Calcium-Mediated Signal Transduction in Plants PDF
Document Details
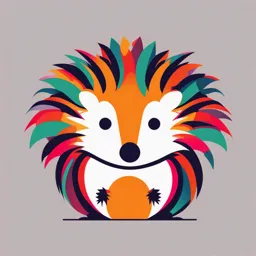
Uploaded by RoomierFallingAction5659
University of Padua
Navazio
Tags
Summary
This document discusses calcium-mediated signal transduction in plants. It explores how plants react to environmental stimuli, converting them into specific responses via calcium signaling. The document also describes various methods for measuring intracellular calcium concentration in plants, including using fluorescent dyes and genetically encoded calcium indicators.
Full Transcript
CALCIUM-MEDIATED SIGNAL TRANSDUCTION IN PLANTS Calcium is one of the most important intracellular messengers, and that is why we need to know about its homeostasis, that is regulated by a complex machinery, its variation during signal transduction, the specificity in this process and how decoding wo...
CALCIUM-MEDIATED SIGNAL TRANSDUCTION IN PLANTS Calcium is one of the most important intracellular messengers, and that is why we need to know about its homeostasis, that is regulated by a complex machinery, its variation during signal transduction, the specificity in this process and how decoding works. Signals can be environmental both biotic and abiotic. These can be chemical or physical factors like temperature, light, salt, drought; while for biotic we mean other organisms that live close to the plants, like pathogens or symbionts. When plants perceive these signals, they convert them to downstream responses thanks to a decoding process of these stimuli that is translated in a defence mechanism or an accommodation strategy depending on the kind of stimulus. And calcium is used for this as an intracellular messenger, but not only in plants, in all living organisms. The calcium signal is given by a transient change in its concentration that is triggered by a stimulus that creates different physiological responses. Usually the variation is given by an increase in calcium concentration. More in detail, stimuli can be: Variations in temperature, like a cold or a heat shock, it can be touch, wind, drought, excess of salt, excess of light or for example a different type of light, like more of a blue or red one. Organisms around it, like pathogens, herbivores that can feed off the plant, symbionts. But why is calcium used this much? We know it’s highly conserved because it’s used in all organisms, also prokaryotes but initially it was a poison for cells, in fact in the cytosol it can induce apoptosis, but also, given that ATP is the energetic currency so it needs phosphates, calcium was dangerous because it could lead to the precipitation of phosphates. At the same time calcium couldn’t be eliminated, and even if it could, when needed couldn’t be produced; the only way to use continue keeping it in the system without it being dangerous was using it for something by keeping it confined outside the cytosol. This of course needed to be done in a very good way, and that’s why there’s a complicated homeostasis machinery and a complicated way in which is used as a signalling molecule. With the movement of few ions evolution managed to do something good. The usual concentration of calcium in the cytosol is 10-7 M in resting condition, while in the apoplast, or outside the cells in general is 10-3 M. When a stimulus happens, transporters are activated and the cytosol fills with calcium that goes up to a concentration of 0.5-5 µM so up to 50 times more in the activated state. Of course we need an off mechanism otherwise a lot of calcium could become dangerous. Organelles are as well a storage for calcium, for example in the vacuole the concentration can be 80 mM, even if it’s not all available. Plastids and mitochondria are important for calcium storage. Calcium goes back and forth inside the cytosol thanks to membrane proteins located in the plasma membrane and in the membranes of the organelles. These proteins can be active or passive systems: They’re passive if they follow it’s gradient in fact they’re called calcium permeable channels and don’t use energy to this. There are 3 main types of channels: 1. Voltage gated channels, that depolarize or hyperpolarize depending on the signal and are located in the plasma membrane, the vacuole and the ER. 1 2. Mechanosensitive channels, that open and close with membrane pressure or tension and are located in the plasma membrane and in chloroplasts. 3. Ligand gated channels, so that need a ligand that binds to the channel in order for it to open or close. The ligands used in the membrane and in the nucleus are cAMP and cGMP; in the membrane, chloroplasts and mitochondria it’s used glutamate; and there are also other possibilities of which scientists are not sure yet that are inositol biphosphate in the vacuole, cADP ribose in the vacuole and ER, NAADP in the ER. They are all activators. They are active when they go against the electrochemical gradient, using energy from ATP to do it. They can be both primary, so Ca2+ ATPases, that pump calcium using a directly ATP; they are located in the plasma membrane, in the ER, vacuole and nuclear envelope. Or they are secondary so they are calcium-proton exchanger: it’s an antiport with protons that diffuse following the gradient, obtaining energy to pump the calcium against it. They are in the plasma membrane, vacuole and thylakoids. It's important to know some methods to measure intracellular concentration of calcium, but also how it changes during transduction because it’s a transient variation of it. We could have both a qualitative measure, so know where the calcium is, and a quantitative measure to know how much is it in a certain part of the cell. One of the first methods is the calcium sensitive fluorescent dye where calcium links to fluorescent molecules. Now what’s more used is protein-based calcium reporters that can be bioluminescent (aequorin protein) or GFP based probes (the most used is a protein called cameleon). Calcium sensitive fluorescent dyes are synthetic molecules that are composed of two parts, a calcium chelator, so that links to calcium (EGTA, BAPTA) thanks to a carboxylic group, and a fluorescent chromophore, that emits the fluorescence. One problem with this is that is difficult to upload the dyes because plants have a cell wall, so we could use glass micropipettes, but we don’t have the guarantee, or we could use protoplasts, but they’re not exactly like the cell because they are missing the cell wall. Also it’s impossible to accumulate the dye in a compartment, it just goes into the cytoplasm and this is another problem. The point of this method is that these molecules change their fluorescent properties when they bind calcium. They can be: 1. Single wavelength calcium indicators (calcium green, Fluo-3). There’s no change in wavelength but in the intensity of the emitted fluorescence. 2. Double wavelength calcium indicators (Indo-1, Fura-2). There’s no change in the intensity but the wavelength changes. We can see both methods for example in the response of stomata when treated with ABA or in growing pollen tubes. The newer technique is the protein based reporters that can be called GECIs, genetically encoded calcium indicators. The first one to be used was aequorin, a bioluminescent molecule that is produced in Aequorea victoria, a jellyfish that also produces GFP. The difference from a fluorescent molecule, is that bioluminescence comes from a chemical reaction and produces photons as a consequence. Aequorin is a calcium protein and emits light when calcium binds to it: it has 3 sites and in order to produce light it needs a prosthetic group that is coelenterazine; when they bind there’s a 2 conformational change that makes coelenterazine be oxidised and become coelenteramide that is then able to emit a photon (and also CO2). In vivo this process is very strange, meaning that when the blue light (470nm) produced by the bioluminescent protein is emitted, GFP gets excited by it and emits a green light (508 nm). The scientists initially extracted the proteins from the jellyfish and injected it in cells but know we use GECIs, so we make the plant express the gene inserting the sequence. The other aspect is that also coelenterazine needs to be inserted because it’s not genetically encoded but it’s very easy because it’s a small hydrophobic molecule that can pass through anything especially the cell wall. So we create the so-called transgenic plants that express our gene of interest but as we said we need to do its reconstruction, so add coelenterazine and this must be done at least the evening before so that the morning after it’s ready to emit the fluorescence. In order to record the photons emitted we use a luminometer, an instrument that let us give the plant or the cell culture a stimulus that triggers calcium and what we see is the blue light emitted that is very precisely detected by a photon counting board. There’s also a software that converts the photons in calcium concentration so that we can also see its variation overtime through a calcium transient. Of course the conversion is made possible by a relationship between these two variables that we can see in the calibration curve: pCa is -log [Ca2+] and we see values from 7 to 5 that mean 10-7 M to 10-5 M, that is the maximum concentration that calcium can reach in the cytosol due to a stimulus, after that we can see that it reaches a plateau. Aequorin in fact works only in this range of concentration but it’s the physiological range so that’s fine for us. Log [L/Lmax] instead is the ratio between the emitted fluorescence and the maximum fluorescence that derives from the process of discharge. This process takes place at the end of the experiment, in order to eliminate all the pool of aequorin by adding a very high amount of calcium and ethanol (disrupts the cells) so aequorin is going to emit all the possible light and gets discharged. By doing this we know that the fluorescence is independent on the experiment, but it’s a general value that we can easily associate with a certain concentration of calcium. 