Safety in Engineering PDF
Document Details
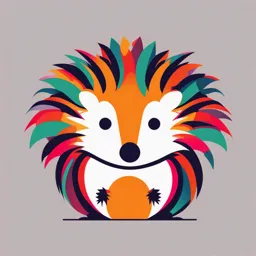
Uploaded by EnterprisingSteelDrums1699
Tags
Summary
This document discusses safety procedures in an engineering context. It emphasizes different safety aspects such as personal protective equipment, electrical safety, fire prevention, and responsibilities of employers and employees. It covers various types of safety risks and mitigation methods.
Full Transcript
Chapter One: Safety 1.1 Accidents: “Accidents do not happen; they are caused”. This often repeated statement is true. If you are honest with yourself, you cannot think of a single accident that could not have been prevented by care and forethought. Accident...
Chapter One: Safety 1.1 Accidents: “Accidents do not happen; they are caused”. This often repeated statement is true. If you are honest with yourself, you cannot think of a single accident that could not have been prevented by care and forethought. Accidents can and must be prevented. They cost the country millions of lost man-hours of production each year, and the cost of human suffering and misery is immeasurable. Therefore, this part is devoted to the problem of personal safety, as the individual is usually the cause and the casualty of most accidents 1.2 Personal Safety For the young worker just entering the industry, safety is a very personal matter. It is the young worker who is most prone to be involved in an accident because of his inexperience. Here are a few suggestions on how to avoid accidents: 1.2.1 Protective clothing a-Overalls These must be worn at all times and should be close-fitting. Sleeves must be buttoned at the wrist or rolled above the elbows. Loose clothing and neckties are easily caught in moving components and will drag the wearer into the machine. b-Shoes The possible injuries associated with unsuitable shoes are severe puncture wounds caused by treading on sharp objects and crushed toes caused by falling objects. So it is necessary to avoid that by selecting suitable shoes that can withstand the working environment. c-Goggles It is easy to lose sight of an eye and almost impossible to restore it. Always wear goggles when grinding, chipping, or machining a material that splinters - such as free-cutting brass. Be very careful if you wear contact lenses and don't look by any chance into the direction of the bright light emitted from the welding operation. pg. 1 Figure 1: Goggles d- Gloves Always wear gloves when handling raw materials. Sheets, bars, castings, fabrications, and forgings, all are delivered to the works with sharp edges waiting for the unwary. 1.2.2 Long Hair If hair becomes caught in a machine, serious scalp injuries may result. These can lead to permanent disfigurement and worse. Drilling turning and rotating driven machines, are especially dangerous in this respect. 1.2.3 Untidiness This is the main cause of many accidents. Never obstruct the gangway. Always clean up spilled oil and water immediately. Untidiness causes bad falls, which are often more serious because the victim thinks he is in a clear and safe area. Never leave tools after using them and scrap material around. Apart from being dangerous, this is a sure sign of an inefficient and unskilled worker. 1.2.4 Behaviors Any action that can distract a worker's attention and break his concentration, such as pushing, shouting, throwing things, or practical joking, can cause serious accidents, as well as make you unpopular with your more mature workmates. A good workshop rule is "Work hard in the shop and play hard away from it". pg. 2 1.2.5 Regulations Men with many years of experience in your sector have created these. Some rules may not seem necessary, or you may see older men who should know better breaking the rules. Don't be influenced. Obey all rules to the letter, and you'll live long enough to understand their purpose. The most important regulations involve smoking. Many processes produce explosive dust and gases. A single spark can cause a fatal accident. If dust and fume extraction fans are provided, always make sure that you use them. Do not tamper with electrical equipment, if you are not satisfied with its condition, ask a qualified electrician to carry out the repair. Compressed air services can help a lot with portable tools and blowing away scraps and chips when used by the right people. However, one common accident is blindness caused by practical jokers who squirt their workmates in the face with an air hose-not realizing the force behind a high-velocity jet or compressed air. 1.2.6 Guards In order to make sure workers are safe, the industry spends a lot of time and creativity on guarding machinery. Very often, this effort is frustrated by irresponsible workers removing the guards from their machines so that they can work faster. Never use a machine unless the guard is in place and functioning correctly. Figure 2: Machine Guards 1.2.7 Lifting Never risk straining yourself while lifting a heavy weight; always use the lifting tackle provided. Remember, because you are using a lifting tackle, the weight may be considerable, and if it slips, a serious accident can occur. Seek advice on the correct use of hooks and slings. Never stand under the load. 1.2.8 Use & Maintenance of hand tools pg. 3 1.3 Electrical hazards General objective: The student knows the importance of safe electrical work in protecting life and property. Some type of electrical equipment is used in every factory. Electricity should be treated carefully. It cannot be seen or heard, but it can kill. Even if it is not fatal, serious disablement can result from shock and burns. Also, a great deal of damage to property and goods can be caused, usually through the fire, as a result of faulty wiring or faulty equipment. 1.3.1 The human body as part of a circuit The specific objective is to draw a circuit diagram including the human body as a resistance. In order to minimize the risk of shock and fire, any metalwork other than the intended current-carrying conductor must be connected to the earth. The neutral of the electrical supply is earthed at the source of distribution, i.e. the supply path for the current will be available through the earth when a fault occurs, see Fig. (3). To be effective, this earth path must be sufficiently low resistance to pass are relatively high current when a fault occurs. This higher current will in turn operate the safety device in the circuit, i.e. the fuse will "blow". Figure 3: Earthen System Accidents happen when the body provides a direct connection between the live conductors- when a part of the body or a tool touches equipment connected to the supply. More often, however, the connection of the human body is between one live conductor and the earth, through the floor or adjacent metalwork, see Fig. (4). Metal pipes carrying water, gas, or steam, concrete floors, radiators, and machine structures all readily provide a conducting path of this kind. pg. 4 Figure 4 1.3.2 Electrical sources of danger Specific objective: To list likely sources of electrical danger. With the increasing use of portable electrical equipment, the sources of danger are many. It is essential that a correctly wired plug be used for all portable equipment, Fig. (5). Never improvise by jamming wires in sockets with nails or matches. Molded rubber plugs are preferable to brittle plastic types since they are less liable to mechanical damage. All electrical connections must be secure - loose wires or connections can arc, leading to overheating which could result in a fire. A fuse of the correct rating must be fitted - this is your safeguard if a fault develops. Never use makeshift fuses as pieces of wire. Any external metal parts must be correctly earthed so that, if any fault develops, the fuse will blow and interrupt the electrical supply - never run power tools from lamp sockets. The connection between the plug and the equipment should be made with the correct three core flexible cable suited to the current rating of the equipment. Never use braid covered cable for portable equipment, it can fray, and lead to a fault BROWN wire - LIVE conductor BLUE wire - NEUTRAL conductor GREEN/YELLOW wire - EARTH conductor Figure 5: Wire Type pg. 5 Any external metal parts must be correctly earthed so that if any fault develops, the fuse will blow and interrupt the electrical supply - never run power tools from lamp sockets. The connection between the plug and the equipment should be made with the correct three- core flexible cable suited to the current rating of the equipment. Never use braid-covered cable for portable equipment, it can fray, and lead to a fault and cause shock or fire. The old threadbare cable should never be used -have it replaced immediately by a qualified electrician. Do not, under any circumstances, interfere with any electrical equipment or attempt to repair it yourself. Equipment should always be carefully disconnected from the mains before making any adjustment, even changing a lamp. 1.4 Fire Fighting The best way you can help is to learn the correct fire drill, both how to give the alarm and how to leave the building. It only requires one person to panic and run in the wrong way to cause a disaster. “In an emergency never lose your head”. Smoke is the main cause of panic. It spreads quickly through a building, reducing visibility and increasing the risk of falls down staircases. It causes choking and even death by asphyxiation. If you do have to help to fight a fire, there are some basic rules to remember. Fire is the rapid oxidation (burning) of combustion (burnable) materials at comparatively high temperatures. Remove the air or the fuel or lower the temperature. Do not continue to fight a fire: 1- if it is dangerous to do so; 2-if there is a possibility that the escape route may be cut off by fire or smoke; 3- If the fire continues to grow in spite of your efforts. 4-If there are gas cylinders or explosives in the vicinity of the fire. Recall that prevention is always better than cure, and fire prevention is better than firefighting. Fires have a small beginning and it is amongst rubbish left lying about that many fires originate. So make a practice of constantly removing rubbish, shavings, and off- cuts, and remove intervals to a safe place. Highly flammable materials should be stored in specially designed and equipped compounds away from the main working areas. 1.5 Safety Duties in the Factory 1.5.1 Duties of the Employer The employer should provide: Healthy and safe plants and systems maintained in good order. Healthy and safe facilities for handling, storage, and transport of articles and substances. Health-and-safety information, instruction, training, and supervision. A healthy and safe place of work maintained in good order, with a safe means of getting to and from it. pg. 6 A healthy and safe working environment with adequate facilities and arrangements for welfare. 