HF Class One Slides PDF
Document Details
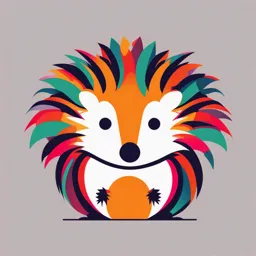
Uploaded by IlluminatingFourier
Tags
Summary
These slides cover human factors in aviation, including airmanship, professionalism, responsibility, and various models like SHELL. It also touches the physiology of flight and how the effects can impact human performance.
Full Transcript
Airmanship, Professionalism and Responsibility Airmanship – Is all about making good decisions both on the ground and in the air, which requires a high degree of: - Situational awareness - Knowledge - Skills - Discipline Class exercise: List as many features of good airma...
Airmanship, Professionalism and Responsibility Airmanship – Is all about making good decisions both on the ground and in the air, which requires a high degree of: - Situational awareness - Knowledge - Skills - Discipline Class exercise: List as many features of good airmanship as you can think of on the whiteboard. Professionalism: ‘Professionalism in aviation is the pursuit of excellence through discipline, ethical behavior and continuous improvement.’ – National Business Aviation Association. - Piloting for personal reasons would indicate a Private Pilot Licence, and as per CAA CAR Part 61.155: (b) The holder of a private pilot licence must not act as pilot-in-command or as co-pilot of an aircraft (1) For remuneration; or (2) If the aircraft is being operated for hire or reward Commercial pilot licence privileges: CAA CAR Part 61.205 (2) Act as pilot-in-command of an aircraft that is certificated for single pilot operation and engaged on an operation for hire or reward: (3) Act as pilot-in-command of an aircraft that is certificated for multi-pilot operation and engaged on an operation for hire or reward, that is not an air transport operation; (4) Act as co-pilot of an aircraft that is certificated for multi-pilot operation and engaged on an operation for hire or reward. Airline transport pilot licence privileges: CAA CAR Part 61.255 (1) Any of the privileges of a private and commercial pilot licence: (2) Act as pilot-in-command of an aircraft that is required to be operated with a co-pilot and is engaged on an air transport operation or an operation for hire or reward. SAFETY: “The condition of being protected from, or unlikely to cause, danger, risk, or injury” EFFECTIVENESS: “The degree to which something is successful in producing a desired result” EFFICIENT: “Achieving maximum productivity with minimum wasted effort or expense” - Oxford Dictionary The safety and security of the aircraft and its crew and passengers. Operate the aircraft efficiently and economically with good time management skills Adhere to the aircraft flight manual and company standard operating procedures. The pilot-in-command is responsible for not only the safe operation of the aircraft, but also for a number of people, including: - Co-pilot(s) - Cabin crew - Other crew such as loadmasters, etc. - Passengers The co-pilot will assist the pilot-in-command in being responsible for the safe operation of the aircraft, cabin crew and passengers. If the pilot-in-command become incapacitated, the co-pilot will assume the role as the pilot-in- command: i.e. the co-pilot is second in command. Human factors models and programmes Human factors is the study of the interrelationship between humans, the tools and equipment they use in the workplace, and the environment in which they work - Social and personal skills - Used to reduce the likelihood of errors The SHELL model The SHELL model refers to the interaction between you (the liveware) and various elements that make up the aeronautical system. According to this model, a weakness in the interaction of two or more of these elements is generally the cause of aviation accidents, incidents, and errors. SOFTWARE: Company policies, rules, regulations, documents, checklists, manuals, placards, maps, charts, and other components that make up an organisation’s standard operating procedures (SOPs). HARDWARE: Physical components utilised in the system. These include infrastructure, equipment, computerised systems, displays, controls, tools, seats, and all other items used in the performance of aviation duties. ENVIRONMENT: The setting in which the aviation operations take place. Includes the physical (airspace, terrain, weather, airports), operational (IFR, VFR, airline, charter, military), political, and socio-cultural conditions that impact the overall operation. LIVEWARE (OTHERS): Human components that operate outside the flight deck. Includes air traffic control (ATC), cabin-crew members, dispatchers, mechanics, company management, gate personnel, government authorities, fuellers, baggage handlers, etc. LIVEWARE (YOU): The pilot/flight crew member -Knowledge -Skills and abilities -Training -Attitude -Cultural beliefs -External influences Liveware (both