3 Pros Cons There’s the possibility of protein targeting to the different There are potential difficulties is plant transformation, intracellular compartments so we can see calcium not only they can not accept the gene. in the cytosol but also in the compartments. The concentration of calcium probe that we need is much There’s a very low light emission because one aequorin lower than the one required for the dyes and in this way emits only one photon so you’ll always need the we don’t interfere with its homeostasis and they don’t bind luminometer and you need a big population of cells, or a a lot of calcium so there’s less buffering effect. plant, not a single cell. Dyes emit a lot of photons. There’s a high signal-to-noise ratio, so we’re sure that You need to reconstitute the holoprotein a day before so there’s no noise, because there are no other that there’s time for them to create the complex. bioluminescent molecules in the cell. It provides very accurate measurement in a wide range. Aequorin has already been used in many compartments, mitochondria, nucleus, chloroplasts and the tonoplast so the membrane of the vacuole, because the internal part is too acid so calcium probes can’t go inside for now. In order to do this, we need to engineer aequorin chimeras so fuse it with something else usually a nucleotide sequence encoding a specific targeting sequence or proteins localized in a specific compartment. The only problem is that we can’t attach anything to the C-terminus part of the protein because otherwise it would lose its bioluminescent properties. The engineering works like this: In the vacuole they attach the H+ phosphatase to the N-terminus that is a proton pump of the vacuolar membrane. In mitochondria the first 87 aa of the β subunit of F1 of the ATP synthase to the N-terminus. In the nucleus to the N-terminus is attached the nucleoplasmin that contains a nuclear localization signal in the sequence. In chloroplasts to the N-terminus there’s a transit peptide of the small subunit of RubisCO that is encoded by the nuclear genome. The problem comes with the ER, because there’s a signal peptide to the N-terminus but if it goes in, it then follows the secretory path that goes in the Golgi and the lysosomes, so it would be ideal to add a sequence of a retention signal tetrapeptide to the C-terminus, the KDEL (in animals) or the HDEL (in plants). The problem is that if we add it aequorin won’t emit light anymore. To solve this we use an uncleavable ER signal peptide that is not cleaved after it enters the ER so it doesn’t receive the signal that it needs to exit. There’s also the need to add a mutation to the aequorin so to decrease calcium affinity. The calcium transient is generated by the calcium-permeable channels, the pumps and the exchangers that work in combination to the calcium inside and outside the cell when the stimulus happens and when it’s done; the stimulus can for example be the injection of cold water that makes the cell go from 20° to 5°. It’s described by kinetic parameters: The lag phase, the time needed by the calcium to come into the cytoplasm after the stimulus; it usually takes some seconds. The value of the peak, that depends on the stimulus, its intensity and duration. The timing of the peak, so how long it takes and it’s usually in minutes or hours. 4 The total duration of the transient that at the end needs to go back to null because it’s otherwise toxic for the cell. Another GECI is based on fluorescent proteins like GFP so can be GFP-based calcium indicators; given that they don’t bind to calcium we also need something else. The first indicator that was created was the cameleon that derives from the calmodulin (CaM) that is a calcium binding protein and also it changes colour like a chameleon. This protein uses the FRET technique (fluorescence resonance energy transfer): when the calmodulin binds calcium, it changes its conformation so they link to a target peptide and to two variants of the GFP, the CFP (cyan FP) that emits blue light and the YFP (yellow FP), yellow light. When CaM and calcium bind CaM wraps around the peptide bringing the two FPs close to each other and because of FRET we see that in low calcium the light emitted is blue, but in high calcium they get close to each other and the emitted light is yellow. With this technique we get a very good imaging (using the fluorescent microscope), but only on where the calcium is, not on his concentration, so the methods can be used depending on what’s the goal of the experiment. Another type that uses the GFP specifically is the GCaMP, that contains only that and the calmodulin; with high calcium there’s light because it’s bound to the CaM so it’s calcium dependent. We can use them also at low magnification and see how calcium moves around in an entire organ for example. There were two important experiments about this: the first was made on an insect, that sucks the floem from leaves and when it does there’s a calcium response in the exact point in which it happened. The second is more large scale, because it’s with a caterpillar, so that bites the leaf and the calcium transient is not localized anymore, but it’s a systemic response, so it spreads all over the plant giving the signal that something dangerous it’s happening and activating a defense mechanism. They thought it could be enzymatic (something in the saliva) or mechanical, and so it was because they tried cutting a leaf with a scissor and the result was the same, even if the consequence is at a chemical level. Another indicator is the GECO, genetically encoded calcium indicator for optical imaging. It’s based on a single fluorescent protein that can be of different colours so that you can use more than one at the same time but in different compartments in order to distinguish between calcium signals that come from different parts. For example when there’s a symbiotic event in root airs both in the nucleus and in the cytosol there’s a calcium signal. The only thing that is not clear is how the signal is propagated, because it could be by the floem, but some think it could also be thanks to ROS molecules. 5 We must say that calcium singals are stimulus specific because they are so many so they need to be recognized and this happens thanks to the calcium signature that is encoded by the cells depending on the different characteristics that every stimulus brings: they can have a different lag phase, rise time (how long it takes to reach the peak), total transient length, shape, but also the transient can happen in different places of the cell, for example if different organelles are involved, the origin of the calcium can be different, for example if it’s an influx in the cytosol, or from the ER, the vacuole or mitochondria, there can be different pumps, permeable channels and exchangers involved. They can also change in duration an amplitude, bceause it could be long or short, and the gradient of calcium concentration can vary a lot. There are also a lot of specific signals, so that have a specific oscillation in the transient and we call it calcium spiking and it can happen for example when a plant interacts with a symbiotic organism and the transient changes depending on the organism so the plant recognizes which is it. It’s not always an increase in all the cytosol, there can be microdomains in specific locations where the concentration of calcium changes, for example close to the mouth of the protein pump or close to an organelle. In fact we can say that there is also a plant organellar calcium signalling, so where organelles are involved and each of them has a specific calcium transient that can be recognized, but a really cool thing that they can do is fine-tune the transient because they can be involved in the flux but they can also store calcium so they can also dissipate the signal. For example, in chloroplasts it’s important for the metabolism and a lot of other processes like the import of proteins and plastids division; recently a new channel was found that is usually in mitochondria and it’s related to the perception of osmotic stress, in particular drought. How does the plant interpret these messages? With calcium binding proteins, called specifically calcium sensors that can bind calcium and interpret the signal. The calcium binding site is called EF hand so there’s a structural domain that is helix- loop-helix, so two α helixes and a loop in between that is rich in glutammate and aspartate that are acidic amminoacids so negatively charged and that’s here where calcium binds. The topology recalls that of a hand. E and F stand for the first amminoacid of the helix that is E = glutammate, F = phenylalanine. The binding site has high affinity for calcium so it’s very specific and binds it at a very low concentration. After the binding there’s a conformational change in the protein so that it can interact with the downstream effectors and target proteins that deliever the message. 6 Calcium sensor proteins can be: 1. Sensor relays, that don’t have any enzymatic activity so they just transit the original signal to another protein that has enzymatic activity. 2. Sensor responders, they combine in the same protein both the calcium binding and the enzymatic activity and for now all the known proteins are kinases. Both give a physiological response at the end. Sensor relays can be of two kinds: 1. The calmodulin (CaM), that is a small acidic protein (150 aa) with 4 EF hands positioned at the extremities in a structure of a dumbell. This protein is highly conserved in all organisms (plants share a 90% identity with vertebrates) and it regulates target proteins by interacting with them; in Arabidopsis there are 7 CaM genes. When CaM binds calcium it undergoes a conformational change that exposes hydrophobic regions that are then recognized by the target proteins. 