1.5.2 Duties of Employees The duty of the employee at work: To take reasonable care of the health and safety of himself, other workers, and the general public. To observe the provisions of the acting safety regulations wherever applicable to him or matters under his control. To co-operate with his employer in complying with the acting safety regulations. pg. 7 Chapter Two PROPERTIES OF ENGINEERING MATERIALS 2.1 Properties of Engineering Materials The intelligent use of all types of engineering materials depends upon a thorough knowledge of their particular properties. This knowledge is obtained in a cumulative manner from scientifically designed tests and from the previous experiences of engineers and designers with materials. It is possible to approach the study of the properties of engineering materials from both a qualitative or descriptive viewpoint and from a quantitative viewpoint. The range of properties found in different classes of materials is very large and Table 2.1 provides a partial classification. Table 2.1 A Classification of the Properties of Engineering Materials Class Property Class Property Physical Dimensions, shape Mechanical Strength: tension, Density of specific compression, shear gravity porosity and flexure (under Moisture content static, impact of Macrostructure fatigue conditions) stiffness, toughness, elasticity, plasticity, ductility, brittleness, hardness and wear resistance. Chemical Oxide or compound Thermal Specific heat composition Expansion Acidity or alkalinity Conductivity Resistance to corrosion or weathering. Physico-chemical Water-absorptive or Electrical Conductivity water-repclient action & Magnetic permeability Shrinkage and well Magnetic Culvanic action due to moisture changes Acoustical Sound Transmission Optical Colour Sound Reflection Light transmission Light reflection 2.2 Some Important Physical Thermal And Electrical Properties 2.2.1 The Density The density of a material is its mass per unit volume, and is directly related to: 1. The atomic weights of the atoms or other atomic particles present in the material, and 2. The number of atoms of each type per unit volume which is in turn determined by the method of packing and the inter-atomic distances. Heavy stems packed closely together in a structure having a high coordination number give a material of high density, while a large amount of free space in the structure makes for low density. The density of a solid usually found by weighing it in air and then determining its volume by a displacement technique. 2.2.2 The Porosity The porosity of a substance reveals the volume of voids or pores present in the material, and is usually expressed as a percentage of unit volume. Porosity may arise out of processes of natural growth, as in wood; it may be deliberately introduced into a material by processing, as in a firebrick, or it may occur accidentally, as in some metals because of imperfect casting techniques. It has considerable influence in particular instances. Upon such properties as heat conductivity, refractoriness, strength, brittleness and absorptive capacity. 2.2.3 Moisture Content Moisture content can have considerable influence upon the mechanical properties of many porous materials. Wood is the most outstanding example in this field. Freshly felled green wood often contains water in amounts two to three times the actual amount of wood substance present. Seasoning reduces this down to from 7 % to 16 % moisture, this being accompanied by a tremendous increase in strength properties. Furthermore, the loss of moisture is an accompanied by a corresponding decrease in thermal and electrical conductivities. The specific heat of a substance is the amount of heat required to cause unit mass of that substance to rise through one degree of temperature. 2.2.4 Thermal Conductivity The ability of a material to transmit heat energy is expressed in terms of its thermal conductivity; metals are the best conductors of heat. One common method used in engineering is to rate different materials in terms of their relative thermal conductivities. Table (2.2): Thermal Conductivities Compared with Silver as the Standard of 100 Metals Non-Metals Silver = 100 Glass = 0.15 Copper = 90 Asbestos = 0.06 Aluminum = 48 Wood (Seasoned) = 0.05 Brass = 27 Cork = 0.01 Mercury = 1.5 Felt = 0.01 2.2.5 Heat Resistance Heat resistance is largely determined by the melting point of a material together with its chemical stability, oxidation resistance, and ability to retain strength at high temperatures. Metals Melting point oC Materials Melting point oC Mercury -39 Silica Brick 1700 Lead 327 Magnesia Brick 1900 Aluminum 660 Graphite Brick 3500 Copper 1083 Titanium 1812 Iron 1535 Tungsten Carbide 2870 Tungsten 3380 Zircon 1852 2.2.6 Electrical Conductivity The ability of a material to conduct an electric current depends upon internal structure. Conduction in solid materials is the result of the movement of electrons throughout the atomic lattice, while liquids conduct if they possess ions, which can move in accordance with an applied potential difference. Table (2.4) Good Conductors Poor Conductors and Insulators Good Conductors Poor Conductors Insulators All metals Water Air Carbon Stone Glass Wood Silk Cotton Rubber (Pure) Oils Paper Many plastics Many Ceramics 2.2.7 Optical Properties The optical properties of an engineering material may or may not be of importance, although such properties as color and luster often assist in the identification of a material. When light rays strikes a non-luminous body they will be transmitted, reflected, or absorbed according to the nature of the body, the color of the body depending upon the degree of transmission, reflection or absorption. 2.2.8 Mechanical Properties The mechanical properties of materials are usually of primary interest to the engineer since they determine the ability of a machine or structure to withstand loads without failure. Very often, if a material exhibits good strength properties but is deficient in some other direction, steps will be taken to overcome this deficiency rather than to find a substitute material. Thus, steal, which possesses good strength properties but corrodes badly by rusting, is protected by galvanizing or by painting. 2.2.8.1 Strength Strength determines the ability of a material to withstand stress without failure. Strength varies according to the type of applied loading, and thus it is possible to assess tensile, compressive shearing, and torsion strengths. 2.2.8.2 Hardness Hardness is usually defined in terms of the ability of a material to resist scratching, abrasion, indentation, or penetration. 2.2.8.3 Elasticity This is the ability to return to the original shape and dimensions of a material after being subjected to a load that caused, or tended to cause, deformation. The elastic properties of a material are of extreme importance to the structural engineer since materials loaded beyond their elastic range develop a permanent set and may cause a weakening of the whole structure by altering stresses imposed on other members within the structure. 2.2.8.4 Stiffness It is a measure of its ability to resist deformation or deflection under load, and is a property closely associated with elasticity. Very often the engineer is more concerned with the stiffness of a material than with its strength. For instance, suspended beams of steel and aluminum may both be strong enough to carry the required load but the aluminum beam will sag or deflect further. In other words, the steal beam is stiffer than the aluminum beam. 2.2.8.5 Plasticity It refers to the ability of a solid material to undergo some degree of permanent deformation without rupture. Plastic deformation will only occur after the elastic range has been exceeded. Many hot and cold working processes such as rolling, extruding, pressing, forging, and spinning depend upon plastic deformation and the ability of a material to alter its shape under relatively severs loading. Some materials, such as glass at room temperature ad cast iron, exhibit no plasticity and are termed brittle. 2.2.8.6 Workability or (Formability) It denotes the ability of a solid material to undergo all types of deformation processes without failure. It is clearly related to the concept of plastic deformation, and is generally considered to embody the properties of malleability and ductility. In the strict sense of the word, malleability is that property of a material that allows it to be hammered and rolled out into thin sheets. While ductility refers to the ability to be drawn out into thin wire. However, it is important to realize that some materials may be malleable and not ductile; lead, for instance, can be readily rolled and hammered out but cannot be drawn into wire. 2.3 Engineering Materials 2.3.1 Introduction Almost every substance known to man had found its way into the engineering workshop at some time or other. Neither this lecture nor in fact the entire course could hold all the facts about all these materials. Therefore, it is intended to show how engineering materials can be grouped into families. Then some of the more common materials will be taken from these groups and examined in greater detail. Table 2.5: Engineering Materials Metals Non-Metals Ferrous metals Non-ferrous metals Plastics Natural (Contain iron) and alloys (contain (Rubber, glass, no iron) wood, emery, Ceramics, diamonds, oil, etc) Wrought Iron Alloy Thermosetting Thermoplastics Steels plastics Plain Carbon steels Cast irons Table (2.5) shows the main groupings of engineering materials. The specialist in each group could break them down further and, in fact, no engineer or scientist can be expert in all materials. Metals and Non-Metals The elements may be roughly divided according to their properties into two groups, the metals and the non-metals. The characteristic properties of these two groups are summarized in Table (2.6). able 2.6 The Characteristic Properties of Metals and Non-Metals Metals Non-Metals Usually solid at ordinary May be solids liquids or gases. temperatures. Usually dull if Solid. Are lustrous on a freshly-cut surface. Usually brittle if solid. Usually malleable and ductile to some degree. Usually non-conductors Usually good thermal and (Insulators) of heat and electricity. electrical conductors. Do not alloy, but may chemically combine to form compounds. Usually form alloys. An illustration of these properties may be obtained by comparing copper and sulfur. Copper, a reddish-pink metal, has excellent malleability and ductility, it melts at 1083 oC, it forms a wide range of alloys, and both it and its alloys have excellent conductivities. On the other hand, the most common form of sulfur is a yellow vitreous non-metallic brittle solid or powder, It melts at 119 oC, it is a non-conductor and although it combines chemically with a wide range of metals to form sulfides, it does not form any alloys. 