forms) is the most flexible and most important component of the SHELL model. The role of human factors in promoting safer aviation: Human factors identifies that the human interaction with the other elements of the system, is the result of accidents, incidents and errors, rather than the human element on it own. Human factors programmes work to identify the flaws in the interaction of the system elements and to develop methods to mitigate accidents, incidents and errors. Physiology and the Effects of Flight The Atmosphere The gases in the atmosphere: - Nitrogen 78% - Oxygen 21% - Argon 0.93% - Carbon dioxide 0.04% - Trace amounts of neon, helium, methane, krypton and hydrogen, as well as water vapor. Atmospheric gases are held in place by gravity. Variation of pressure as altitude increases Near the Earth’s surface, the gas molecules are more tightly packed meaning that pressure is greatest at sea level than at altitude. At 18,000ft the atmosphere is half what it is at sea level. At 34,000ft the atmosphere is a quarter of what it is at sea level. The three major effects of altitude on the human body caused by pressure changes: - Lower external pressure causes gases inside the body cavities to expand. - Lower oxygen pressure causes hypoxia. - Dissolved gases come out of solution due to decreased external pressure. Partial pressure is the pressure exerted by a given gas in a mixture At sea level the atmospheric pressure is 760 mm Hg. So the partial pressure of oxygen outside the lungs at sea level is 760 x 0.20 (% of oxygen in the atmosphere)= 150 mm Hg. Once breathed in this further reduces to 102mm Hg – the required partial pressure of oxygen in the lungs for normal human functioning. - The partial pressure of oxygen reduces to half at around 9,000 ft. Where as atmospheric pressure reduces to half by 18,000 ft - 10,000ft 55mm Hg - 8,000-10,000ft hypoxia sets in - 33,700ft you’ll need 100% oxygen, any higher and it will need to be under pressure Circulation and respiratory systems Physiology and anatomy of the respiratory system: There are 3 major parts of the respiratory system: - The airway, which includes the nose, mouth, pharynx, larynx, trachea, bronchi, and bronchioles, carries air between the lungs and the body’s exterior. - The lungs act as the functional units of the respiratory system by passing oxygen into the body and carbon dioxide out of the body. - The muscles of respiration, including the diaphragm and intercostal muscles, work together to act as a pump, pushing air into and out of the lungs during breathing. The respiratory system: Physiology and anatomy of the circulatory system: The human cardiovascular system is composed of a heart which pumps blood through a closed system of blood vessels. The heart is composed mostly of cardiac muscle, or myocardium. Its primary function is to transport nutrients, water, gases, wastes, and chemical signals throughout the body Blood: -Is the primary transportation system for materials. Arteries carry oxygen rich blood through the body away from the heart and veins carry carbon dioxide rich blood back to the heart. -Is slightly alkaline, regulates pH levels. -Regulates body temperature. -Protects from fluid loss by clotting at the site of injuries. -Protects from toxins and pathogens, through the action of white blood cells and antibodies. Physiology and anatomy of the circulatory system: The circulatory or cardiovascular system is one of the major body systems. It transports oxygen and nutrients to various parts of the body and takes away carbon dioxide and waste products. It is made up of: - The heart, a muscular pump the size of a closed fist that is divided into a left and right side and has two chambers on each side. It contracts forcing blood through one-way heart valves and on through the arteries. The heart has major vessels that supply it with deoxygenated blood (travels back to the heart from the body), and major vessels that carry oxygenated blood away from the heart to all the parts of the body. - Blood vessels, consisting of arteries, veins and capillaries. Arteries carry oxygenated blood to the cells of the body, veins carry deoxygenated blood away from the cells. Hypoxia Is a deficiency in the amount of oxygen reaching the tissue cells. The four primary types are: Hypoxic (sometimes called hypoxemic) hypoxia: a decrease in the number of oxygen molecules available to the respiratory system. Hypoxic hypoxia results from the decrease in atmospheric pressure associated with gains in altitude. Hypoxemic (or anaemic) hypoxia: With this form of hypoxia, the insufficient oxygen state is not caused by a lack of available oxygen, but rather by the inability of the blood’s haemoglobin to transport adequate oxygen to the body’s cells. Such conditions can have environmental and/or physiological causes. Histotoxic hypoxia: Sufficient oxygen