2. Calmodulin-like proteins (CMLs), are similar to CaM in fact they derive from the same ancestral but can share from 16% to 75% of identity in fact they can have a different number of EF sites (1,2,4,6) and they don’t have any other domain; in Arabidopsis there are 50 isoforms. Both have a very large number of protein targets, kinases, phosphatases, transporters but also transcriptional factors. Sensor responders can be: 1. Calcium-dependent protein kinases (CDPKs) that have enzymatic activity in fact they phosphorilate the target protein. They are present only in plants and protists, 34 isoforms in Arabidopsis that are activated by different stimuli and are in different compartments of the cell. They contain a CaM like domain so 4 EF hands and a kinase domain with the 4 EF hands, then the initial variable sequence is for membrane association so that it can attach to the different compartments and know where to do it. But the most strange part is the junction, that is an autoinhibitory domain so when the cell is in resting condition it blocks the active sites, but when calcium binds because its concentration increases the kinase is activated. 2. Calcineurin B-like proteins (CBLs), are proteins that are similar to the B subunit of calcineurin in animals; this proteins are phosphatases to regulate calcium and are composed of two subunits, A that is catalytic, B regulatory so actually in plants we have just the B subunit so it shouldn’t be consider a responder, but a relay because there’s no enzymatic activity. But they specifically interact with another family of proteins that is the CBL- interacting protein kinases (CIPKs) and they are always bound to them so this forms the CBL-CIPK complex that is 7 a bimolecular sensor responder and becomes active only when calcium binds. There are 10 isoforms of CBLs and 26 of CIPKs in Arabidopsis, located in different parts of the cell. 3. Calcium/calmodulin dependent protein kinases, they have a double dependence on calcium, it can bind directly to EF hands or indirectly to CaM. It’s similar to CPDKs but there are 3 EF hands and a CaM binding domain that overlaps with an autoinhibitory domain that blocks the functioning of the protein. The protein needs both the direct and indirect bond to activate the kinases activity. The interesting thing is that there’s only one isoform of this protein but not in Arabidopsis, in fact it’s related to symbiosis and Arabidopsis can’t establish symbiosis. 8 PHYTOHORMONES They are hormones of plants, so phytohormones that are involved in the regulation of growth and development that requires efficient communication so we need a chemical messenger to do it, and hormones are the perfect fit. Plant hormones are natural substances that even at small concentrations can influence plant physiological processes, specifically they regulate cell activity like division, elongation, differentiation, pattern formation, organogenesis, reproduction, and responses to abiotic and biotic stresses. Hormones are different between plants and animals: Animals Plants They have specific production sites. Production sites aren’t specific, so they can be synthetized in many different organs. They specific target an organ or a Their function is not limited to a single process but they can reach many events cell type. and many organs, very distant cells, close ones or the same cell as well. They are involved in specific For different concentrations of the same hormone there can be different processes in a precise location of physiological responses, because the same hormone is involved in many the cell. processes; at the same time the same process is affected by a combination of homones, that can be sinergical or antagonistic. They are synthesized, then transported, perceived by a receptor to which they bind so to activate a signal transduction and to create the biological response. The main hormones are auxins, gibberellins, cytokinins, abscisic acid and ethylene, that were discovered some time ago and we know almost all about them, but there are also some new molecules, like brassinosteroids, salicylates, strigolactones and jasmonates. 9 AUXIN They were the first to be discovered even if they weren’t looking for them because they didn’t even know hormones existed at all; they were studying phototropism, so the fact that plants grow oriented towards light. These studies were made on coleoptiles of cereals, so a structure that can be found right after germination and protects the young leaves; this structure it’s particularly sensitive to light so it was useful. Essentialy they heated the coleoptile with a unilateral light and they noticed that it bent in that direction. If they cut the tip of the coleoptile there was no bending because there wasn’t the site of perception of light and the same thing happens if we put an opaque cap on the tip. So we can conclude that the apex of the coleoptile has a light perception site and probably a response site right under it and there’s this signal travelling in the plant that controls growth. They also tried the same experiment but with a MICA sheet that is an impermeable substance that can be layered in more or less sheets so to modulate the action, but it can also be inserted partially, and that’s what they did, they inserted it on the light side and there was a curvature, on the dark side and there wasn’t. If instead they used a gelatin block, that is permeable, the curvature happened anyways meaning that this substance was moving inside the plant. So they analysed some tips and identified this molecule that was involved in growth and gave a phototropic response. Auxins are a group of phytohormones, so there are many kinds, but the main is the indole-3-acetic acid, IAA, that is also the most abundant; the other have a similar structure and some were also artificially synthetized by us and they are used in the lab in order not to take the one coming from the plants. They all have in common the fact that they have a partial charge separation that is at the specifc distance of 0.5 nm and this is what makes it possible to interact with the receptors. Auxins are synthetized in rapidly dividing and growing tissues, in particular the shoot apical meristem, but also in young leaves, developing seeds, something is produced by the roots. 10 The biosynthetic pathways are usually 3 in plants, plus one that is found in bacteria but it’s still similar to the others; they all start from the aa tryptophan: 1. TAM: tam stands for tryptamine that is an intermediate and this reaction happens with a decarboxylation, a demmination and the final oxydation. 2. IPA: ipa stands for indole-3- pyruvic acid, and in this case the first two reactions are inverted. 3. IAN: ian stands for indole-3- acetonitrile and doesn’t have the final oxydation like the others. 4. IAM: is the one found in bacteria, specifically Agrobacterium when it infects the plants and has indole-3- acetamide as the intermediate. All the pathways are active in different tissues. These are the canonical pathways but they actually found a pathway that was tryptophan independent; they found it in a mutant of corn that couldn’t produce any Trp, but still produced IAA. The pathway started from a precursor of indole that is indole-3-glycerol phosphate, then it’s tranformed into indole, then IPA and finally IAA. The metabolism of auxins involves also a conjugation of the molecules in order for them to be stored or degraded. When they conjugate to glucose or aspartate and then they are oxidisied it’s irreversible so they are degraded. Instead if they are not oxidisied it’s reversbile, for storage and it happens most of the time because it would be a waste of time and energy to produce them all over again; it can happen with sugars, aa, peptides, glycoproteins, glycans. The transport can happen in two ways: 1. Apolar: it’s common for all hormones and it’s the movement through the floem, it’s a passove flow, quick and for long distances. 2. Polar: it’s unique for the auxins and essentialy it’s a directional, oriented transport that goes from the shoot apex to the root apex and it happens in the cell of the parenchima of the xylem, because the xylem is composed of dead cells so it couldn’t go through. There are two different transports, the basipetal, that happens from the shoot towards the base, while in the roots it’s acropetal, so it goes from the base to the roots. This helps create a gradient of auxins, but it’s slow and requires energy, but the gradient is fundamental for many processes. 11 Let’s see the experiment made to understand how this polar transport works: donor-receiver agar block → we have a piece of coleoptile where A stands for the apex and B for the base, and we insert it into two agar blocks, one is the receiver, but the other is the donor, that is radioactive, so everytime that auxins move we can see them through autoradiography. 1. The receiver becomes radioactive because auxins move from the donor. 2. The receiver is not radioactive, so it doesn’t receive auxins and this means that probably it follows gravity. 3. If we change the direction of the coleoptile, the receiver is not radioactive. 