2.3.3.a Metals The engineer looks at metals from a very different viewpoint from that of the pure scientist, being more concerned with their properties and applications. Classification And Grouping Of Engineering Materials With Their Behavior In Chemical Reactions A typical classification of metals as used in engineering would be as follows: METALS Pure Alloyed Coated Ferrous Non-ferrous Sintered Pure Metals Pure metals are those obtained when an ore is refined to yield a metallic element. An alloy is formed when two or more relatively pure metals are melted together to form a new metal having, in many instances, quite different from those of the two metals used in its manufacture. An outstanding example of this is the alloy formed 18% chromium and 8% nickel are added to low- carbon steel, forming a steel which will not rust or corrode, this being quite different from the behavior of the original low-carbon steel. Coated metals Coated metals, including such metals as tinplate, tern plate, galvanized iron, and zinc anneal, are manufactured so that the desirable properties of both metals can be married together. Sintered Metals Sintered metals are those produced by the techniques of powder metallurgy, and they possess very different properties and structures from those of metals that have cast and worked using conventional techniques. Ferrous Metals A ferrous metal is one composed principally of iron, while non-ferrous metals are those composed principally of metals other than iron. It should be noted from the outset that a non-ferrous alloy may contain iron in some small proportion (for instance, high-tensile brass often contains about 1% iron by weight), and that ferrous alloys may contain quite significant proportions of non-ferrous metals. Ferrous Alloys Ferrous alloys are of the utmost importance in engineering, and are classified on the basis of the percentage of carbon and other alloying elements present. Same of the more important groupings are given below: 1- Dead mild steel: Containing between about 0.1% - 0.15% carbon. 2- Mild Steels: Containing between about 0.15% and 0.25% carbon, are used where low coat, moderate strength and good weldability are required. 3- Medium Carbon Steels: Containing between 0.3% and 0.6% carbon, these steels have higher strengths but reduced weldability. 4- High Carbon Steels and Carbon Tool Steels: Contain up to 1.5% carbon; they have poor weldability but can be heat-treated to become hard and tough. 5- Cast Irons: Contain between 2% and 4% carbon, and are excellent and inexpensive general purpose ferrous casting alloys. Alloy Steels The most common alloying metals added to steels are: Nickel: to refine the grain and strengthen the steel. Chromium: to improve the heat treatment and corrosion resistant properties. (Stainless steel contains a large amount of chromium. Molybdenum: reduce temper brittleness and enables an alloy steel to operate continuously at high temperatures without becoming brittle. Manganese: improve wear resistance. Steels containing a high percentage 14 percent) of manganese are used for bulldozer and plough blades. Tungsten & Cobalt improve the ability of steel or remain hard at high temperature and are used extensively in cutting tool materials. Non-Ferrous Metals The term non-ferrous metals refer to the 38 metals other than iron that are known to engineer. The non-ferrous metals that are most commonly used by engineers are shown in Table (2.7). Table (2.7): Common Non-Ferrous Metals Metal Density Melting Properties Typical Uses g/cm3 point Aluminum 2.7 660 Highest of the commonly The base of used metals, high many electrical and thermal engineering conductivities, soft, alloys. Light ductile. weight electrical conductors. Copper 8.9 1083 Soft, ductile and low The base of brass tensile strength, it is and bronze much easier to join by alloys. It is used soldering aluminum. extensively for Corrosion resistance. electrical conductors and heat exchangers Lead 11.3 328 Soft, ductile and very Electrical cable low strength. High sheaths, the base corrosion resistance. of solder alloys. The grids for accumulators plate. Lining chemical plants. Silver 10.5 960 Soft, ductile and very Widely used in low tensile strength. electrical and Highest conductivity for electronic any metal. engineering for switch and relay contacts. Tin 7.3 232 Resist corrosion. Coats sheet mild steel to give tin plate. Used in soft solders. An alloying element in bronzes. Zinc 7.1 420 Soft, ductile and low Used extensively tensile strength. to coat sheet Corrosion resistant. steel to give galvanized iron. The base of die- casting alloys, an alloying element in brass. In addition to the above non-ferrous metals there are: Chromium, Cobalt, Manganese, Molybdenum, and Nickel. These are used as alloying elements in alloy steels and are described above. Chromium and nickel are also electroplated onto a variety of metals, both as a decorative finish and to give protection against corrosion. The similarity in appearance between many materials of different physical properties makes it essential that some form of permanent identification should be marked on them, e.g. color-coding. 2.3.3.b Non-Metals Plastics There are an ever-increasing number of synthetic materials available under the name of plastic. These materials show no plastic properties in their finished condition, in fact many of them are elastic, but during the molding operation by which they are formed they are reduced to a plastic condition by heating to just above the temperature of boiling water. There are two main groups of plastic materials: 1. Thermosetting plastics. These undergo a chemical change during molding and can never be softened by reheating. These materials are hard, rigid, and rather brittle. 2. Thermoplastics: These can be softened as often as they are reheated. They are not as rigid as the first group and tend to be tougher. Properties of Plastics The properties of plastics can vary widely, but all plastic materials have some properties in common. 1. Electrical Insulation All plastic materials exhibit good electrical insulation properties. However, their usefulness in this field is limited by their low heat-resistance and softness. 2. Strength/ Weight Ratio Plastic materials vary in strength very considerably. Some of the stronger, such as nylon, compare favorably with the weaker metals. All of them are much lighter than most metals. Therefore, properly chosen and proportioned their strength/weight ratio compares favorably with many light alloys. 3. Corrosion Resistance All plastic materials are inert to most inorganic chemicals. Thus they can be used in environments that are hostile to the most corrosion resistant metals. They are superior to rubber in that they are resistant to attack by oils and greases. 2.3.3.c Natural Materials Rubber Anti-vibration mountings. Coolant and compressed air hoses. Transmission belts. Glass Spirit level vials (the tube containing the bubble). Optical measuring instrument lenses. Wood Casting Patterns. Emery Abrasive wheels, and grinding pastes. (Now a day usually produced artificially to control quality). Ceramics Cutting-tool timps. Electrical Insulators. Diamonds Turning tools for fine finishing light alloys. Lapping Chapter Three: Metrology 3.1 Definitions Measurement is the process of giving a physical variable a particular number. The measurement system serving as a tool for this quantification of variables. Calibration is the process of putting a known value into a measurement system in order to see what comes out of the system. The known value used for the calibration is called the standard. This standard may be a piece of equipment or an object having a well-defined physical attribute to be used as a comparison. A dimension defines a physical variable that is used to describe some aspect of a physical system. A unit determines the fundamental value associated with any dimension. A unit defines a measure of a dimension. For example, terms such as mass, length, and time describe the basic dimensions with which we associate the units of kilogram, meter, and second. The term "metrication" refers to the conversion of English units to metric units. Table (3.1) shows standard dimensions and units in SI and English units: Table 3.1 Dimension Unit Unit SI US Length Meter (m) Foot (ft) Mass Kilogram (kg) Pound-mass (Ib.m) Time Second (s) Second (s) Force Newton (N) Pound-force (Ib) Temperature Kelvin (K) Rankin (R) True value is the value obtained from an instrument or measuring system deemed by experts to be acceptably accurate for the purpose to which the results are put. pg. 1 Correctness or accuracy is the degree of conformity of a measured or calculated value to some recognized standard or specified value (the "true value"). The difference between the measured and true value is the error of the measurement E = true value - measured value Precision is the repeatability of the measuring process or the ability to reproduce a certain reading within a given accuracy. Sensitivity is the ability to detect differences in a quantity being measured. If one micrometer is able to measure a perceptible difference and another is not, the first one is more sensitive. Sensitivity and readability are associated with the device while precision and accuracy are associated with the measuring process. Tolerance is the amount of variation permitted in the part or the total variation allowed in a given dimension. “A shaft” might have a nominal size of 63.5 mm. But for practical reasons this figure could not be maintained in manufacturing without great cost. Hence a certain tolerance would be added and if a variation of +0.08 mm could be permitted, the dimension would state 63.5±0.08mm. Figure 3.1 A shaft pg. 2 3.2 A rule The degree of accuracy to which work may be produced when measurements are made by a rule depends on the quality of the rule, and on the skill of the user. The marks on a good-class rule vary from 0.12mm to 0.18mm wide, so that we cannot expect to obtain a degree of accuracy much closer than within 0.012mm, but a good workman should be able to work as closely as this. The most useful and convenient markings are millimeters on one face of the rule. It is graduated in millimeters on one edge and 1/2 millimeter on the other. A good rule is worth looking after and should neither be used as a feeler gauge under shaper tools or milling cutters, nor for scraping Tee slots and machine tables. The end of the rule particularly should be preserved from wear, as it