reaches the body’s cells, but the cells are unable to properly utilise the available oxygen. This inability to use oxygen is most commonly caused by alcohol, drugs (narcotics), and/or certain poisons. Stagnant (or ischaemic) hypoxia: Caused by a lack of satisfactory blood flow. Often results from physiological problems, e.g., poor circulation, arterial constriction, shock) or environmental conditions (e.g., high G loads). Causes of Hypoxia: Hypoxic (hypoxemic) hypoxia: caused by a reduced concentration of oxygen particles available for cellular respiration. Commonly the result of increasing altitude, and the corresponding drop in atmospheric pressure. It can arise from failure to use supplemental oxygen at high altitudes, loss of aircraft pressurisation / decompression, and/or extended operations at moderate altitudes without supplemental oxygen/pressurisation. Hypoxemic (or anaemic) hypoxia: The oxygen is sufficient for respiration but the haemoglobin is unable to sufficiently transport it. This is often caused by anaemia, blood loss/donation, or disease. Carbon monoxide adheres to haemoglobin approx. 200 times easier than oxygen which can reduce the amount of oxygen being delivered through the body. Histotoxic hypoxia: This happens when cells cannot properly use the oxygen delivered to them. It is caused by ineffective oxidative phosphorylation enzymes. Histotoxicity can be attributed to alcohol, certain drugs (mainly narcotics), and certain poisons (e.g., cyanide). Stagnant (ischaemic) hypoxia: Poor circulation prevents the blood from delivering sufficient oxygen to the cells. In flight, this can be caused by high G-loads. From a physiological standpoint, shock, constricted arteries, cold temperatures, and heart problems can all contribute to stagnant hypoxia. The symptoms of hypoxic hypoxia are: Apparent personality change. A change in outlook and behaviour with euphoria or aggression and lack of inhibitions. Impaired judgement. Loss of self-criticism and individuals are unaware of their reduced performance. Short-term memory loss exacerbates this condition and can occur at approximately 12 000 ft. Headache (particularly if mildly hypoxic for a long period). Tingling in hands and feet. Increased rate of breathing - hyperventilation. Muscular impairment. Finely co-ordinated movements become difficult through slow decision making and poor fine muscular control. Handwriting becomes more and more illegible. In the late stages of hypoxia, muscular spasms and convulsions may occur. Memory impairment. Short-term memory is lost making drills difficult to complete. This starts at approximately 12 000 ft. Visual sensory loss. Vision is affected early. Colour perception is reduced and peripheral vision is gradually lost. The light-sensitive cells of the eye are particularly oxygen “hungry” and a deterioration of night vision can occur at altitudes as low as 5000 ft. Tunnel vision develops making it necessary to make larger head movements to scan the instruments and the external environment. Impairment of consciousness. As hypoxia progresses the individual’s level of consciousness drops until he/she becomes confused, then semi-conscious, and unconscious. Cyanosis. An individual who has become hypoxic at altitude is likely to be Cyanosed, that is the lips and fingertips under the nails will develop a blue tinge, due to much of the blood haemoglobin being in the deoxygenated state. Formication. The hypoxic individual may experience formication, a creeping sensation on the skin, as of ants crawling over the body. Unconsciousness. Death. Detecting Symptoms of Hypoxia Hypoxia is more difficult to detect than one would imagine. A person’s lack of awareness that there is a problem and rapid loss of consciousness is the biggest danger with hypoxia. This difficulty in recognising hypoxia occurs for several reasons: - Euphoria: A feeling of happiness or contentment brought about by a reduced amount of oxygen reaching the brain. - A false sense of security or well-being: Hypoxic pilots tend to downplay the importance of their physiological state, often assuming everything will be fine. Detecting Symptoms of Hypoxia cont. - Gradual onset: In many cases, the subtle accumulation of hypoxia can make it very difficult to detect, even for pilots who are aware of its symptoms. - Memory, alertness, judgment, and decision-making ability are impaired: Hypoxia limits the time of useful consciousness during which pilots are capable of rational thought. As altitude increases, this period decreases substantially. Hypoxic Effects on Vision and Cognitive Performance Vision: Hypoxia affects the cells of the eye that are responsible for peripheral and night vision, the rods. Reduction in night visual acuity is one of the first symptoms and can occur at as low as 5,000 ft. The eyes gradually lose their peripheral vision, resulting