4. In this case it’s radioactive even if the direction is the opposite, but also the blocks are inverted and this means that it doesn’t follow gravity, but on the other hand it follows a specific direction, so from shoot apex to root apex, helped by proteins that make it follow it. When auxins accumulate, adventitious roots are produced and we can see that this happens always on the root apex because auxins accumulate always in the same place, so at the ending point that is the root apex, even if it’s against gravity. The polar influx happens in the parenchima cells associated with the vascular tissue and given that this is a slightly acidic environment (5 pH) and IAA is a weak acid, 25% of it it’s in the protonated form, so IAAH, that simply crosses the membrane, 75% it’s dissociated as IAA- so we need an influx transporter, that is AUX1, a simporter with 2 protons and it’s positioned in the apical end of the parenchima cells. At this point auxin is inside the cell, where the pH is around 7 so it’s all in the dissociated form so another transporter is needed: the family of the PIN proteins, auxin efflux carrier proteins that are transport facilitators. There are 8 isoforms of PIN, but the most important one is PIN1, at the basal end of the cells and the mutant lacking this protein doesn’t have leaves nor flowers so it’s very important. The polar transport depends on their positioning in fact they are auxin efflux regulators. They work in association with other membrane proteins: ABCB, ATP binding cassette of B class. They are ATP dependent membrane transporters so they’re energy dependent and there are 21 isoforms of them. They can be localized also in the tonoplast because they can store toxic compounds in the vacuole. The ABCB proteins are randomly and uniformely distributed on the plasma membrane but they can colocalize with the PINs in order to regulate the polar transport, in fact when they do there’s an enhanced auxin transport. There can be inhibitors of the auxin transport, that are both synthetic and natural, they were found in some plants and they are essentially flavonoids. They don’t really know how they work, just that probably they block ATP hydrolysis. They can also inhibit protein phosphorylation in fact it can control auxin transport and this was discovered with the PINOID mutant that is essentialy a gene that encodes a kinase; the mutant doesn’t develop much and it’s similar to the PIN 12 mutant so this determines the correct localization of the membrane proteins. Another level of regulation is the protein trafficking, that still regulates the position of the proteins because the PINs are not stable, they are continuously recycled. This was demonstrated with an experiment in which a plant was treated with brefeldin A that alters the vescicolar trafficking and they noticed that the localization of the PINs was different, they go inside the cell in a compartment in the middle of it, but if it’s washed out it goes back to normal. Instead if it’s washed put with blockers of the microfilaments it doesn’t go back because they’re needed to move the vescicles. The process of PIN protein relocalization provides the plant with the possibility to dinamically respond to the environment, meaning that if the proteins are not correctly localized auxin moves in a different direction and the plant grows in that direction. But this is also an advantage because the plant can respond to changes in the environment, for example if light is only on one side, it grows only in that direction. Auxins induce cell elongation, regulating the growth of both the stem and the root and depending on the location and on the concentration it can both have a stimulation effect and an inhibition effect. We can see that for the stem to grow we need a muche higher concentration of auxins, while roots are more sensitive to them and if the concentration is too high there’s inhibition of the growth (different concentrations for stems and roots as well). Growth induced by auxin has a lag phase so it takes 10-15 minutes to make it grow and this doesn’t depend on the plant but probably this tells us that the machinery is already there. If we add sucrose, an hosmolite, to the IAA it can maintain turgor pressure so the growth effect is potentiated. If we observe the elongation rate it’s almost the same for each plant because the kinetic is the same. It’s composed of a first rapid response right after the lag phase, and then there’s a slow response, that starts after we reach the maximum speed and when it stabilizies it remains constant. The rapid response it’s a very high increase in the elongation rate and it happens through the process of acidic growth, so there’s a decrease in the pH of the cell wall in response to the auxin and this has can affect the activity of proteins especially the membrane ATPases that pump protons in the cell wall. It happens because of the machinery that is already there, so there’s no need for gene expression. They demonstrated that acidity was what determined the growth apart from the auxins, so elongation is caused by an acidic environment in the apopolast and it was measured with an extensometer. 13 The cause must be something contained in the cell wall already, so they thought it could be enzymes and they tried to make the proteins non-functional with heat or proteases and then restore them and in fact the growth was restored as well. They determined that the proteins doing this were the expansins and it worked like this: auxins stimulate the activity of plasma membrane H+-ATPases so this acidifies the cell wall and activates cell wall enzymes involved in the destabilization of bonds between and within cell wall polymers → they slide making the cell wall loose. The role of expansins is that of catalysing the weakening of hydrogen bonds between cellulose microfibrils and hemicelluloses (=cross-linking glycans). But there are also XET, xyloglucan endotransglycosylase that cuts and joins hemicelluloses in new configurations so it cuts and then creates a new configuration of the molecules so that for example they can go further from each other, elongating the cell wall. The slow response on the other hand is for sure caused by gene expression and in particular is due to the induction of the synthesis of H+-ATPases that then are recruited by the membrane. But how is auxin really involved in phototropism? We know it’s an oriented growth that is made possible by auxins but we also know that they are mainly produced in the shoot apex and that it seems to go from the irradiated side to the shaded so it moves laterally and the two sides grow differently due to this movement. In fact they measured exactly the growth rate and in the irradiated side is a lot lower than the shaded because of the different distribution of auxins. They also made an other experiment in which gelatine blocks are used to make the tips of the coleoptile grow and then this blocks were put on the top a cut coleoptile only on one side to see what happened. Essentially they saw that even without a light there was a curvature on the opposite side of where the block was. The curvature, as we can see, is proportional to the concentration of auxins. 14 To demonstrate the later transport they did another experiment: 1. If the coleoptile was put in the dark, there still was a curvature (of angle 25.8) so they thought that in the light auxins were destroyed. 2. But they tried the same thing with a unilateral light and the angle is the same, so this means that auxins are not destroyed by light, in fact they also found them analysing the coleoptile. 3. They used a mica sheet that it’s completely inserted both in the coleoptile and in the agar block, so they are independent. After heating, the angles are exactly the same. 4. So they tried with the mica sheet partially inserted in the coleoptile, so that there could be communication and in fact the side that is illuminated has a curvature angle that is about half the one on the dark side, so there’s a lateral transport of auxins, and this is due to the PIN proteins. The PIN1 is involved because it gets destabilized, but also PIN3 because it changes location, it’s redistributed to the side. Another process in which auxins are involved is gravitropism: the growth of a plant following gravity, and it can be positive, like in the case of roots because they grow in that direction, or negative, if they grow in the opposite direction, like it happens in stems. Even when we move a plant the growth is reoriented in order to follow gravity, and the same thing happens in the seeds. The involvement of auxin was tested in the usual way, with the curvature test and in this case the angle of orientation was directly dependent on the concentration of auxins. They used an horizontal coleoptile tip with two agar blocks inserted and then they put them in two different coleoptiles missing the apex to see where there was more auxin and indeed they found that in the lower half, auxin was a lot more and as we can see the curvature is a lot, on the other hand when they use the upper half, it doesn’t really curve that much, and if we go look at the numbers the lower half has the double amount of auxins because it moves towards the gravity force. Also in this case the lower side grows faster in the stem, so there’s an upward bending, and this is the phenomenon of negative gravitropism. In the stem gravity is perceived in the endodermoid layer, essentialy is the innermost layer of the cortical cilinder so it’s localized between the cortical cilinder and the central cilinder, and it’s reach in amyloplasts so contains a high number of starch granules that is why is alsi called the starch sheath. The granules work like statoliths, but we don’t know the exact process. They were found in mutants that showed gravitropism only in shoots so this means that they perceived it differently. 