generally forms the basis for one end of the dimensions. Rusting of the rule should be avoided by oiling it during weekends and when it is not in use. The thickness of a rule may often be put to good use, and the rules mentioned above will be found 1mm and 1/2mm thick respectively. When taking measurements with "a rule", it should be so held that the graduation lines are as close as possible (preferably touching) to the faces being measured. The eye which is observing the reading should be as near as possible opposite to the mark being read. This avoids what is called parallax, and the reader may try for himself the errors which parallax may cause if he reads the large hand of a clock when it is vertical, by looking at it not from the front, but from a very acute side angle. Figure 3.2: A rule pg. 3 3.3 Inside & Outside Calipers Fig. (3.3) shows typical inside and outside calipers. These are devices for transferring the distance between the faces of a component to the rule and helping to reduce sighting errors. The shape of calipers varies according to whether they are for external or internal use, and they may be stiffly jointed at the hinge of the legs; their opening is maintained by the friction at the joint; or the joint may be free and spring-controlled, with the opening being adjusted and maintained by a nut working on a screw. Screw-controlled spring calipers are more instrument and tool making. Figure 3.3 The Construction & Use of Calipers The constructional features and some uses of calipers are shown in Figure 3.4 The accurate use of calipers depends upon a highly developed sense of feeling that can only be acquired by practice. When using calipers, the following rules should be observed: 1. Hold the caliper gently and near the joint. 2. Hold them square to the work. 3. No force should be used to spring them over the work. Contact should only just be felt. 4. The caliper should be handled and laid down gently to avoid disturbing the setting. pg. 4 5. When a nice feel has been obtained on the job the size should be read on a rule by resting the end of one leg on the end of the rule and taking the reading at the other. To set outside calipers to a fairly particular size they should be set from a block or gauge of the given dimension. 6. The opening of inside calipers may be checked by a rule or micrometer. If the rule is used, hold the end of the rule and one leg of the calipers pressed against a vertical flat surface (e.g. edge of a lathe bed) and read the other leg. Figure 3.4 Transfer Calipers These are used to measure the sizes of undercuts where it is impossible to insert or remove conventional calipers without disturbing their setting. (Figure 3.5a) shows the inside and outside transfer calipers together. After setting the caliper, the auxiliary leg is locked. The contact leg is released as shown in (Figure 3.5 b) After the removal of the caliper from the component, the contact leg is again brought back into position against the auxiliary leg and the setting is measured. pg. 5 Figure 3.5 3.4 The Vernier Caliper Figure (3.6) shows the Vernier caliper, which is another precision measuring device commonly used in the workshop. It will be seen that, unlike the micrometer caliper, the Vernier caliper can make both inside and outside measurements on one instrument. Again, the Vernier caliper reads from zero to the full length of its beam scale, whereas the micrometer only reads over a range of 25mm. However, the Vernier pg. 6 caliper does not give such accurate readings as the micrometer for the following reasons: 1. It is difficult to obtain a correct feel due to its size and weight. 2. The scales are difficult to read even with the aid of a magnifying glass. Note: For inside readings, the thickness of the jaws must be added to the scale reading. Vernier calipers are made with beam scales ranging from135mm- 1000mm with various accuracies (0.1mm, 0.05mm, and 0.02mm). Figure 3.6 The Vernier Scale We have seen that the purpose of a Vernier scale is to provide a more accurate and reliable method of estimating a part of a millimeter. The principle is illustrated in Figure (3.7) where the Vernier scale will allow accurate readings to 0.1mm. Figure 3.7 Note that the rule or fixed number is graduated in millimeters. If now, the width of the first division on the Vernier scale is 9/10 mm, then a movement of 1/10 mm is easily obtained by bringing the first line on the Vernier scale to coincide with the first line on the fixed scale. Each division on the Vernier scale will also be 9/10 mm; therefore, the second line will be 2/10 mm from pg. 7 the nearest line on the fixed scale. It is evident that the tenth line on the Vernier scale now coincides with a line on the fixed scale. The accuracy of the Vernier scale, therefore, is the difference between the width of the first division on the Vernier scale, and the width of the first division on the fixed scale. The measurement of the diameter of a bar proceeds in the same manner as before. The number of cm is read off, the number of mm, and now the Vernier scale is used to determine the part of 1 mm that remains. Thus, in Figure (3.8) the reading would be 11.7 mm, made up as follows: Figure 3.8 Vernier reading to 2/100 or 0.02 mm is of real value for both measuring and marking out. The 0.02 mm Vernier The accuracy of a Vernier scale, as previously stated, is the difference between a division on the Vernier scale and a division on the fixed scale. In order to read 0.02 mm, this must be the difference between the widths of these divisions. Figure (3.9) shows a close view of the Vernier scale at zero reading. Note that the Vernier scale covers a distance of 1.2 cm, or as 1 cm = 10 mm, the total length of the Vernier scale =12 mm. Always remember that the main or fixed scale is numbered in centimeters, and it is necessary to multiply by 10 to obtain millimeters. From 0 to 1 on the fixed scale, we have 20 divisions, hence each division = 1/2 or 0.5 mm. Now to find the width of a division on the sliding scale we pg. 8 must divide the total length of the sliding scale by the number of divisions, thus: Width of one division on Vernier or sliding scale = 12/25 mm or 0.48mm Hence the accuracy of the Vernier scale will be the difference between a division on the fixed scale and one on the sliding scale. Thus: 0.5 mm - 0.48 mm = 0.02 or two-hundredths of a millimeter. Perhaps it is as well to admit that the principle of the Vernier is not readily assimilated by students, for it is possible to use Vernier calipers and yet not fully understand the principle involved. But the use of a Vernier is not confined to Vernier calipers. There are Vernier micrometers, and Vernier scales are widely used on rotary tables, dividing heads, barometers, sextants, and protractors. It is well worthwhile to master the principle involved, remembering that it is only a device to remove the "guesswork" involved when an engineer has to estimate a part of a division. pg. 9 Figure 3.9 3.5 Micrometers Although the Vernier is an excellent device, there is still the possibility of small errors due to the smallness of the Vernier scale causing difficulty in deciding which are coincident to magnify or enlarge the reading, and this is the principle of the micrometer. Figure (3.10) shows a 0.25mm external micrometer, having the main parts named. The micrometer, as shown in the figure is used for quick, accurate measurements to the one hundred of a millimeter. The micrometer requires the use of an accurate screw thread as a means of obtaining a measurement. The screw is attached to a spindle and is turned by the movement of a thimble or ratchet at the end. The barrel, which is attached to the frame, acts as a nut to engage the screw threads, which are accurately made with a pitch of 0.5mm. Each revolution of the thimble advances the screw 0.5mm. On the barrel, a datum line is graduated with two sets of division marks. The set below the datum line reads in millimeters, and the set above the line reads in half millimeters. The thimble scale is marked in 50 equal divisions, figured in fives so that each small division on the thimble represents 1/50 of 1/2mm, which is 1/100 mm or 0.01mm. To read the metric micrometer to 0.01mm, examine Figure (3.11) and first note the whole number of major divisions on the barrel, then observe whether there is a half millimeter visible on top of the datum line and last read the thimble for a hundred. The thimble reading is the line coinciding with the datum line. Example are given in Figure 3.11 pg. 10 Figure 3.10 Figure 3.11 pg. 11 3.6 Internal Micrometer The internal micrometer, Fig. (3.11) is designed for inside measurement and consists of a micrometer measuring head to which may be added extension rods and a spacing collar to cover a wide range of measurements. Each extension rod is marked with the respective capacity of the micrometer when that particular rod is used. The smallest size is 25-55 mm with a measuring range of 5mm. The next size covers 50-120mm with a measuring range of 10mm, while the largest covers 200-825mm. Reading is taken in the same way as described for the external micrometer, although, as already stated, the measuring range of the micrometer head is reduced to 5 or 10mm. Great care must be taken when using, this instrument, as each of the measuring anvils has a spherical end, resulting in point contact. Experience in use is essential to pg. 12 Chapter Four: Casting Process Casting process is considered one of the very old process that had been used in the shaping of material, it was used before 6000 years before production of jowls, arrow heads and another metal tools, casting is used for the production of (transportation equipment, transportation means, agricultural machines and mechanical machine). 4.1 Definition Casting is the process in which molten metal flows by gravity or other force into a mold where is solidifies in the shape of the cavity (simply: melt the metal, pour it in a mold, and let it freeze), or it is the process of forming objects by putting liquid or viscous material into a prepared mold or form, or it is the process in which metal shapes are formed by pouring the liquid metal in mold ,this mold contain cavity ,and the metal take the shape of that cavity after freeze, so the shape of casting is determined by the shape of the cavity of mold. Casting is an object formed by allowing the material into solidify. A foundry is the collection of necessary materials and equipment to produce a casting. Casting process is usually conducted in special place called foundry. A mold is the container that has the cavity of the shape to be cast. In this chapter, the sand casting will receive first attention because it one of the oldest operations (4000 BC) and it is the most used for casting process, sand casting is the suited for iron and steel at their high melting temperature but also predominates for aluminum, brass, bronze, and magnesium. 