Harper's Biochemistry Chapter 22 - Oxidation of Fatty Acids (Ketogenesis) PDF
Document Details
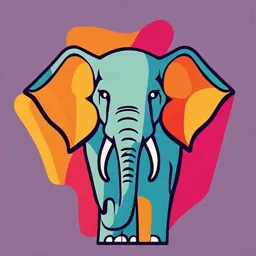
Uploaded by PrizeMeerkat
Kathleen M. Botham, PhD, DSc, & Peter A. Mayes, PhD, DSc
Tags
Summary
This chapter from Harper's Biochemistry discusses the oxidation of fatty acids and the process of ketogenesis. It covers the metabolic pathways and processes involved, including the activation, transport, and breakdown of fatty acids within mitochondria, and the production of ketone bodies.
Full Transcript
C H A P T E R Oxidation of Fatty Acids: Ketogenesis Kathleen M. Botham, PhD, DSc, & Peter A. Mayes, PhD, DSc 22 O B J E C TI V E S Describe the processes by which fat...
C H A P T E R Oxidation of Fatty Acids: Ketogenesis Kathleen M. Botham, PhD, DSc, & Peter A. Mayes, PhD, DSc 22 O B J E C TI V E S Describe the processes by which fatty acids are transported in the blood, activated and transported into the matrix of the mitochondria for breakdown After studying this chapter, you to obtain energy. should be able to: Outline the β-oxidation pathway by which fatty acids are metabolized to acetyl- CoA and explain how this leads to the production of large quantities of ATP. Identify the three compounds termed “ketone bodies” and describe the reactions by which they are formed in liver mitochondria. Recognize that ketone bodies are important fuels for extrahepatic tissues and indicate the conditions in which their synthesis and use are favored. Indicate the three stages in the metabolism of fatty acids where ketogenesis is regulated. Indicate that overproduction of ketone bodies leads to ketosis and, if prolonged, ketoacidosis, and identify pathologic conditions when this occurs. Give examples of diseases associated with impaired fatty acid oxidation. BIOMEDICAL IMPORTANCE OXIDATION OF FATTY ACIDS Fatty acis are broken own in mitochonria by oxiation OCCURS IN MITOCHONDRIA to acety-CoA in a process that generates arge amounts of Athough acety-CoA is both an en point of fatty aci catabo- energy. When this pathway is proceeing at a high rate, three ism an the starting substrate for fatty aci synthesis, break- compouns, acetoacetate, d-3-hyroxybutyrate, an ace- own is not simpy the reverse of the biosynthetic pathway, tone, known coectivey as the ketone boies, are prouce but an entirey separate process taking pace in a ifferent by the iver. Acetoacetate an d-3-hyroxybutyrate are use as compartment of the ce. The separation of fatty aci oxia- fues by extrahepatic tissues in norma metaboism, but over- tion in mitochonria from biosynthesis in the cytoso aows prouction of ketone boies causes ketosis. Increase fatty each process to be iniviuay controe an integrate with aci oxiation an consequenty ketosis is a characteristic of tissue requirements. Each step in fatty aci oxiation invoves starvation an of iabetes meitus. Since ketone boies are acy-CoA erivatives, is catayze by separate enzymes, uti- aciic, when they are prouce in excess over ong perios, izes NAD+ an FAD as coenzymes, an generates ATP. It is an as in iabetes, they cause ketoaciosis, which is potentiay aerobic process, requiring the presence of oxygen. ife-threatening. Because guconeogenesis is epenent on fatty aci oxiation, any impairment in fatty aci oxiation eas to hypoglycemia. This occurs in various states of car- Fatty Acids Are Transported in the nitine eficiency or eficiency of essentia enzymes in fatty Blood as Free Fatty Acids aci oxiation, for exampe, carnitine palmitoyltransferase, Free fatty acis (FFAs)—aso cae unesterifie (UFA) or non- or inhibition of fatty aci oxiation by poisons, for exampe, esterifie (NEFA) fatty acis (see Chapter 21)—are fatty acis hypoglycin. that are in the unesterifie state. In pasma, onger-chain FFA 217 218 SECTION V Metabolism of Lipids are combine with albumin, an in the ce they are attache Long-Chain Fatty Acids Cross the to a fatty aci–bining protein, so that in fact they are never reay “free.” Shorter-chain fatty acis are more water soube Inner Mitochondrial Membrane as an exist as the unionize aci or as a fatty aci anion. Carnitine Derivatives Acy-CoAs forme as escribe earier enter the intermem- Fatty Acids Are Activated Before brane space (see Figure 22–1), but are unabe to cross the inner Being Catabolized mitochonria membrane into the matrix where fatty aci breakown takes pace. In the presence of carnitine (β-hyroxy- Fatty acis must first be converte to an active