15 In roots in fact they were discovered first and they work in a different way: roots have at their extremity the so-called root cap, a structure that is both protective of the root apical meristem but also has the same starch sheath. Specifically it was found because when removing the cap plants didn’t have a gravitropic response. Inside the cap there’s this structure, the columella that is the one rich in amyloplasts and again works as a statolithe, essentially the fact that they’re heavy get them to sediment at the basal level of the cell and they produce a pressure on the ER and this makes the roots grow towards the gravity. If the plant is differently oriented the pressure on the ER changes and it won’t be uniform anymore so this triggers the different response that is an unknown signalling cascade. Probably this cascade involves an increase in pH of the columella cells, but also changes in calcium concentration that enters the cell immediately after and this was seen in the cells that are closer to the columella, so that are in the lower part of the plant. What happens in the roots is that there’s not the normal transport of auxins that we described, but there’s this fountain model, or auxin reflux loop, especially in the cap, because when auxins arrive from the acropetal transport in the columella there’s a lateral distribution of the hormone thanks to a lateral distribution of the PIN proteins, especially PIN2, so the auxin goes back up uniformely towards the root elongation zone. If we orient a root horizontally we can see that in the lower side auxin is accumulated because of PIN3 that is distributed laterally not uniformely. So actually in the roots a higher concentration of auxins inhibits the growth of the cells, causing gravitropism, there’s a downward bending. To determine this model we used DR5, a synthetic promoter of auxins. Another important aspect in which auxins are involved is apical dominance, so the apical bud of a plant can block the growth of lateral buds, and indeed if we eliminate it, all lateral buds develop, but if we put auxin on top, the inhibition is activated again. In this regulation there are actually two hormones working, auxins that inhibit the growth and cytokinines that promote it; probably there are also other hormones involved like strigolactones that regulate the structure of the buds. Actually IAA also negatively regulates genes that encode proteins involved in the synthesis of cytokinins, so we can conclude that IAA almost completely regulates this activity. Auxins are also involved in the formation of lateral appendixes, so it regulates the phyllotaxy, so the pattern of leaf emergence from the shoot apex, and they do when the concentration of auxin is high. 16 Another process is the formation of lateral roots and this happens in a region just above the elongation zone, the pericycle, that is in the xylem lobes, in which cells can be activated by auxin to elongate. Then it’s involved in the formation of adventitious roots so it stimulates cell division in tissues that are not the roots. In induces the differentiation of vascular tissues, for example when we cut the apex and then we also wound the plant so to interrupt the vascular flow, when we apply auxin cells tend to reconstitute the system because they are stimulated to grow by auxin. Another function is fruit development, that means it’s formation, growth, but not it’s ripening and it was proved that auxins are produced by the seeds, in fact if a fruit has no seeds cannot grow, but if we spray it with auxins it starts to grow again. Now, due to commercial use, we try to produce parthenocarpic fruits, so without the seeds and they need a strategy to uncouple the development of the seeds from the production of auxins → we could apply exogenous IAA, or we could make the plant produce auxins without needing the seeds and the fecondation process, essentialy we engineer plants genetically. Finally it regulates morphogenesis of plants in vitro together with cytokinins. If we increase their concentration we obtain a so-called callus, essentially a cluster of cells that grow indefinitly is put in the right conditions with the right amount of hormones. But if we move the calus to a medium that contains only one of the hormones: If we increase cytokinins and decrease auxins plants tend to produce the organ that produces the hormone that is less present so we obtain the shoots. If we do the opposite we obtain the roots. It all depends on the auxin/cytokinin ratio. Now let’s analyse the auxin signalling pathway: auxin-induced genes contain sequences called AuxRE (Auxin Response Elements) in their promoter, and it’s a very conserved sequence. Proteins called ARFs (Auxin Response Factors) are covalently bound to these sequences and they are transcriptional activators. They are inhibited by the binding with AUX/IAA proteins, which are negative regulators of transcription. But when auxin arrives it binds to a receptor TIR1, a component of a ubiquitin E3 ligase complex, called SCFTIR1 and it’s in the nucleus so it’s a soluble protein. This complex ubiquitinates AUX/IAA, that it’s its target protein, for degradation. 17 Another receptor is AFB (Auxin signaling F-box), they are of 5 types and all have this f-box motif that is a protein-protein interaction motif and targets the complexes. So the activation of transcription is summarized in this picture: when auxin arrives binds to TIR1, SCF is activated, ubiquitinates AUX/IAA that is degraded so ARF is not blocked anymore and transcription is activated. ARF works in dimers to enhance gene expression. Auxin-induced genes fall into two classes: 1. Primary response genes or early genes, that induce in less than 2 hours and are for example AUX/IAA that has a primary response and GH3 that is involved in irreversible degradation and this shows us that there is autoregulation of this processes. 2. Secondary response genes or late genes, induce in 2-4 hours and are correlated to stress situations and this is a reason why it takes more time, for example it happens with ACC synthase, that is for the synthesis of ethylene (cross-talk between phytohormones). Their expression can be blocked by inhibitors of protein synthesis because it requires protein synthesis; specifically the early genes transcribe for transcription factors that are needed to express late genes, and this is another reason why they take more time. This tells us that there’s a tight regulation of the signalling pathway and it’s an autoregulation. There was actually a recent discovery of an additional pathway that is still under study and involves ABP1, auxin binding protein 1 that is a receptor located on the plasma membrane that is recycled by the ER and it’s hypothesised that when auxin binds, the proton-ATPase is activated through a phosphorylation because a kinase is released so the cell wall is acidified. 18 GIBBERELLINS They were studied because they found a disease in rice plants, Bakanae, caused by the fungus Gibberella fujikuroi, and when it infects the plant, it grows a lot and can’t sustain itself. They analysed the fungus and found that it secretes molecules inside the plant that they called Gibberellin A, that actually were a mix of 3 molecules, GA1, GA2 and GA3. They hypotesised that plants can produce this substance as well and this can stimulate a very strong stem elongation, even a dwarf mutant if added with GA grows as tall as the WT. And also the cabbage that needs long days to grow, can grow the same in short days if added with GA. They discovered 140 different gibberellins that are numbered in the order of discovery and from a chemical point of view they are diterpenoids, so have 4 isoprenoids units that consist of 5 carbons, so there are 4 carbon rings that form a tetracyclic skeleton. The base of all the molecules is the ent-giberrelane and then all are modified depending on the type. Usually they are made of 20C, but can lose 1 during the biosynthetic pathway because the position 4 and 10 can form a lacton. Modifications can affect the biological activity of the molecules: OH, hydroxylation → in position 2 it determines total inactivation and this is how a plant inactivates GA, in position 3 determines the most active GA, while in 13 it depends on the plant. The double bond between C1 and C2 gives more biological activity and can stop the OH in C2 so the molecule can’t be inactivated anymore. The biosynthesis of GA belongs to the complex biosynthetic pathways of terpenes, that are secondary metabolites. The pathway starts with IPP (isopentenyl pyrophosphate), that is a 5C molecule, this reacts with DMAPP (dimethyl allyl pyrophosphate), that is a isomer of IPP, so again 5C. when they interact they generate GPP (geranyl pyrophosphate) that has 10C, and then many molecules of IPP are continuously added to generate first FPP (farnesyl pyrophosphate) and then GGPP (geranylgeranyl pyrophosphate), that is the base molecule of 20C from which all of the GA are synthetized. Another pathway starts from this molecule and it’s for the production of carotenoids. It is important to study the biosynthetic pathways of plants in order to accurately plan the biotechnological interventions, for example they engineered plants to produce more carotenoids and they were dwarfs because they used the pathway to produce carotenoids and not GA, so they couldn’t grow. The biosynthesis involves three intracellular compartments: stage 1 in the plastids, stage 2 in the endoplasmic reticulum and stage 3 in the cytosol. In the plastids and in the ER there are always the same functions, but then the process in the cytosol can change, there can be two different parallel pathways that depend on the plant, on the cell type and on the need of the moment. 19 The pathway is specifically: 1. Plastids: IPP is transfomed in GGPP, that is a 20C linear molecule, so they needed two enzymes to create ent-kaurene, that is a cyclic molecule composed of 4 rings and it’s the precursor of all GA. 2. ER: ent-kaurene leaves the plastids and the first molecule is synthetized → GA12, made of 20C and with a carboxylic group. At this point there can be or not the hydroxylation of C13, so two different pathways are possible. 