerase to the promoter, and transcription is initiated. Molecular biology 1 (Chromatin remodeling) Course instructor: Dr. Aya Helal (Ph.D) Lecturer of Clinical Biochemistry and Molecular Biology Chromatin remodeling chromatin remodeling is the enzyme-assisted process to facilitate access of nucleosomal DNA by remodeling the structure, composition and positioning of nucleosomes. Epigenetics modification Epigenetics, the study of the chemical modification of specific genes or gene-associated proteins of an organism resulting in Changing in gene expression without affecting the primary DNA sequence. So, epigenetic modifications regulate the processes during the transition from genotype to phenotype. Epigenetic modification including DNA methylation, histone modification and micro RNAs. Chromatin remodeling DNA is tightly packaged in the nucleus with the help of packaging proteins (histone proteins) to form repeating units of nucleosomes which further bundle together to form condensed chromatin structure. Theses condensed structure occludes many DNA regulatory regions, not allowing them to interact with transcriptional machinery proteins and regulate gene expression. To overcome this issue and allow dynamic access to condensed DNA, a process known as chromatin remodeling alters nucleosome architecture to expose or hide regions of DNA for transcriptional regulation. Chromatin remodeling Chromatin remodeling is the dynamic modification of chromatin architecture to allow access of condensed genomic DNA to the regulatory transcription machinery proteins, and thereby control gene expression. Such remodeling is principally carried out by 1) covalent histone modifications by specific enzymes, e.g., histone acetyltransferases (HATs), deacetylases, methyltransferases, and kinases 2) ATP-dependent chromatin remodeling complexes which either move, eject or restructure nucleosomes. Chromatin remodeling Aberrations in chromatin remodeling proteins are found to be associated with human diseases, including cancer. Targeting chromatin remodeling pathways is currently evolving as a major therapeutic strategy in the treatment of several cancers Histone-modifying complexes Specific protein complexes, known as histone-modifying complexes catalyze addition or removal of various chemical elements on histones. These enzymatic modifications include acetylation, methylation, phosphorylation, and ubiquitination and primarily occur at N-terminal histone tails. Such modifications affect the binding affinity between histones and DNA, leading to loosening or tightening the condensed DNA wrapped around histones Methylation of specific lysine residues in H3 and H4 causes further condensation of DNA around histones, and thereby prevents binding of transcription factors to the DNA that lead to gene repression. Histone acetylation relaxes chromatin condensation and exposes DNA for TF binding, leading to increased gene expression. DNA methylation DNA methylation is an epigenetic modification that occurs as a result of a biological mechanism that leads to the attachment of a methyl group onto the 5th carbon of cytosine (5mC). DNA methylation processes are controlled by the DNA methyltransferase enzyme (DNMTs) family, which create 5 methylcytosine (5mC) via transferring a methyl group from a donor S- adenosyl methionine (SAM) to the 5th carbon of cytosine. A: CH3 is added to cytosine in the presence of DNA according to methylation DNMT enzymes and SAM (donor). B: Symmetrical status is either Hypomethylated CpG methylation. C: Diagram shows relation or hypermethylated. between DNA methylation and gene expression. DNA methylation Hypomethylation is a process that leads to gene activation (oncogenes) and is carried out by two mechanisms. Passive process: loss or reduction of DNMT enzyme during cell division. Active process: ten-eleven translocation (TET) enzyme via an enhanced oxidation mechanism leads to convert methylated cytosine to unmethylated cytosine. Hypermethylated is a process that leads to gene inactivation (Tumor suppressor genes). which suggested an increase of DNMTs enzymes activity in cancer cells compared to normal cells. Histone methylation takes place on lysine or arginine residues, and is regulated DNA methylation image DNA methylation controlling gene expression. (A) The CpG island promoter is unmethylated and allows binding of transcription factors, which is required for transcription initiation. (B) The CpG island promoter methylation deters binding of transcription factors and causing gene inactivation. Histone acetylation and deacetylation : Histone acetyl transferase (HAT): tend to add acetyl group on lysine residue on histone tail, which leads to chromatin becoming permissive and as a consequence a transcription process takes place. Histone deacetylase (HDAC): tend to remove an acetyl group, causing stability of chromatin structure and expression inhibition. Histone acetylation and deacetylation : Acetyl group remove + charge Decrease interaction between histone and negatively charged DNA packed chromatin converts to unpacked chromatin Acetylated histones cannot pack as well together as deacetylated Increase histones. Gene expression Epigenetics The histone code is a hypothesis that the transcription of genetic information encoded in DNA is in part regulated by chemical modifications to histone proteins, primarily on their unstructured ends. Together with similar modifications such as DNA