in tunnel vision. Cognitive performance: As oxygen availability drops, cerebral performance diminishes accordingly. In most cases, cognitive ability begins to decline at lower pressure altitudes than many bodily indications appear. Effective mental capability (as measured by time of useful consciousness) ceases before physiological limits (i.e., unconsciousness) are reached. Preventing Hypoxia Hypoxia can be difficult to detect so prevention is the safer method of action. Supplemental oxygen: One of the most effective ways to avoid hypoxia is supplementary oxygen. This is the primary reason aviation authorities require the availability and usage of oxygen for operations above certain elevations. Cabin pressurisation: Cabin pressurisation increases the concentration of O2 available for respiration. Rate of ascent: High rates of ascent tend to negate an individual’s tolerance to a reduced-oxygen environment. If possible, decrease the aircraft’s climb rate to permit the body more time to adjust to the reduced atmospheric pressure. Time at altitude: Hypoxia and its effects increase with time at altitude. Limit the time spent at high altitude and use supplemental oxygen. Alcohol/drug use: Alcohol and certain drugs raises the body’s susceptibility to hypoxia. Whenever possible, avoid usage of such substances. Smoking/carbon monoxide avoidance: Cigarette smoke and faulty aircraft cabin heaters contain carbon monoxide. Avoid cigarette smoke and use an aircraft carbon monoxide detector. Physical conditioning: Exercise allows the body to efficiently utilise oxygen. You can lower the possibility of hypoxia with a regular active lifestyle. Nutrition: A healthy diet can help delay the onset of hypoxia and reduce its physiological effects. Acclimation: Those who live at higher elevations tend to have a reduced risk of susceptibility to hypoxia. Environmental factors: Being too hot or too cold in a cabin can increase the body’s oxygen usage. If available, use aircraft environmental systems to maintain a moderate cabin environment. Also, dressing appropriately for the flight conditions can help reduce the body’s need for energy (and therefore O2). Use of automation: Hand flying and manual navigation/calculations require more mental and physical effort, and therefore, more oxygen. If available, flying with autopilot, GPS, etc., can reduce one’s O2 requirements and susceptibility to hypoxia. Treating Hypoxia To recover from hypoxic conditions, the concentration of inhaled oxygen needs to be increased. This is possible through the use of supplemental oxygen and/or a decrease in pressure altitude. Normally, recovery is quite rapid once normal inspired oxygen levels have been restored. Tolerance to hypoxia between individuals & the likelihood of experiencing it: -Age: Elderly generally have weaker pulmonary systems than younger people which can make them more susceptible to hypoxia. -Physical fitness level: People with an active lifestyle are less likely to experience hypoxia -Environment acclimation: People who reside at higher elevations are more climatized to lower atmospheric (and oxygen) pressure. -Nutrition: Adequate nutrition helps to increase the tolerance of an individual to hypoxia Tolerance to hypoxia between individuals & the likelihood of experiencing it: -Fatigue: A person is more likely to suffer hypoxia sooner if fatigued. -Alcohol/Drugs: Can make a person more sensitive to hypoxia. -Smoking: Smokers are far more vulnerable to hypoxia. Oxygen Paradox Recovery from Hypoxia usually occurs within seconds after re-establishing a normal alveolar partial pressure. In some instances following the sudden administration of oxygen to correct the hypoxic state, the individual develops a temporary increase in the severity of symptoms. This is known as ‘Oxygen Paradox’. The subject may lose consciousness or develop fits for a period lasting up to a minute. Accompanying symptoms are mental confusion, deterioration of vision, dizziness, and nausea. These are temporary symptoms and the oxygen must not be removed or it could make the hypoxic state worse. Time of Useful Consciousness (TUC) Refer chapter one in ‘Human Being Pilot’ book Physiological Effects Following Loss of Cabin Pressurisation Hypoxia - Significant reduction in O2 partial pressure available for respiration. At 25,000 ft (or FL 250), atmospheric pressure is approximately one-third the value of sea level pressure. Barotrauma - When the air contained in the body’s organs and cavities expands in response to the drop in air pressure. Such occurrences are characterised by pain and discomfort in the affected areas. Decompression sickness - Commonly known as the bends, decompression sickness is often associated with SCUBA diving. This condition occurs whenever the inert gases dissolved in the blood (mainly nitrogen, although other gases can also be