3. Cytosol: the non-hydroxylation pathway uses GA20 oxydase that does many oxydative reactions that produces as a final GA one with 19C, that has a carrier biological activity; then it can be hydroxylazed in C3 by GA3 oxydase, determining the most active GA. The hydroxylation pathway has the same intermediates, and uses the same enzymes. At this point both can be inactivated by GA2 oxydase, that is a catabolic step. The pathway was discovered with many mutants, for every enzyme we have one, and for example for the first enzyme is a complete dwarf, while for the enzymes that produce ent-kaurene they are not completely dwarfs because they are regulated by a small family of genes that shows redundancy. But there’s also a slender mutant, so very tall because there’s no inactivation of GA so it grows a lot. The regulation of this process is done by the control of the pathway, the transport and the conjugation, that can be reversible with sugars, or permanent with OH; it can also be due to environmental factors and feedback inhibition. Gibberellins regulate their own metabolism: a high concentration of active GAs inhibits the expression of GA20ox e GA3ox genes→ thus repressing the GA synthesis: negative feedback regulation; a high concentration of active GAs stimulates the expression of GA2ox gene → thus causing the hormone inactivation: positive feedforward regulation. The synthesis is also regulated by the photoperiod, for example spinach usually grow with long days, but can grow with short days even if they show a rosette form, but if we add them exogenous GA, they grow like the WT. GA are produced in the shoot apex, in developing seeds because embryos produce them, in the anthers because they are involved in pollen formation and development. They were determined with the use of the transgenic reporter GUS, that is a bacterial gene that produces a blue color if added with the right substrate, so is useful for this kind of things. Knowing this pathway can be useful so we modulate the plant height, because for example a mutant for overexpressed GA20ox generates more active GA so higher plants, and it could be useful in sugarcane so we can obtain more sugar from longer stems. The height can be genetically engineered, depending on what we want to obtain, like in wheat, too high plants are difficult to harvest, so we need to modulate the right height. Of course mutant plants deficient in the production of gibberellins can exhibit additional phenotypic defects, like an altered development of seeds. 20 GA are involved in the germination of seeds, and in the mobilization of starch reserves, in fact we can see that seeds have an embryo, one or two cotyledons, but most of it is the endosperm, that is the reserve of starch and site of communication between endosperm and embryo. In the cotyledon there’s the scutellum, that mediates the exchange of substances between the embryo and the endosperm because the embryo produces GA that are transported to the endosperm through the scutellum. In particular the target site of GA is the aleurone layer, that is at the periphery of the endosperm and it contains a large quantity of protein storage vacuoles, so of a lot of proteins, that are hydroxylases. These are liberated from a signal that comes from transcription factors activated by GA and go into the endosperm to release starch granules and degrade starch so to produce glucose, needed by the seed. The degradation of starch happens because it’s formed by polymers of amylose and amylopectin, the first is composed of monomers of glucose linked by α(1-4) bonds, it’s a linear molecule, the second has also branches given by α(1-6) bonds, so the need to undergo hydrolytic degradation. There are two enzymes involved, α-amylase, that breaks α(1-4) linkages randomly in the molecule, and β-amylase that breaks them only at the extremities, creating as a result β-maltose. Of course there’s also the need to create glucose from maltose, and this is done by the α-glucosidase, and then they also need to eliminate the branches, so there’s the debranching enzyme. There’re actually a lot of other enzymes, but these are the main ones. The only enzyme that is present inside the endosperm already is the β-amylase, because it has a pre-peptide that gives inactivation, so it needs to be cleaved off to activate it. The others are brought there, as well as the protease to activate the β-amylase. GAs induce the expression of several hydrolytic enzymes, both hydrolases and proteases, used for the degradation of proteins in the vacuoles, so indirectly they are used for the degradation of starch. In fact we can see that the synthesis of α-amylase after treatment with GA increases after some hours. Gas are involved in stem growth, both affecting cell division and cell elongation, in response to treatment they increase the number of cells and their size, so a plant grows a lot. For example there’s this deepwater rice plant that undergoes periodical floodings so when this happens there’s a sudden elongation of the plant in order not to be submerged because there’s stimulation of the synthesis of GA. This happens especially in the internodes, between nodes, so where the leaves grow, and that’s because they have the intercalary meristem, a group of interspersed cells that can divide if stimulated by GA. In fact GA induces the expression of CDKs, cycline- dependent protein kinases that act in checkpoints of the cell cycle, accelerating it, so as we can see there’s an increase in the number of nuclei in S phase and in G1. 21 They don’t really know how it works for cell elongation, but they’re sure they work together, with different actions, in fact if plants are treated with exogenous GAs, there’s induction of the growth after a lag phase of at least 40 minutes, so way longer than auxins, and if they are added, there’s an additive effect so they think the mechanism of growth for the two hormones are different. In particular Gas induce the synthesis of expansins, and auxins contribute, because the height of the plant is proportional to the concentration of expansins, there’s cross-talk between hormones but we don’t exactly know how. We know that the synthesis of GAs can be induced by auxins; this was demonstrated by the decapitation of a plant, that is the main source of auxin, and in fact GAs are not produced anymore, but if auxins are applied exogenously GAs increase. This regulation happens in two ways: by inducing the expression of GA3ox and by inhibiting the expression of GA2ox, so by activating a lot of GA and stopping its inactivation. GAs are also involved in the transition from the juvenile plant, that cannot reproduce, to the adult that is sexually mature. This was seen in conifers’ seedlings that if treated with GA, there was the induction of buds for reproduction, and in ivy they also saw the conversion of shoot apical meristem in the juvenile form, so there are leaves instead of fruits. They also stimulate the growth of parthenocarpic fruits and they accelerate the production of malt, so they accelerate the degradation of starch granules. The signaling pathway, that leads to the response of the plants. The first receptor that was discovered was GID1, GA insensitive dwarf 1, that is a soluble protein localized in the nucleus, in fact not all receptors are inserted in the membrane. This binds to GAs leading to a conformational change that activates the pathway. There are negative regulators of the GA response, the DELLA (depends on the aa of the sequence) proteins, that as we can see are characterized by two domains, the DELLA domain, in the N-terminal that is a regulatory domain that binds to the receptor complex and can activate the signaling pathway by binding to it. The C-terminal domain is the GRAS domain, that is a repressor domain that binds to the transcription factors and inhibit them, so it’s an indirect repression. Then we also find the nuclear localization sequence that is needed to bring the molecule into the nucleus, and the leucine repeats that are needed for protein-protein interaction, in fact DELLA can interact with many other proteins. There are 5 isoforms in Arabidopsis, only 1 in rice. GAs can cause degradation of DELLA and this is needed in order to have a cellular response. This was demonstrated by growing transgenic plants with DELLA fused with GFP but using still the endogenous promoter and they saw that without GA, DELLA is in the nucleus, when treated with GA instead after two hours we see no more fluorescence. If instead they were treated with drugs (like paclobutrazole) that inhibit the synthesis of GA the signal is even more intense because there’s absolutely no gibberellins present, not even endogenous ones. Finally DELLA is degraded normally by ubiquitination, in fact in all of these passages the only way to stop the process is the hydroxylation of GAs that inactivate them, there’s no feedback or anything. The signaling pathway in the absence of GAs work through the DELLA protein that represses the positive transcription factors so it’s indirectly because it doesn’t bind directly to DNA → repression of transcription. In the presence of (active) GAs instead they bind to the receptor in the nucleus and this changes its conformation so it can interact with DELLA through the Della domain. DELLA undergoes a conformational change as well and becomes able to associate with GID2 (GA insensitive dwarf 2), an F-box protein that is part of SCF, so a ubiquitin E3 ligase, and this 22 means that DELLA is ubiquitinated and degraded through the proteasome so it cannot affect the positive transcription factors anymore → activation of transcription. GID1 appears like a box with N-terminal extension that can close like a lid on top of it when GAs bind and this is also the domain that interacts with DELLA and then both the della and the gras domain undergo conformational changes so that SCF can bind to gras and the polyubiquitination happens. All of this was of course discovered thanks to mutants, that are involved in the signaling not in the biosynthetic pathway and they actually obtained strange results from them, and later they found out that it depended on where the mutations happened. In fact the mutation in the gras domain is slender, so very tall, instead in the della domain it’s dwarf. This happens because when there’s a mutation in the gras domain, the repressor domain doesn’t work, so essentially DELLA cannot work as a repressor and this means that the repression is always off so there are a lot of GAs because they are not blocked and the plant grows so much, but even in their absence the plant can still grow. On the other hand if the mutation is in the della domain, the regulatory domain doesn’t work so DELLA is not degraded and the repression is always on, the signaling pathway doesn’t even start even if there are GAs, and this brings the plant to the impossibility to grow. We talked about a transcription factor, this is the GARE, gibberellin response element, that is part of the sequence of the promoter of GA response genes, it’s a very conserved sequence and it can bind to different transcription factors. An example is its role in the α-amylase pathway: this gene needs the transcription of GAMYB, so a MYB of GAs that are general transcription factors and this is an early gene, so then it can stimulate the expression of late genes, like the α- amylase, and they also measured when these genes were expressed, and demonstrated that are expressed at different times, meaning that late genes need the expression of early genes. 