4.1.1Type of cold forming mold Non-permanent mold, if the mold is broken to take the casting as in sand casting Permanent mold, if the mold is not broken and can be used again for similar casting process as in (non-permanent mold casting) 4.1.2 Type of cold forming mold Molding materials Molding equipment Tools patterns Melting equipment Gloves and other safety preparation. 1 4.2 Molding Procedures A simple procedure for molding an aluminum tray using removable pattern is illustrated in figure (1) Figure 1: simple procedure for molding an aluminum tray 2 4.2.1 Mold prepared characteristics The mold must be strong enough to hold weight of the metal. The mold must resist the elusive action the rapidly flowing metal during pouring. The mold must generate minimum amount of gas when filled with molten metal gases contaminate the metal and can disrupt the mold. The mold must be constructed so any gases formed can pass through the body of the mold itself, rather than penetrate the metal. The mold must withstand the high temperature of the metal and strip away cleanly from the casting after calling. 4.3 Sand Casting 4.3.1 Mold Sand Mold sand consist of silica particles and clay and water, these component are mixed so the clay absorb most of the water transformed into adhesive material cover the silica particles, with the compression and ramming for these composition silica particles adhere together and give the mold good resistance and solidification enable it to stand in front of different condition. There are many properties that we must know about the sand which called sand quality and these quality are: Strength: the ability of the sand to maintain the stage of the mold Permeability: allowing a lot of air and gas to pass through. Thermal stability: it is the resistance to cracking and bucking due to the stress Collapsibility: allowable shrinking without cracking used. Reusability: if the sand can be reused Sand Type/ Sand Classification Green sand molds: It consists of sand, clay, and water, and this sand is common and has many advantages it can be used to create complex part geometry, it is capable of producing parts to net shape, it is capable of proven very large parts, and it can be done on any metal that can be heated to the liquid state, but on the other hand it has some disadvantages in which it makes some limitations on mechanical properties, porosity problems poor dimensional accuracy, and surface finish and lizard environment to humans. Dry sand mold: In which organic binders rather than clay are used and also backing is used. Skin-dried mold: In which the surface of the mold is dried to a depth of 1-2 cm by torches or heating lamps, and special bonding material such as organic is added. 3 4.3.2 Pattern Mold sand is compressed or rammed into a special box called a molding box flask, the mold for this blank is made in a flask that consists of two parts, the top is called the cope and the lower is called the drag. The parts of the flask are held in a definite relation to one another by pins on either side of the drag, which fit into openings in angle clips fastened to the side of the cope when more than two sections are needed to increase the depth of the cope or the drag intermediate flask section known as the cheek are used. A flask is a wood or metal frame in which a mold is made.it must be strong and rigid so as not to distort when it is handled or when sand is rammed into it, it must also resist the pressure of the molten metal during casting. A pattern is a form used to prepare and produce a mold cavity, it is another tool in the hands of a foundry man. It has been said that poor casting may be produced from a good pattern, but good casting will not be made from a poor pattern. The first step in making a casting is to prepare a model, known as a pattern that differs in a number of respects from the resulting casting. These differences known as pattern allowances, compensate for metal shrinkage, provide sufficient metal for mechanical surfaces and facilitate molding. Material used for pattern: Although patterns are made from a variety of woods, white pine is favorite because it is straight-grained, light, easy to work, and unlikely to warp or check. Other woods suitable for patterns are mahogany, cherry, beech, poplar, basswood, and maple, the last being especially desirable for work on the lathe. Pattern allowances: A pattern is a replica of casting that is used to make a mold cavity but it has slightly large dimensions. This change in the pattern is due to when the cast solidifies, it shrinks at some limit due to the metal shrinkage property at the time of cooling. So to compensate for this, a pattern is made a little bigger. These slight changes in the pattern are known as pattern allowance. Types of Pattern Allowance There are the following types of pattern allowances are used in the casting process. Shrinkage Allowance Draft Allowance Machining Allowance Deformation or Camber Allowance Shake or Rapping Allowance 4 Shrinkage allowance As metal solidifies and cools, it shrinks and contracts in size. To compensate for this a pattern is made larger than the finished casting by an amount called shrinkage allowance, dimension is not shown oversize on a part or pattern drawing to allow for shrinkage, but the pattern maker measures to the finished dimension with shrink rules, such a rule has a scale that is longer than standard by definite proportion such as (1/16, 1/8 or 3 in/ft. or 0.8,1.2, 2.3 mm/100mm). Shrinkage is different for different metals, different shapes of castings of the same metal, and different molding or casting methods, as an example, the shrinkage allowance of some materials is as follows: For C.I. Casting =0.8 mm/each 100mm For steel =2.0 mm/each 100mm For brass = 1.2 mm/each 100mm For aluminum =2.3 mm/each 100mm Figure 2: Shrinkage allowance Machining allowance: Is the amount by which dimensions on casting are made oversize to provide stock for machining, the dimension on a pattern drawing includes machining allowance? The amount of metal for machining must be no more than necessary but enough to assure that cutters can get an ample bite beneath and completely remove the hard scale and skin on the surface of the casting. Figure 3 Figure 3: Machining allowance 5 Distortion allowance: May be added to the dimensions of certain objects such as large flat plates and U-shaped casting that are expected to bend on cooling. The purpose of this allowance is to displace the pattern in such a way that casting will be of the proper shape and size after it distorts the process. Figure 4 Figure 4: Deformation or Camber Allowance Draft allowance: when the pattern is drawn from a mold the tendency to tear away the edges of the mold in contact with the pattern is greatly decreased if the surfaces of the pattern parallel to the direction it is being withdrawn, are slightly tapered. This tapering of the sides of the pattern known as the draft is done to provide a slight clearance for the pattern as it is lifted up. The draft is added to the exterior dimensions of a pattern and is usually 1/8 to ¼ per foot, Interior holes may draft as large as 3/4 inch per foot. Figure 5 Figure 5: Draft allowance 6 4.3.3 The core The core is used in the casting process in order to make a cavity or hole in the casting, the core is manufactured from the silica particles and organic ponds as linseed oil or starch in order to water. This core is formed in a special box called a core box to increase its resistance and hardness this kind of core is called dry sand core, and there is another kind called green sand core from wet sand. 4.4 To make the mold The steps of making the mold are summarized as the following: 1. The normal procedure is to make the first image of the piece and form the mold around this is called the pattern. 2. Checking the cleaning-free action of any loose pieces 3. Place the pattern on the molding board which fits the flask being used with the pins down (with the parting surface down on the board) 4. Molding sound is then riddled to cover the pattern to a depth of about 3 cm riddling is absolutely necessary for good reproduction of the pattern. 5. The riddle sand is then pressed around all the pockets and corners of the pattern with fingers 6. Sand is then put into the drag to a depth of (8-10) cm and packed carefully with a rammer, the remainder of the mold is filled and then rammed. The mold must be rammed carefully and uniformly hard in order to obtain a smooth easily cleaned casting surface, and to avoid metal penetration into the sand, swelling of the mold break out, or other casting defects on the other hand if it is rammed too hard it will not permit the steam and gas to escape when the molten metal enters the mold 7. After the ramming has been completed the excess sand is leveled off with a straightedge called a strike rod or bar, (to ensure the escape of gases when the casting is poured, small vent holes are made through the sand using vent wire). 8. The bottom board is placed on the drag, and clamps are applied to hold the drag between the ram-up board and the bottom board. 9. The drag is then inverted, the clamps are removed and the ram up board is removed exposing the pattern, the surface of the sand is smoothed over with slick (trowel)and then covered with a fine of dry parting material (parting sand), the parenting material prevents the sand in cope sticking to the sand in the drag (parting material for large mold is usually fine silica sand and for medium and small molds is finely ground powder such as talc or silica flour. 10. Next, the cope is placed on the drag, sated firmly, and aligned with the aid of drag pins. 11. To provide a place for the molten material to enter the mold a usually tapered pin known as spruce is placed approximately 3 cm to one side of the pattern 12. The operation of the filling, ramming, and venting of the cope proceeds in the same manner as in the drag. 13. At this point, the mold is complete except for the removal of the pattern and the spruce pin. The spruce pin is first withdrawn. Then cope is carefully lifted off to one side. 14. Before the pattern is withdrawn the sand around the edge of the pattern is usually moistened with a swab so that the edge of the mold is firmly together 7 when the pattern is withdrawn. to loosen the pattern. A draw spike is drawn into and rapped lightly in all directions, the pattern can then be withdrawn by lifting the draw spike. 15. Before the mold is closed, a small passage known as (the gate) must be cut between the cavity made by the pattern and the spruce opening. The passage is shallowest at the mold so that after the molten metal has been poured the metal in the gate may be broken off close to the casting. 