affected) bubble out of solution. Altitude sickness - Sometimes referred to as hypobaropathy or acute mountain sickness (AMS). If supplemental oxygen is not introduced and/or altitude is not decreased, hypobaropathy can progress to pulmonary oedema (fluid in the lungs) and/or cerebral oedema (swelling of the brain) - both of which can be lethal. In terms of reference elevations, altitudes above 18,000 ft are considered extreme altitudes. Hypothermia - Based on International Standard Atmosphere (ISA) figures, ambient temperature at 25,000 ft will be approximately -35° C. This extreme cold, especially when combined with the sudden temperature change that accompanies uncontrolled decompression, can exacerbate the onset of hypothermic symptoms. Frostbite - Vasoconstriction begins in the vessels of the body’s extremities in an effort to conserve the body’s core temperature. As temperature continues to plummet, more blood is diverted from the extremities. Below a certain level, this lack of blood flow results in the freezing and death of the blood-deprived tissues, which is known as frostbite. Physical injuries - As flight altitude increases, differential pressure between the cabin and the atmosphere increases. With a high-altitude loss of pressurisation, this pressure differential can cause objects (and passengers) to be thrown about the cabin. The risk of being hit (or of hitting) airborne objects can result in bodily injury. Flight Crew Actions Following Loss of Pressurisation at Altitude - Don personal O2 masks - Verify deployment of cabin masks and confirm the flow of oxygen - Begin an emergency descent: Once supplemental O2 is available and in use, the flight crew must immediately begin an expedited descent to a lower-pressure altitude. The target altitude will vary given the exact circumstances, but will generally be 8,000-10,000 ft, or the minimum safe altitude (if higher). a) Power to flight idle b) Speeds/propeller pitch (if applicable) - low pitch/high RPM setting c) Establish a spiralling descent at 30°-45° angle of bank. d) Depending on company/recommended aircraft procedures, you might be advised to deploy landing gear and/or flaps as their respective deployment /extension speeds are reached. However, other aircraft or circumstances might recommend that you descend in the clean configuration. Always refer to the established procedures for your aircraft/company. e) Airspeed: Your goal is to establish the maximum possible speed without exceeding aircraft structural limitations. Always refer to aircraft/company procedures for specifics to your aircraft and situation. f) Call air traffic control (ATC) - Declare an emergency, advise of your situation/intentions, and seek the maximum available assistance. g) Verify target descent altitude. Hypoxia Hyperventilation Hyperventilation is over breathing in excess of the ventilation required to remove carbon dioxide. Over breathing induces a reduction in the carbon dioxide and thus decreases the carbonic acid balance of the blood. This disturbance of the acid balance has a number of effects, the major one being that haemoglobin gives up its oxygen readily only in an acid medium. Causes of Hyperventilation Emotional/mental causes These can often lead to unconscious increases in breathing rate. Anxiety Fear Stress/emotional tension Panic attacks Physical catalysts Aerobic exercise: During strenuous exercise, the body uses much more oxygen than when at rest. Hyperventilation is a common response, in which the body works to increase its supply of oxygen. Hypoxia: The body attempts to compensate for the decrease in oxygen availability. Cold shock Pain Physiological causes Fever Infections Stroke Aspirin overdose Asthma Early emphysema Hyperthyroidism Meningitis Metabolic acidosis Chronic obstructive pulmonary disease (COPD) Various brain and nervous system diseases/deficiencies Symptoms of Hyperventilation The symptoms of hyperventilation are as follows: Drowsiness Light-headedness / dizziness Shortness of breath / feelings of suffocation Headache Delayed reaction time Impaired judgment Slurred speech Chest pain Euphoria Numbness Tingling in the extremities Clammy / pale appearance Muscle spasms Eventual unconsciousness (if corrective measures are not taken). Treating Hyperventilation The most effective step to promote recovery from hyperventilation is to slow the breathing rate, as this allows the body to restore the proper balance of carbon dioxide. Consciously slow the rate of respiration Talk aloud / sing Possible use of medication (depending on the underlying cause(s)) Lifestyle changes / stress-reduction techniques Differences between Hyperventilation and Hypoxia Hypoxia: A state of insufficient oxygen being delivered to or received / utilised by parts of (or the entire) body. Characteristic indications of hypoxia include limp, flaccid muscles, and a