methylation it is part of the epigenetic code ATP-dependent chromatin remodeling Remodelers chromatin remodelers provide the mechanism for modifying chromatin and allowing transcription signals to reach their destinations on the DNA strand. Understanding the nature and processes of these cellular construction workers remains an active area of discovery in genetic research. chromatin remodelers are large, multiprotein complexes that use the energy of ATP hydrolysis to mobilize and restructure nucleosomes Remodelers are necessary to provide access to the underlying DNA to enable transcription, chromatin assembly, DNA repair, and other processes Remodelers convert the energy of ATP hydrolysis into mechanical force to mobilize the nucleosome. Remodelers contain a subunit with a conserved ATPase domain. chromatin remodeler complex is called SWI/SNF ATP-dependent chromatin remodeling Remodelers It seems that the ATPase binds approximately 40 base pairs inside the nucleosome, from which location it pumps DNA around the histone-octamer surface. This enables the movement of the nucleosome along the DNA, thus permitting the exposure of the DNA to regulatory factors. (DNA and RNA polymerases and Transcription Factors) ATP-dependent chromatin remodeling Remodelers Chromatin remodeling complexes in the dynamic regulation of transcription: In the presence of acetylated histones (HAT mediated) and absence of methylase (HMT) activity, chromatin is loosely packaged. Additional nucleosome repositioning by chromatin remodeler complex, SWI/SNF opens up DNA region where transcription machinery proteins, like RNA Pol II, transcription factors and co-activators bind to turn on gene transcription. ATP-dependent chromatin remodeling Remodelers In the absence of SWI/SNF, nucleosomes can not move farther and remain tightly aligned to one another. Additional methylation by HMT and deacetylation by HDAC proteins condenses DNA around histones So, this makes the DNA unavailable for binding by RNA Pol II and other activators, leading to gene silencing. Thank you, my sweeties, LOVE YOU Dr. AYA Molecular biology 1 (DNA Packing) Course instructor: Dr. Aya Helal (Ph.D) Lecturer of Clinical Biochemistry and Molecula Biology The eukaryotic chromosome: The eukaryotic chromosome is composed of both double-stranded DNA and proteins. The combination of DNA and protein is called chromatin. Two types of chromatins are formed: 1) Euchromatin: loosely packaged (less condensed), can be transcribed. 2) Heterochromatin: tightly packaged (highly condensed), not transcribed. Chromatin: The primary function of chromatin is to package DNA into a smaller volume to fit inside the nucleus, which is only 2-4 µm (10-6 m) in diameter. The basic structural unit of chromatin is the nucleosome. Nucleosomes consist of DNA wrapped around the protein core, which is composed of histone protein. The major proteins of chromatin are the histones, basic amino acid (arginine and lysine) that facilitate binding to the negatively charged DNA molecule. Histones Histone, type of protein that plays a critical role in the structural organization and regulation of DNA within the nucleus of eukaryotic cells. Histones were discovered in avian red blood cell nuclei by German biochemist Albrecht Kossel about 1884 Histones Histones are water-soluble and contain large amounts of basic amino acids, particularly lysine and arginine. They combine ionically with DNA to form nucleoprotein complexes known as nucleosomes. Each nucleosome is made up of an octamer of two copies of four different histone proteins, designated H2A, H2B, H3, and H4. Histones Histones, influence the accessibility of DNA to transcription machinery through the formation of chromatin, also and therefore serve an essential role in controlling gene expression. Histones also assist with managing chromatin structure during DNA repair processes and with organizing chromatin during cell division, ensuring correct chromosome segregation. Histones structure The nucleosome core is formed of two H2A-H2B dimers and a H3-H4 tetramer, forming two nearly symmetrical halves by tertiary structure. Chromatin: TEMs of isolated chromatin (A) Negatively stained 30 nm helix (B) Shadowed “string of beads” Beads on the string Chromatin: During mitosis and meiosis, the condensed chromosomes are assembled through interactions between nucleosomes and other regulatory proteins. Nucleosome There are five major types of histones called H1, H2A, H2B, H3 and H4. The structure of nucleosomes have been studied by treating chromatin with DNase, large amount of DNA are degraded, leaving only small particles composed of DNA and proteins. The DNA in these small DNA-protein complexes consists of fragments of about 210 bp. This DNA is protected from DNase digestion due to its tight association with the proteins. Chromatin structure during cell division In interphase, eukaryotic chromosomes have two distinct regions that can be distinguished by staining. Heterochromatin usually contains genes that are not expressed, and is found in the regions of the centromere and telomeres. The euchromatin usually contains genes that are transcribed, with DNA packaged around nucleosomes but not further compacted. Nucleosome These data allow us to build a model for the organization of the nucleosome. Approximately 145 bp of genomic DNA is associated with the octamer of four histone proteins (8 proteins). The DNA located between the nucleosomes, about 