4.5 Casting Split pattern To facilitate the molding of some components, the pattern, instead of being made in one piece is split into two or more parts. These are dowelled so that they may be fitted together accurately to a finished shape, such an example is given by the flanged pipe shown in figure (6), and its molding would be carried out as follows: 1. Ram up one-half in the bottom box 2. Turnover, fit the half, and ram the top box 3. Remove the pattern and insert the core. Figure 6 8 4.6 Important remarks The behavior of cast metal, when the molten metal is poured into a mold the casting begins too cool inwardly from all bounding surfaces because the heat can flow outwardly through the mold. The metal on the surfaces is more or less chilled because at first the mold is relatively cool if the chilling is served, the surface may be appreciably hardened. Under usual conditions, a fine, close- grained structure occurs near the surface and coarser grains toward the center where cooling is slower. If a section is thick, enough metal may be withdrawn by contraction from the center before it cools to leave a void or cavity, such a defect in the casting may be avoided by providing a supplementary mass of metal, called a riser. The purpose of the riser is to feed liquid metal by gravity into the body of the casting to keep it full. The riser is cut off after the casting has cooled. The mold surface may be sprayed, swabbed, or dusted with a prepared coating material. Such coating often contains silica flour and graphite, but their composition varies considerably depending on the kind of material being cast, A mold coat improves the surface finish of casting and reduce possible surface defects, sometimes before the iron is poured the weight may be put on top to prevent the liquid metal from running out between the cope and drag. 9 10 Chapter Five: Metal Forming Process All elements can be found as solid, liquid, or gaseous, depending on the temperature and pressure surrounding these elements, materials with a specific crystal composition are called crystalline materials. Materials that do not have a crystal composition are called non- crystalline materials or amorphic materials. 5.1 Metal Forming The ability of a metal to accept forming is considered one of the most important manufacturing characteristics, as it enables changes in the metal and transfers it from one form to another without the need for melting. When the metal is stressed past its yield point, it changes shape in a way that is not permanent. 5.2 Cold Working and Hot Working Cold forming, often known as cold rolling, is a forging technique used to shape metal materials at near room temperature. When metal is formed at cooler temperatures, the material's tensile strength stays the same or gets stronger, but it can still be manipulated in very complex ways. Cold forming is a high-speed process that allows manufacturers to produce large amounts of metal-based products in a fast, consistent, and cost-effective manner. When compared to high-heat formation methods, cold formed products are harder, produce greater yield, feature higher tensile strength, and have a superior surface finish. A variety of metals are suitable for cold forming. For example: Alloy Steel (1045, 1117, 1215, 4140, 4150, 4340, 8620, H-11), Stainless Steel (316, 416, 17-4), Aluminum (2024, 6061), Other materials are simply too brittle for this process, such as cast iron. Hot forming is a process of sheet metal forming that is also known as hot stamping or press hardening. All forming processes run above the recrystallization temperature of the metal used. During the hot forming of the sheet metal, the material recovers and softens. This enables high equivalent strain despite low forming forces. Hot forming includes several processes, such as forging, hot rolling, and extrusion. 1 5.3 Rolling Rolling is a metal forming process in which metal stock is passed through one or more pairs of rolls to reduce the thickness, make the thickness uniform, and/or impart a desired mechanical property. The concept is similar to the rolling of dough. Rolling is classified according to the temperature at which the metal is rolled. If the temperature of the metal is above its recrystallization temperature, then the process is known as hot rolling. If the temperature of the metal is below its recrystallization temperature, the process is known as cold rolling. Rolling mills are either two- high, four -high, or six- high, or they contain a larger number of rolls. In addition, there is a supportive roll that prevent the working roll from bending during the rolling process as illustrate in Figure 5.1 Figure 5.1: Rolling process for a hot metal, and the rolling types 2 5.3 Forging Forging is a way of forming a metal, in which a metal is shaped by hammering or pressing. These compressive forces are applied to the metal with a tool such as a hammer. Forging is often categorized according to the temperature at which it is performed—cold, warm, or hot forging. A wide range of metals can be forged. Typical metals used in forging include carbon steel, alloy steel, and stainless steel. Very soft metals such as aluminum, brass, and copper can also be forged. 5.3.1 Open-die forging (smith forging) Open-die forging is also known as "smith forging." A hammer strikes and deforms metal on a stationary anvil (as shown in Figure 5.2). The operator is in charge of putting the metal in the right place and direction to get the desired final shape. Flat dies are used, with some having specially shaped surfaces for specialized operations. Open-die forging is suitable for simple and large parts, as well as customized metal components. Figure 5.2: Open die forging The advantages of open-die forging are: better fatigue resistance and strength, reduces chance of error and/or holes, improves microstructure, continuous grain flow, finer grain size 3 5.3.2 Closed-die forging (impression-die) Closed-die forging is also known as impression-die forging. The metal is placed in a die and attached to an anvil. The hammer is dropped onto the metal, causing it to flow and fill the cavities. The hammer is timed to come into contact with the metal in quick succession on a scale of milliseconds. Excess metal is pushed out of the die cavities, resulting in flash. The flash cools faster than the rest of the material, making it stronger than the metal in the die. After forging, the flash is removed. (as shown in figure 5.3) The advantages of closed-die forging are: produces parts up to 25 tons, produces near net shapes that require only a small amount of finishing, economic for heavy production. Figure 5.3: Closed die forging 5.4 Metal Extrusion Metal extrusion is a forming process in which we force metal (either hot or cold) through a die. This imparts the die shape to the extruded metal as it passes through the cavity. The material emerging from the die is known as " extrudate" The metal endures compressive and shear stress to achieve the die shape. Because of the way these forces work and the high temperatures, this process can be used to shape materials that would normally be brittle. Extrusion can also be done with nonmetals like ceramic, plastic, clay, concrete, polymers, etc. 4 Extrusion is a low-cost process due to reduced wastage and has a high rate of production, it can form brittle materials as it applies only compressive and shear forces on the billet, the resultant products have an excellent elongated grain structure in the material direction, the products also have a smooth surface finish that reduces the amount of post-treatment, extremely thin wall thickness can be achieved through extrusion (~3mm for steel and ~1mm for aluminum), extrusion can create extremely complex cross-sections with a uniform wall thickness throughout the product. 5.4.1 Direct extrusion Direct extrusion uses a stationary die while the ram creates pressure on the fluid metal. Due to this pressure, the fluid escapes through the die opening. The die opening is in the container on the opposite side of the punch. Thus, the extruded part comes out in the direction of the ram motion. This is why it is also known as "forward extrusion." Shown in figure 5.4. 5.4.2 Indirect extrusion In indirect extrusion, the die opening is on the ram. As the punch compresses the fluid metal, it flows through the die opening in the opposite direction of the ram. This is why it is also known as "backward extrusion". Shown in figure 5.4 Figure 5.4 Direct and Indirect Extrusion 5 5.4.3 Impact extrusion In impact extrusion, the punch is brought in contact with the billet at a high speed. The resultant pressure pushes the extrudate out through the clearance between the punch and the die. This die cavity gives the extrudate the required shape. Once the punch is lifted the extrudate rises with it and is stripped from it using a stripper plate. (Figure 5.5) Impact extrusion is based on cold extrusion and usually forms softer metals such as aluminum, copper, and lead. Figure 5.5: Impact extrusion 5.5 Drawing forming process Drawing is a metalworking process that uses tensile forces to elongate metal. As the material is drawn (pulled), it stretches and becomes thinner, achieving a desired shape and thickness. Drawing is classified into two types: sheet metal drawing and wire, bar, and tube drawing. Sheet metal drawing is defined as a plastic deformation over a curved axis. For wire, bar, and tube drawing, the starting stock is drawn through a die to reduce its diameter and increase its length. Drawing is usually performed at room temperature, thus classified as a cold working process; however, drawing may also be performed at higher temperatures to hot work large wires, rods, or hollow tubes in order to reduce forces. Drawing differs from rolling in that pressure is not applied by the turning action of a mill but instead depends on force applied locally near the area of compression. This means the 6 maximal drawing force is limited by the tensile strength of the material, a fact particularly evident when drawing thin wires. Drawing Forming Process type: Wire drawing (Figure 5.6) Tube drawing (Figure 5.7) Deep Drawing (Figure 5.8) Figure 5.6: The wire drawing process Figure 5.7: Tube drawing Figure 5.8 Deep Drawing 7 Chapter Six: Metal Machining Forming The main goal of metal machining is to get parts of products with the right size and shape. This is basically done by removing layers of metal using a tool called a cutting tool. The layers removed from the surface of the mineral are called chips. The operation, if done by using manual tools, is called a manual operation. If the operation is done by using machines, it is called an operation by automatic machines. 