bluish colouration in the extremities known as cyanosis. Hyperventilation: A condition of inadequate carbon dioxide in the body generally caused by excessive respiration. Symptoms of hyperventilation might include spastic muscles and a clammy/pale appearance. Entrapped Gases Barotrauma Defined Barotrauma is a term for the various types of tissue damage that can result from a strong pressure gradient between an air-filled body cavity and the ambient environment (which can be air or water). Barotraumatic injuries are most often associated with aircraft occupants who are exposed to airborne loss of pressurisation and with SCUBA divers. Causes of Barotrauma Pressure changes: -The inverse pressure/volume relationship described by Boyle’s Law (i.e., as a fluid’s pressure increases, its volume decreases (and vice versa)). While gases can be compressed, the body’s tissues cannot. As a result, sudden drops in atmospheric pressure cause air-filled cavities to expand, placing increased stress on the organs/tissues in question. -Rapid increases in external pressure lead to a reduction in the volume of air in such cavities / tissues, resulting in a squeezing effect on the body and its organs. If the affected cavities are unable to equalise the internal air pressure with ambient conditions, possible complications and injury can result. A person is more likely to experience pain on descent than ascent. Ascent - Air inside the cabin is pressurised, but at a lower pressure than the ear - this is why you get a blocked ear feeling as the pressure in the ears hasn’t equalised. The Eustachian tube opens letting air out from the middle ear, equalising the pressure. Some of the air is absorbed into the bloodstream, via the ear cavity lining. The process is usually not painful. Descent - External pressures increase rapidly and forcing the eardrum to bulge inwards, stretching the eardrum which can be very painful. Usually this is due to a respiratory infection. The four main areas in the human body which contain trapped gas: Behind the eardrum In the Middle Ear The Sinuses Stomach. Increase in altitude: -Reduction in pressure and gas expands -Increase in pressure differential across wall of whatever is trapping it Decrease in altitude: -Increase in pressure, gas contracts and creates a vacuum Pain can be experienced in any of the above body areas on the climb or descent but more likely: Descent: -Middle ear -Sinuses Ascent: -Sinuses -Stomach Aero-odontalgia Aero-odontalgia refers to symptoms related to various dental ailments due to change in ambient pressure in flight. It commonly presents as pain in tooth due to trapped air in a dental cavity after a filling has been done. Other reasons for dental pain could be due to infection of the gums or the root canal or even referred pain from maxillary sinus (Sinus Barotrauma). Aero-odontalgia can be prevented by regular dental care and periodic dental check up. Symptoms of Barotrauma Lungs: A very important consideration to remember is that the lungs do not sense pain, so a person experiencing abnormal lung expansion may be unaware of impending injury or damage. Ears: Severe pain, hearing loss (temporary or permanent), ringing in the ears (tinnitus), ear infections, bleeding ears, and vertigo are all possible symptoms of barotrauma. Sinuses: Excruciating pain in the upper cheeks (maxillary sinuses), above the eyebrows (frontal sinuses), and in the upper teeth (barodontalgia) are all indications of barotraumatic exposure. Nosebleeds (epistaxis) are another common sign of barotrauma in the paranasal sinuses. Stomach: As you ascend the gases in your stomach can expand and may cause abdominal distention and stomach cramps. Preventing Barotrauma Rate of climb / descent: Whether flying or scuba diving, the best safeguard against barotrauma is to use a gradual rate of ascent / descent. Doing so allows the body sufficient time to adjust to changes in ambient pressure. Pressure suit: If flying at significantly high altitudes, wearing a pressure suit can help protect the body by pressurising certain areas of the body to protect against exposure to low atmospheric pressure conditions. Consciously equalise pressure: -Open your sinuses and Eustachian tubes during climb and descent. Swallowing, yawning, chewing of gum, and performing the Valsalva manoeuvre (closing the mouth, pinching the nose, and blowing) are effective methods to open the Eustachian tubes. For infants, nursing during climb and descent can help to clear the airways. Avoid flying with certain medical conditions: Whenever possible, do not fly when suffering from nasal, ear, or respiratory conditions. Treating Barotrauma Hyperbaric oxygen chamber: Hyperbaric oxygen therapy has long been used to treat decompression sickness. This form of treatment is effective because the administered O2 helps the body to re-absorb the inert