50 base pairs of DNA separating each pair of nucleosomes, termed the linker DNA which is the most basic (10 nm fiber or beads) on a string conformation it adds stability to the chromatin structure. The H1 histone interacts with the nucleosome and some of the linker DNA as it enters and exits the nucleosome, which implies that it may serve to cross-link nucleosomes. Types and composition of histones From DNA double helix to interphase chromatin. Binding of basic histone proteins causes the 2 nm DNA double helix to undergo coiling, forming first a 10 nm filament studded with nucleosomes that is further coiled to give a 30 nm chromatin fiber. In interphase, the chromatin fiber is organized in looped domains, each containing about 50-200 kilobases of DNA, that are attached to a central scaffold of nonhistone proteins. High levels of gene expression require local uncoiling of the chromatin fiber to give the 10 nm nucleosomal filaments. Histones make five types of interactions with DNA: Hydrogen bonds between side chains of basic amino acids (especially lysine and arginine) and phosphate oxygens on DNA Helix-dipoles form alpha-helixes in H2B, H3, and H4 cause a net positive charge to accumulate at the point of interaction with negatively charged phosphate groups on DNA. Hydrogen bonds between the DNA backbone and the amide group on the main chain of histone proteins Nonpolar interactions between the histone and deoxyribose sugars on DNA. Non-specific minor groove insertions of the H3 and H2B N-terminal tails into two minor grooves each on the DNA molecule Translation in Prokaryotes TRANSLATION Translation involves the conversion of the base sequence of the mRNA (genetic code) into amino acid sequence of a polypeptide. A protein consists of one or more polypeptides; which are composed of amino acid. Every polypeptide has a free amino group at one end (N terminus) and a free carboxyl group at the other end (C terminus). TRANSLATION Twenty amino acids are used to make proteins in all living cells. Amino acid of polypeptide are joined by a peptide bond formed between the carboxyl group of one amino acid and the amino group of an adjacent amino acid- amino acid. The nature of the genetic code 1. The code is a triplet code. Each mRNA codon that specifies an amino acid in a polypeptide chain consists of three nucleotides. The evidence that the genetic code is a triplet code came from genetic experiments done by Crick 1960. 2. The code is continuous. The mRNA is read continuously, three nucleotides at a time. Triplet genetic codons and their corresponding amino acids The nature of the genetic code 3. The code is almost universal. Almost all the organisms share the same genetic language. Therefor, we can isolate an mRNA from one organism, translate it by using machinery from an other organism and produce the protein. The code is not completely universal, e.g. mitochondria of some organisms have minor changes in the code. 4. The code is degenerate, with two exceptions, more than one codon occurs for each amino acid (AUG codes for methionine and UGG codes for tryptophan); this multiple coding is called the degeneracy of the code. The nature of the genetic code 5. The code has start and stop signals. In both eukaryotes, and prokaryotes, AUG (codes for methionine) is always the start codon for protein synthesis. Only 61 of the 64 codons specify amino acids; these codons are called sense codons. The other 3 codons UAA, UAG, and UGA do not specify an amino acid. These three codons are the stop codons or chain-terminating codons. Composition of eukaryotic ribosomes RNA-binding sites in the ribosome Each ribosome has: a binding site for mRNA three binding sites for tRNA A-site: aminoacyl-tRNA P-site: peptidyl-tRNA E-site: exit tRNA molecules: matching amino acids to codons in mRNA Translation: The process of protein synthesis Polypeptide synthesis takes place on ribosomes, where the genetic message encoded in mRNA is translated. mRNA is translated in the 5` to 3` direction and the polypeptide is made in the N-terminal to C-terminal direction. mRNA codon recognizes the tRNA anticodon and not the amino acid carried by the tRNA. Adding an amino acid to tRNA: The correct amino acid attaches to the tRNA by an enzyme called aminoacyl-tRNA synthetase. The process is called aminoacylation, or charging and produces an aminoacyl-tRNA (or charged tRNA). Aminoacylation uses energy from ATP hydrolysis. 1.The initiation phase of protein synthesis in eukaryotes 1. Initiation complex (small ribosomal subunit + initiation factors) binds mRNA and searches for start codon 2. Large ribosomal subunit adds to the complex 3. Translation starts 4.... 2. The Elongation phase of protein synthesis in eukaryotes Step1:An aminoacyl-tRNA molecule binds to the A-site on the ribosome Step2:A new peptide bond is formed Step3: The small subunit moves a distance of three nucleotides along the mRNA chain ejecting the spent tRNA molecule Step4: The next aminoacyl-tRNA molecule binds to the A-site on the ribosome Step5:... 3.The Termination phase of protein synthesis in eukaryotes Termination of translation occurs when a stop codon (UAA, UAG, or UGA) is encountered. When the ribosome encounters the stop codon, the growing polypeptide is released and the ribosome subunits dissociate and leave the mRNA. After many ribosomes have completed translation, the mRNA is degraded so the nucleotides can be reused in another transcription reaction.