6.1 Machining by hand tools There are many machining operations that can be carried out by many simple manual machining tools, but certain conditions must be met, the most important of which are: choosing the appropriate machining hand tool, for example, the hardness of the hand tool is higher than the hardness of the piece to be machined. Specifying and marking the required dimensions correctly on the piece to be machined and ensuring that the work steps are organized in the correct sequence 6.1.1 Filing Filing is a material removal process in manufacturing. Similar, depending on use, to both sawing and grinding in effect, it is functionally versatile, but used mostly for finishing operations Parts of a File It has the following main parts of a file as shown in figure 6.1: Figure 6.1: Parts of File 1 1. Handle 2. Tang 3. Heel 4. Face 5. Edge 6. Tip or Point A wooden handle is fitted on its tang. Teeth are cut on its face and edge so that it can perform the job of cutting. The part having no teeth is called as heel. Thick side of file is called edge and its lower end is known as tip or point. Types of File Tools While making a selection of file for a specific job, some important points should be considered. In other words, we can say that files can be classified into the following categories: 1. On the basis of length A file is measured according to its length. This length includes whole file except its tang portion i.e. length from heel to tip is the measurement of the file. In fitter trade, file of 100 mm to 450 mm length are used. 2. On the basis of shape (as shown in figure 6.2) Flat file: his file is of rectangular cross-section in shape. It is made slightly tapered both in width and thickness. Double cut teeth are cut on the face and simple-cut teeth are cut on the edge. These are used to reduce flat surface by filing of finishing. Round file: This type of file has a round section. It is used for rubbing or finishing keyhole of small diameter. Half-round file: It is a file which is on the side and curved on the other side. Double cut dents are cut on it and like a round file, it is also tapered. With this file, the damaged hole can be set in order again. Triangular file: It is triangular in shape. Its angles are of 60°. Filing of “V” slots job, square and rectangular jobs of above 60° and below 90° angle are done with this file. It is also known as three square files. Square file: It is square in shape and is somewhat tapered. Filing of rectangular, square groove, slots and key-way is done with this file. Hand file: This file is almost similar to the flat file. It is used for filing of internal right angle side of a job. It is also called safe edge file. Knife file: Its shape is like the edge of a knife. Its thin edge is about 10° angle. It is used for filing small grooves and slots whose angle is less than 60°. It is generally used in the lock industry for making keys. 2 Figure 6.2: file based on shape 3. In the basis of grades (Figure 6.3) Rough File: In this type of file, teeth are of big size and less in number. It is used for cutting soft materials because its cutting is rough. Because of rough cutting, it cannot be used for hard metal. Bastard File: Teeth of this file are comparatively smaller than those of the rough file. It is used initially to shed metal (through filing) in large quantity. Second cut File: This is a file of medium grade. In the filler trade, this file is mostly used to bring the job in the proper size. As compared to the bastard file, filing done with this file makes the surface plain. Smooth File: This file makes the surface quite plain, in addition to making a job of accurate size through filing Dead Smooth File: Its teeth are very close to each other and it rubs off the metal in very little quantity. It is used for bringing shinning on the job after finishing has been done. 3 Figure 6.3: file based on grades 4. On the basis of cut Single Cut File: This is file has parallel lines of teeth running diagonally across its face in one direction only. Metal surface is rubbed in little quantity and thus the surface becomes smooth. Therefore, it is used for hard metal and for finishing. As shown in figure 6.4-A Double Cut File: This file has two rows of teeth crossing each other at an angle of 40° to 45° and the other row has angles of 70° to 80°. Because of double teeth, this file cuts metal quickly but it cannot make the surface very smooth. That is why it is generally used for normal filing as shown in fig 6.4- (B). It is also called a coarse type file. In 30° to 35° and the other row is of 80° to 87° angles as shown in fig 6.4- (C). Curved Cut File: It is also known as Vixen file. The curved-cut file is used for filing of the wide surfaces of soft metal like aluminum, zinc, copper and brass. It has circular teeth as shown in fig 6.4- E. Spiral Cut File: These types of teeth are cut in round or semi-round files. The shape of their teeth is like that of threads. Rasp Cut File: This is a file of special thick teeth. These teeth are of triangle shape and are in a bulging state. It is used for jobs of woods, plastic, fiber, hard rubber, and job of horns and hoof of animals. 4 Figure 6.4: File based on cut 6.1.2 Sawing In the process of sawing, a tool called a saw blade with a row of closely spaced teeth cuts a narrow slit into the work piece. Sawing is used to separate work parts into two or more pieces or to cut off an unwanted section of a part. These processes are often called cut-off operations and since many manufacturing projects require cut-off operations at some point in the production sequence, sawing is an important manufacturing process. Basically, sawing is a simple process: As the blade moves past the work, each tooth takes a cut. Depending on the thickness or diameter of the work, the number of teeth cutting at one time varies from two to ten or more. Saws may be of the continuous cutting (band or rotary) or reciprocating type. All saw blades have certain common characteristics and terminology: Rake angles: Rake angles are 0 degrees or neutral rake on most saw blades. Some have a positive rake angle. Width: The width of a saw blade is its total width, including the teeth. Set: The set of a saw blade means the offsetting of some teeth so that the back of the blade clears the cut. The "raker" set is most frequently used and is furnished with all hacksaws and band saws unless otherwise specified. Kerf: The kerf is the width of the cut made by the saw blade or the material cut away. The thickness of the blade is called the gage. Pitch: The pitch of a saw blade is the distance between the tops of two adjacent teeth. This is specified in teeth per inch. The most famous saw blade materials are Carbon steel, High-speed steel (HSS), Tungsten carbide-tipped blades 5 Figure 6.5: The shape of the teeth in the blade 6.1.3 Chiseling Chiseling (as shown in figure 6.6) is a cutting operation involving a hand tool known as a chisel. This operation is quite common in woodworking (carpentry) to give a proper shape to the wood. However, the chiseling operation is also frequently used in shaping metals (Metalworking) and stones/bricks (Masonry). The cutting tool, Chisel, is a metallic blade with a sharpened edge. In the chiseling process, the blade is forced into the work piece to cut/shape it. Figure 6.6: Chiseling 6 Following are the different parts of a chisel (Figure 6.7): Head Body Forging angle Cutting edge Cutting angle: The angle at which a cutting edge of chisel is grinded is known as its cutting angle. For cutting hard metal, the cutting angle is more and for cutting soft metals the cutting angle is less. While grinding chisels for cutting various kinds of metals their angles should be as under: Mild steel – 55 °, Cast iron – 60 °, Tool steel – 65 ° to 70 °, Copper – 45 °, Aluminum – 30 °, Brass – 50 ° Figure 6.7: part of chisel Following are the different types of chisel (figure 6.8 illustrate it): Flat chisel: In these types of chisel, it is an all-metal chisel used for obtaining a flat surface on metal by chipping. This chisel is used to cut wires, thin sheets and round rods etc. The edge of this chisel is in “V” shape and is flat, which is raised from the center. Because of this projected parts, it does not get stuck into the cut surface, while chipping. Cross cut chisel: It is mainly used to cut square slots and channels. Keyway on a shaft is made with this chisel. Its cutting edge is from 3 mm to 12 mm in width. In order to ensure that this chisel does not get stuck into the grooves and slits while using it in the grooves and slits, the part behind its edge is made comparatively thin. 7 Side cut chisel: Where other types of chisel cannot be used easily, side cut chisels are used. The keyway and cotter way is cleaned with this chisel. It is almost similar to the flat chisel but the part which performs the function of cutting is turned to one side. It is turned at an angle and then forged. Its cutting angle is generally at and forging angle at an angle. Round Nose Chisel: Its edge is round. It is used for making an oil groove in a bearing and for cutting slits at corners. Its forging angle is at and cutting angle is of 35 ° to 40 °. In such jobs where the drill is dislocated at the time of drilling and some substance is left, the leftover metal is set right with this chisel. Diamond point chisel: This is made of good quality steel. Its shape is square and it is tapered. Its edge is pointed and of the shape of a diamond. On the basis of its square ends, its measurement is determined. Square corners and triangle corners are cleaned with it. We can make a groove in plain surface with this chisel. Cow mouth chisel: In these types of chisel, its shape is like that of the broad mouth of a cow. That is why it is known as cow mouth chisel. Edges of the protruding parts of plain metal and rivet heads and big round holes are made with it. Its forging angle is at an angle. Figure 6.8: different types of chisel 8 The following points should be kept in mind while using a chisel: 1 Accurate marking should be done on the metal to be cut. 2 Always hold the chisel properly with your left hand and the hammer in your right hand. 3 Set the chisel properly on the marked line, then strike the hammer on its head. Before striking the second blow on the chisel, it should be set again. 4 While cutting with a chisel, we should concentrate on its cutting edge. 5 Strike the hammer blow at the center of the head of the chisel. 