gas that has bubbled out of solution. Medical treatment: Decongestants, painkillers, and, in severe cases, surgery, might be necessary to treat aerosinusitis. Penicillin and anti-dizziness medications have been used for aerotitis. When permanent hearing loss results from barotrauma, hearing aids are often required. In cases of extreme barodontalgia, dental procedures could be needed to correct barotraumatic damage. Altitude adjustment (when feasible): Many conditions (notably sinus block) are common during rapid descents. If you or another aircraft occupant experiences pain or other symptoms of barotrauma, stop the descent. In most cases, initiating a gradual climb will help alleviate the pressure differential. Before recommencing descent, notify air traffic control of the situation and insist on a shallow descent profile. Decompression Sickness - When nitrogen in the body comes out of solution as small bubbles. - Happens when ambient pressure is abruptly reduced. - Ultimately the individual may collapse and in rare cases DCS may occur or persist after descent and go on to cause DEATH. Hypoxia and cold increase the risk as does age and excess body mass/obesity. Symptoms Joints. Bubbles in the joints (shoulders, elbows, wrists, knees and ankles) cause rheumatic-like pains called the bends. In aviation the shoulder, wrist, knee and ankles are most commonly affected. Movement or rubbing the affected parts only aggravates the pain but descent usually resolves the problem. Skin. Nitrogen bubbles released under the skin causes the creeps when the sufferer feels that a small compact colony of ants are crawling over, or just under, the skin. Respiratory system. This is known as the chokes. Nitrogen bubbles may get caught in the capillaries of the lungs blocking the pulmonary blood flow. This leads to serious shortness of breath accompanied by a burning, gnawing and sometimes piercing pain. Brain. The bubbles affect the blood supply to the brain and the nervous system. This effect is known as the staggers. The sufferer will lose some mental functions and control of movement. In extreme cases chronic paralysis or even permanent mental disturbances may result. The secondary symptom is: Post descent collapse. This may occur up to four hours after the primary symptoms when nitrogen bubbles have combined and therefore not gone back into solution and have reached the heart. Avoided by Preoxygenation before exposure to high altitudes, thus reducing the body store of nitrogen as much as possible. DCS in Flight and Treatment If the symptoms of DCS appear in any passenger or crew member, the pilot should commence an immediate descent to a level at which the symptoms are relieved. The aircraft should land as soon as possible. Meanwhile the sufferer should be kept warm and rested and put onto a 100% oxygen supply. Urgent medical assistance must be sought on landing even if the patient appears to have recovered. Flying and Diving DCS is rare but the incidence is greatly increased for individuals who have been diving, using compressed air, shortly before a flight. The pressure that a 30 ft column of sea water exerts is the same as that exerted by the atmosphere at sea level (i.e. 760 mm Hg). Therefore a person at a depth of 30 ft is exposed to a pressure of 2 atmospheres (1 atmosphere caused by the air above the water and the other by the water itself). In scuba diving, air under pressure is used and this increases the amount of nitrogen in the body. Recommended Intervals between Diving and Flying Whilst there are no legal requirements for the time interval between flying and diving, there are recommendations that one should follow to keep oneself safe: Do not fly for at least 24 hours after the last dive if at all possible. But if not possible, - Dive of 10 m or less: Do not fly for 12 hours after the dive. - Dive of greater than 10 m: Do not fly for 24 hours after the dive. - Dive of greater than 55 m: Do not fly for 48 hours after the dive - If you intend to fly above 8,000 ft AMSL: Do not fly for 48 hours after the dive. (Most pressurised aircraft pressurise their cabins to around 8,000 ft AMSL) The effects of explosive decompression on the body: The main danger to flight crew and passengers, apart from possible ejection from the aircraft, is inadequate oxygen, or hypoxia. The symptoms of early-stage hypoxia are different from those of decompression sickness and include - Stomach pain or nausea - Tingling in the extremities - Blue tinge (cyanosis) of lips and fingernails - Rapid breathing - Headache - Nausea - Dizziness - Euphoria - Loss of consciousness - Death Actions to take when dealing with explosive decompression: - Ensure your restraints are secure - Put your oxygen mask on immediately - Assist anyone else who may need help - Descend to a lower altitude (10,000ft) as soon as possible - Try to remain calm!