23 Another aspect that was analyzed was the events induced in the aleurone layer during seed germination: 1. Calcium transient is activated first. 2. Then CaM are induced, telling us that calcium is very important. 3. cGMP, but it’s not clear how this is involved. 4. GAMYB, that is then necessary for the α-amylase. 5. α-amylase. They also hypothesized that GID1 is not the only receptor, probably there’s one in the plasma membrane, so they did experiments in protoplasts with agar beads that stopped GA from enter and they noticed that the signaling pathway was starting anyways, so probably there’s another receptor that has not been identified yet, but they hypothesized a possible pathway: this receptor activates a heterotrimeric G-protein and this activates two pathways, one is calcium independent, so it basically just uses GID2 and then links to SCF, but there’s also a calcium dependent one that was found using inhibitors of calcium and the α-amylase was still produced but it wasn’t secreted, so this pathway is probably needed for secretion, while the other for the synthesis. This pathway is still under investigation. 24 CYTOKININS These hormones were actually discovered by actually looking for them, in particular they were looking for a molecule that was able to stimulate cell division in plant cells, for example like after a wound when cell proliferation is reactivated. They were discovered with Agrobacterium tumefaciens, that generates galls, tumor-like structures used by the bacterium to survive, that induce the cells to produce substances to make cell division happen. Mature adult plants can actually be induced to differentiate so basically they are induced to proliferate and in order to find out which were this molecules, they used coconut milk, that is basically liquid endosperm and they hypothesized that it could be the DNA, because also using adenine it works. So they tried to use a substance with a lot of DNA, so they used sperm from a fish that was autoclaved and they obtained a very good result. The molecule that they isolated was kinetin, that is a DNA degradation product and it’s specifically derived from adenine, so it’s highly probable that plants can produce it too. They did the experiment in a natural environment using maize and they found the first cytokinin, that has basically the same biological activity of kinetin but even more powerful and it’s zeatine. This is the most common cytokinin and also the most present and it’s common to every plant and derives from adenine, essentialy by adding a side chain, an isopentenylic group with 5C and linked to the nitrogen of C6. This group has a double bond so there can be two configurations that are isomers in equilibrium between each other, CIS and TRANS, this is the most common and more biologically active. There are also different forms with different isopentenylic groups but all derive from adenine, that is a 6-amino purine. Some were also artifically synthetized and have no isopentenylic group but still have biological activity. They can be present as ribosides (+ sugars), or ribotides (+ sugar + phosphates) and these forms are not active so they are used by the plants to inactivate cytokinins. Or they can be present in tRNA molecules, close to the anticodon sequence and like this they don’t have any biological activity, the RNA needs to be hydrolyzed. Actually most of them are not produced this way, just a small quantity. They can be produced also by pathogens, like bacteria and fungi that infect plants and produce cytokinins and they affect the plant growth and this brings to the proliferation of the shoot apical meristem and uncontrolled growth of lateral buds, so bundle of branches laterally in the plant, in a phenomenon called fasciation, in which there’s also the loss of the apical dominance. Or they can also infect the plant and make it produce cytokinins, like A. tumefaciens does so that the plant grows the crown galls, so they are in the crown, the base of the stem. The biosynthetic pathway needs a source of isopentenylic group (DMAPP, dimethylallyl pyrophosphate) and of adenine (ATP or ADP), they generate the first molecule of transzeatine that can be contained in a nucleoside or nucleotide and this is made possible by the IPT enzyme, isopentenyl transferase, that transfers the group to adenine and then with an oxidation we obtain transzeatine. Through an isomerase we obtain ciszeatine and with an oxidative cleavage of the lateral chain we get the irreversible inactivation of the hormone. They can be inactivated irreversibly with an oxidative cleavage that degrades them through the cytokinin oxidase; also N- glucosylation of the adenine ring in specific positions (N3, N7 and N9) inactivates them irreversibly. On the other hand O- glucosylation of the side chain causes a reversible inactivation used to store the hormone. 25 The sites of production of CKs are mainly the root apex, so the apical meristem, but a little is produced also in the shoot apex, in lateral buds and in developing leaves; they are translocated through the xylem and phloem, for example from the roots they go through the xylem. They can act both at long and short distance, so with cells nearby but also the same cells in which they are produced, for example they act on the measure of the shoots. They can also be produced in the nodes where they stimulate the growth of the axillary buds. CKs regulate cell division in the stem and in the root, specifically they regulate the activity of the apical meristem, but they have an opposite effect: they promote the growth of the stem but they inhibit that of the roots. As we can see if we overexpress cytokinin oxidase CKs are degraded and the stem cannot grow much and this is due to the effect on the stimulation of cell proliferation in the meristem so where cells divide. In particular the shortage of CKs determines the reduction in size of the SAM and this reduces the stem growth. On the other hand when roots overexpress CK oxidase they grow much more, in fact in this case there are less CKs and roots can grow more, there’s less inhibition. This effect is due to the promotion of the exit of cells from the meristem, so they regulate the number of divisions that cells undergo before leaving the meristem. If there’re high CKs there’s a smaller RAM, so less meristematic cells and the roots grow less. If they’re low instead the RAM gets bigger, there are more meristematic cells and the root grows more, because if they’re low, there’s no exit of cells and this means that the RAM can grow more. A triple signaling mutant was produced, so that doesn’t perceive CKs, it’s smaller both in the shoots and in the roots, but why? It’s a triple mutant because there are 3 receptors and even if there’s a normal production of CKs, they are not perceived, the signaling pathway doesn’t start. This is a gaussian curve of response to cytokinins for the growth of the root and of the stem, in this case the WT has the optimal concentration of CKs, so the triple mutant and the overexpressor aren’t optimal, so there’s no growth. In the case of the root when, the WT has a supraoptimal cytokinin level so there’s inhibition, but in the overexpressor there’s an optimal level, in fact they decrease and plants grow more, but in the triple mutant there are no receptors so even if the CKs are present, again there’s no signaling pathway. Cytokinins regulate specific components of the cell cycle, CDKs, cyclin-dependent kinases, and cyclins that work together and in checkpoints of the cell cycle; specifically the CDKs are regulated by phosphorylation that deactivates them and dephosphorylation that activates them: in the G1→S transition they induce the expression of a D-type cyclin (CYCD3) and in the G2→M transition they activate a phosphatase that removes the phosphate group inhibiting the main CDK the CDC2, so it’s activated and the cell can enter mitosis. Actually the cell cycle is under the control of many hormones, in particular CKs and IAA, because CDC2 is expressed under the control of CDC2. We actually always need the right concentrations of these two hormones because otherwise we’d have just one of the two proteins and the cell cycle cannot work perfectly. 