6.2 Machining by machine tools Machine by machine process uses tools such as lathes, mills, and drill presses to turn material into useful tools using precise cuts. There are some common definitions to automation machine process: Cutting speed is defined as the speed at which the work moves concerning the tool (usually measured in feet per minute). Feed rate is defined as the distance the tool travels during one cycle of the part. Cutting speed and feed determine the surface finish, power requirements, and material removal rate. The depth of cut is the distance that the tool bit moves into the work. usually measured in thousandths of an inch or millimeters. 6.2.1 Turning Turning is a machining process in which a cutting tool moves more or less linearly while the work piece rotates. (figure 6.9) Figure 6.9: Turning process 9 6.2.2 Drilling A drilling machine is one of the important machine tools in the workshop. Drilling is a material-removing or cutting process in which the tool uses a drill bit to cut a hole of circular cross-section in solid materials. This is the most common machining process; one estimate is that 75% of all metal cutting material removed comes from the drilling operation. The drill tools in this process use two types of movement: rotational movement (cutting movement) and axial movement (feeding movement), and the work piece remains stationary during the cutting process. (figure 6.10) Figure 6.10: Drilling 6.2.3 Shaping The cutting tools here used in both operations are single-edge cutting tools to create a straight and flat surface. (Figure 6.11) These machines are limited to low speeds due to their start-and-stop motion. High-speed steel cutting tools are recommended. 10 Figure 6.11: Shaping machine 6.2.4 Griding Machines used to grind in manufacturing are called grinders, or grinding machines (see figure 6.12). Both terms refer to the same device. A grinding machine uses an abrasive product — usually a rotating wheel — to shape and finish a work piece by removing metal and generating a surface within a given tolerance. A grinding wheel is made with abrasive grains bonded together. Each grain acts as a cutting tool, removing tiny chips from the work piece. 11 Figure 6.12: Grinding machine process 6.2.5 Milling Milling is a machining method in which a milling cutter is used as a tool to process the surface of an object. Milling is a highly efficient machining method in which a rotating multi-blade tool is used to cut a work piece. Milling refers to a technique of moving the relative position of a work piece, such as metal, to a tool while cutting or drilling a hole in the material to give the material the desired shape. When working, the tool rotates as the main movement, the work piece moves as the feed movement, and the work piece can also be fixed, but the rotating tool must complete the main movement and the feed movement at the same time. (Figure 6.13) Figure 6.13: Milling machine process 12 Chapter Seven: Joining of materials Joining includes welding, brazing, soldering, and adhesive bonding of materials. This process produces a permanent joint between the parts to be assembled. Which means that they cannot be separated easily by the application of forces. 7.1 Welding Welding is a metal joining process in which two or more parts are joined or coalesced at their contacting surfaces by suitable application of heat or/and pressure. Sometimes, welding is done just by applying heat alone, with no pressure applied In some cases, both heat and pressure are applied; and in other cases only pressure is applied, without any external heat. In some welding processes a filler material is added to facilitate coalescence. Advantages of welding: Welding provides a permanent joint. Welded joint can be stronger than the parent materials if a proper filler metal is used that has strength properties better than that of parent base material and if defect less welding is done. It is the economical way to join components in terms of material usage and fabrication costs. Other methods of assembly require, for example, drilling of holes and usage of rivets or bolts which will produce a heavier structure. Disadvantages of welding: Labor costs are more since manual welding is done mostly. Dangerous to use because of presence of high heat and pressure. Disassembly is not possible as welding produces strong joints. Some of the welding defects cannot be identified which will reduce the strength Types of welding: Welding processes can be broadly classified into fusion welding, and solid state welding. 7.2 Fusion welding: In fusion-welding processes, heat is applied to melt the base metals. In many fusion welding processes, a filler metal is added to the molten pool during welding to facilitate the process and provide strength to the welded joint. When no filler metal is used, that fusion welding operation is referred to as an autogenous weld. Types: Arc welding, Resistance welding, Oxyfuel gas welding, electron beam welding, laser welding. 1 7.2.1 Arc welding In this operation, electric arc is used to produce heat energy and the base metal is heated. Sometimes, both pressure and heat are applied (Figure 7.1) Figure 7.1: Arc welding steps An electric arc is generated when the electrode contacts the work and then quickly separated to maintain the gap. A temperature of 5500°C is generating by this arc. This temperature is sufficient to melt most of the metals. The molten metal, consisting of base metal and filler, solidifies in the weld region. In order to have seam weld, the power source moves along the weld line. Arc Weld Parts: Electrodes Two types of electrodes are used: consumable and non-consumable Consumable electrodes: Present in rod or wire form with 200 to 450 mm length and less than 10 mm diameter. This is the source of filler rods in arc welding. The electrode is consumed by the arc during the welding process and added to the weld joint as filler metal. The consumable electrodes will be changed periodically as it is consumed for each welding trial. This becomes a disadvantage for welders and reduces the production rate. 2 Non-Consumable electrodes: The electrodes are not consumed during arc welding. Though this is the case, some depletion occurs because of vaporization. Filler metal must be supplied by means of a separate wire that is fed into the weld pool. Arc shielding: Shielding gas: This covers the arc, electrode tip, and weld pool from the external atmosphere. The metals being joined are chemically reactive to oxygen, nitrogen, and hydrogen in the atmosphere. So the shielding is done with a blanket of gas or flux, or both, which inhibit exposure of the weld metal to air. Common shielding gas: Argon, Helium. Flux: Used mainly to protect the weld region from the formation of oxides and other unwanted contaminants, or to dissolve them and facilitate removal. During welding, the flux melts and covers the weld region giving protection and it should be removed by brushing as it is hardened. Additional function, other than giving protection: stabilize the arc, and reduce spattering. Power source in arc welding: Both AC and DC can be used; DC is advantageous as better arc control is possible. Polarity: Straight polarity in which work piece is positive and electrode is negative is suitable for shallow penetration (like in sheets) and joints with wide gaps. Reverse polarity in which work piece` is negative and electrode is positive is suitable for deeper welds. 7.2.2 Resistance welding In this operation, electric resistance is generated to the flow of current that generates heat energy between two contacting surfaces that are held in pressure. (Figure 7.2) Weld nugget is generated by this process. Resistance Welding uses no shielding gases, flux, or filler metal, electrodes that conduct electrical power to the process are non-consumable Figure 7.2 Resistance welding 3 7.2.3 Gas welding oxyfuel gas welding is a welding operation in which heat is generated by a hot flame generating a mixture gas of oxygen and acetylene. This heat is used to melt base materials and filler materials, if any are used. (Figure 7.3) Figure 7.3: Gas welding In this process, various fuels are mixed with oxygen and burnt to perform welding such as: Oxyacetylene welding Oxyacetylene welding (OAW): In this case, welding is performed by a flame formed by the combustion of oxygen and acetylene. The flame comes from a torch. A filler rod coated with flux is used sometimes which prevents oxidation, creating a better joint. Acetylene is a famous fuel because it is capable of generating a temperature of 3500°C. Problems and advantages of OAW: 1. The combination of acetylene and oxygen is highly flammable and hence hazardous to environment. 2. It is unstable at pressures much above 1 atm. 3. It is mandatory for the welder to wear gloves, goggles etc. as preventive measures. 4. The equipment is relatively cheap and portable. So it is used as an economical, versatile process that is well suited for low quantity production and repair jobs. 5. It is rarely used to weld plates thicker than 6.5 mm. 7.3 Solid State Welding In this method, joining is done by coalescence resulting from the application of pressure only or a combination of heat and pressure. Even if heat is used, the temperature in the process is less than the melting point of the metals being welded (unlike in fusion welding). No filler metal is utilized. A metallurgical bond is created with little or no melting of the base metals. To metallurgically bond two similar or dissimilar metals, the two metals must be brought into intimate contact so that their atomic forces attract each other. 4 The two surfaces must be cleaned and free of oils, dirt, chemical films, gases, etc. to permit atomic bonding. Some of the solid-state welding processes are applicable to joining dissimilar metals, without concerns about relative thermal expansions, conductivities, and other problems that usually arise when dissimilar metals are melted and then solidified during fusion welding. 7.3.1 Diffusion welding Two part surfaces are held together under pressure at an elevated temperature, and the parts join by solid-state diffusion. (Figure 7.4) Figure 7.4: Diffusion welding Roll welding or bonding Two or more sheets are kept one above the other and rolled to generate bonding between them. If done without application of external heat, then it is called cold roll bonding. With the application of external heat, it is called hot roll bonding. (Figure 7.5) 7.5 Roll welding or bonding 5 7.3.2 Friction welding/Stir welding Joining occurs by the heat of friction and plastic deformation between two surfaces.(Figure 7.6) Figure7.6: Friction /Stir welding 7.3.3 Ultrasonic welding Moderate pressure is applied between the two parts and an oscillating motion at ultrasonic frequencies is used in a direction parallel to the contacting surfaces. (Figure 7.7) Figure7.7: Ultrasonic welding 6 Weld joint configurations Figure 7.8: Weld Joint Types Figure 7.9: Fillets weld Figure 7.8: Weld Joint configuration 7