26 This ratio regulates plant morphogenesis in vitro also, so for example if we cut tobacco leaf disks they generate a callus and we know that from this we can obtain an entire plant that is a clone of the initial. In vivo this can be observed in the effect of mutations in the tDNA that Agrobacterium tumefaciens inserts in the nuclear genome of the plants it infects. In fact the tDNA of these bacteria have the genes for both IAA, we already saw that there’s this pathway, and CKs biosynthesis, with the IPT gene. These hormones make the plant proliferate. If there are low ratios, meaning that one of the two hormones is missing, or mutated the tumors are slightly different, it can also happen because we change gene expression artificially: A. WT, exact ratio. B. Rooty tumor, high IAA/CK ratio so roots are produced in the tumor. C. Shooty tumor, low IAA/CK ratio so shoots are produced in the tumor. CKs promote the growth of axillary buds, so basically they modify the apical dominance that is regulated by IAA, in fact the apical bud produces IAA that inhibits lateral buds growth. There’s actually an interplay in shoot branching regulation, because auxins inhibit the expression of IPT, that is the main enzyme that produces CKs, but it also stimulates the production of CK oxidase, that is instead the enzyme that degrades CK → this maintains CKs in the axillary buds at a low level and they don’t grow. If we decapitate the shoot apex, the axillary buds grow until they become apical buds, so a source of auxin and they inhibit the others, it’s basically a cycle. Another function is the delay of leaf senescence, it doesn’t totally stop it, but this topic is still under investigation. Senescence is the programmed aging that brings to the death of some parts of the plant, and it’s controlled by senescence associated genes. It has been demonstrated though, in transgenic plants expressing the IPT gene fused to the promoter of a SAG, that works basically like a lytic enzyme, the plants are more green because CKs are usually produced in young leaves. Of course this has potential biotechnological applications, because leaves can undergo senescence after a longer period of time. They promote the movement of nutrients, in particular their acquisition, in fact it was demonstrated that nutrients are transported towards tissues treated with cytokinins thanks to radioactivity. The leaves treated became sinks instead of sources for nutrients, and this is how senescence is delayed probably. They promote the development of chloroplasts, in fact in seedlings grown in the dark etioplasts grow, but if treated with cytokinins chloroplasts start to be created, prolamellar bodies become prothylakoids. Finally they are involved in the formation of root nodules during nitrogen-fixing symbiosis that can happen between legumes and rhizobia, so they activate cell division in the root cortex and these nodules are important for nitrogen fixation, that is fundamental for plants. The signaling pathway is based on a mechanism that is very common in bacteria that from stimuli induce gene expression and it’s the two-component system, that as the name says, it has 2 components, so two proteins. 27 The first is the sensor histidine kinase, that is inserted in the membrane, it works in dimers and it binds the ligands; it’s as well composed of an input domain outside the membrane that specifically links to the ligands, and a transmitter domain that is internal and when the ligands bind it autophosphorylates in a conserved histidine residue. The second component is the response regulator, as well composed of two parts, the receiver, that basically receives the phosphate from the transmitter and it’s phosphorylated in a conserved aspartate residue; then there’s the output domain that instead generates the response, so it’s a DNA binding domain that when activated by this phosphorylation can work as a transcription factor and activate gene expression. In plants the situation is a little more complicated because there’s a multiple-step phosphorelay system, that even if it’s similar to that of bacteria is composed of many components and there are more transfers of phosphates. The first is again a sensor histidine kinase, that binds to CKs and works in dimers, but it actually has 3 domains, the input, the transmitter, that still is able to autophosphorylate a histidine, but also a receiver, that with an aspartate it receives the phosphate from itself but another domain and this is why the sensor is actually called hybrid, because there’s already a receiver domain. This is present bot in the plasma membrane and in the ER, and lately they actually think that is more present on the ER membrane than on the plasmatic. The second component is the AHP. The last one is the response regulator, that in the case of CKs is called ARR and it’s located in the nucleus because it needs to activate transcription so it needs to be close to the DNA. The receptors that were identified are CRE1 (cytokinin response 1), AHK2 and AHK3 (Arabidopsis histidine kinase), that probably are activated by different stimuli and activate different pathways, but have the same exact function and structure: an extracytosolic domain that in dimers binds CKs, an intracytosolic domain with histidine kinase activity that autophosphorylates its own H, and an intracytosolic receiver domain that receives the phosphate into an aspartate. Of course all of this was discovered in Arabidopsis mutants with alterations in the receptors; given that there are 3, they created both double and triple mutants, the double mutants showed some differences between each other suggesting that the 3 receptors could be more or less important, but the plants still grew because at least one was present so it overcame the lacking of the other 2. The triple mutant on the other hand is completely unable to grow both in shoots and roots because it can’t perceive the signal. The response regulators, that are in the nucleus are the ARR (Arabidopsis response regulator) and they can be of type-A, that have only the receiver domain, and are early genes, so they are rapidly expressed in the presence of CKs, so they are induced by the signaling pathway and they enter the nucleus where they work as negative regulators. Type-B regulators instead have also an output domain that is basically a transcription factor, so it activates gene transcription 28 because it binds to DNA. It’s a constitutively expressed protein so it’s already in the nucleus and it has a positive effect, in fact we can see that CKs do not induce their expression, but does with type-A. The last component that we didn’t previously considered is AHP (Arabidopsis histidine phosphotranferase) that is a shuttle protein that works between the nucleus and the cytosol and basically catalyzes the transfer of the phosphate group from the receptors to the response regulators. It has a conserved H as well, where the phosphate is transported and as soon as it does its action, it goes back into the cytosol. We can summarize the pathway like this: Citokinin binds to its receptor on the ER/PM (extracytosolic domain); in the intracytosolic kinase domain there is the autophosphorylation of a histidine residue, then the phosphate group is transferred on an aspartate residue of the receiver domain and to a cytosolic AHP (on a H residue). The phosphorylated AHP moves into the nucleus and transfers its phosphate group to a response regulator called type-B ARR (on a D residue) because it’s already there, this binds to DNA through the output domain and induces the expression of cytokinin response genes, so the early genes. Among them there are the Type-A ARR genes and even type-A ARRs are phosphorylated by AHP, at this point they become able to interact with various effectors → they negatively regulate the response to CKs. 29 ETHYLENE It’s a single gaseous hormone, so it’s the first time we find something like this, it’s also a very simple molecule, C2H4, and was discovered when studying germination of seeds in the dark. Usually they obtained tall and long etiolated seedlings, but these were short, thick, they had a tendency to grow horizontally, but when they opened a window to change the air, the phenotype was restored, so probably there was something in there, maybe due to the illumination, so they analyzed the air and found out that it was ethylene, but they didn’t think it could be naturally produced by plants. They stabilized that ethylene gave the so-called triple response to plants treated: 1. It reduces the elongation of the stem. 2. It increases the lateral expansion of the stem (swelling). 3. It promotes a horizontal growth, so the seedling doesn’t grow upright, but bends. Later on ethylene was purified in ripening apples, so it is indeed produced by plants, but it also has a biological activity, specifically it’s active at very low concentrations. It’s a gas so it’s not very easy to work with, now they actually use ethephon, that is soluble and can be easily sprayed, so it’s absorbed by the cells, it dissociates and release ethylene. The biosynthesis of ethylene starts from methionine, that is the precursor, through AdoMet synthase it binds to adenosine and with the help of ATP it loses the phosphates and creates S-Adenosylmethionine. This molecule, through the ACC synthase enzyme is transformed indeed in ACC (1- Aminocyclopropane-1-carboxylic acid), the direct precursor, then transformed in ethylene by ACC oxidase, that needs oxygen to work. ACC synthase can be affected by many factors, like fruit ripening, flower senescence, IAA, wounding, chilling injury, drought, flooding, that promote all the synthesis. Methionine can be recycled through the Yang cycle. ACC can be irreversibly inactivated by the conjugation with Malonyl-CoA. Ethylene is synthetized in all organs because it can’t move so wherever it’s needed it must be produced there. It’s especially needed when there’s senescence, abscission of leaves and flowers, and the ripening of many fruits, it’s induced by many stresses, of both abiotic and biotic nature. In these cases genes encoding the key enzymes are induced or the activity of those enzymes. Ethylene promotes the ripening of many fruits, but not the developme