intermeiate γ-trimethyammonium butyrate), a compoun wiey istrib- before they can be cataboize. This is the ony step in the ute in the boy an particuary abunant in musce; however, compete egraation of a fatty aci that requires energy from carnitine palmitoyltransferase-I, an enzyme ocate in the ATP. In the presence of ATP an coenzyme A, the enzyme outer mitochonria membrane, transfers the ong-chain acy acyl-CoA synthetase (thiokinase) catayzes the conversion group from CoA to carnitine, forming acylcarnitine an reeas- of a fatty aci (or FFA) to an “active fatty aci” or acyl-CoA, ing CoA. Acycarnitine is abe to penetrate the inner membrane using one high-energy phosphate an forming AMP an PPi an gain access to the β-oxiation system of enzymes via the (Figure 22–1). The PPi is hyroyze by inorganic pyrophos- inner membrane exchange transporter carnitine-acylcarnitine phatase with the oss of a further high-energy phosphate, translocase. The transporter bins acycarnitine an transports ensuring that the overa reaction goes to competion. Acy-CoA it across the membrane in exchange for carnitine. The acy synthetases are foun on the outer membrane of mitochonria group is then transferre to CoA so that acy-CoA is reforme an aso in the enopasmic reticuum an peroxisomes. an carnitine is iberate. This reaction is catayze by carnitine palmitoyltransferase-II, which is ocate on the insie of the inner membrane (see Figure 22–1). a-OXIDATION OF FATTY ACIDS INVOLVES SUCCESSIVE CLEAVAGE WITH RELEASE OF ACETYL-COA In the pathway for the oxiation of fatty acis (Figure 22–2), two carbons at a time are ceave from acy-CoA moecues, starting at the carboxy en. The chain is broken between the α(2)- an β(3)-carbon atoms—hence the process is terme β-oxiation. The two-carbon units forme are acety-CoA; thus, pamitoy(C16)-CoA forms eight acety-CoA moecues. FIGURE 22–1 Role of carnitine in the transport of long-chain fatty acids through the inner mitochondrial membrane. Long- chain acyl-CoA formed by acyl-CoA synthetase enters the intermem- brane space. For transport across the inner membrane, acyl groups must be transferred from CoA to carnitine by carnitine palmitoyl- transferase-I. The acylcarnitine formed is then carried into the matrix by a translocase enzyme in exchange for a free carnitine and acyl-CoA is reformed by carnitine palmitoyltransferase-II. FIGURE 22–2 Overview of β-oxidation of fatty acids. CHAPTER 22 Oxidation of Fatty Acids: Ketogenesis 219 The β-Oxidation Cycle Generates FADH2 & NADH Severa enzymes foun in the mitochonria matrix or inner membrane ajacent to the respiratory chain catayze the oxia- tion of acy-CoA to acety-CoA via the β-oxiation pathway. The system procees in cycic fashion which resuts in the eg- raation of ong fatty acis to acety-CoA. In the process, arge quantities of the reucing equivaents FADH2 an NADH are generate an are use to form ATP by oxiative phosphorya- tion (see Chapter 13) (Figure 22–3). The first step is the remova of two hyrogen atoms from the 2(α)- an 3(β)-carbon atoms, catayze by acyl-CoA ehyro- genase an requiring favin aenine inuceotie (FAD). This resuts in the formation of Δ2-trans-enoy-CoA an FADH2. Next, water is ae to saturate the oube bon an form 3-hyroxyacy-CoA, catayze by Δ2-enoyl-CoA hyratase. The 3-hyroxy erivative unergoes further ehyrogenation on the 3-carbon catayze by l-3-hyroxyacyl-CoA ehyro- genase to form the corresponing 3-ketoacy-CoA compoun. In this case, NAD+ is the coenzyme invove. Finay, 3-ketoacy- CoA is spit at the 2,3-position by thiolase (3-ketoacy-CoA- thioase), forming acety-CoA an a new acy-CoA two carbons shorter than the origina acy-CoA moecue. The shorter acy-CoA forme in the ceavage reaction reenters the oxiative pathway at reaction 2 (see Figure 22–3). In this way, a ong-chain fatty aci with an even number of carbons may be egrae competey to acety-CoA (C2 units). For exampe, after seven cyces, the C16 fatty aci, pamitate, wou be con- verte to eight acety-CoA moecues. Since acety-CoA can be oxiize to CO2 an water via the citric aci cyce (which is aso foun within the mitochonria), the compete oxiation of fatty acis is achieve. Fatty acis with an o number of carbon atoms are oxi- ize by the pathway of β-oxiation escribe earier, prouc- ing acety-CoA unti a three-carbon (propiony-CoA) resiue remains. This compoun is converte to succiny-CoA, a con- stituent of the citric aci cyce (see Chapter 16). Hence, the propionyl resiue from an o-chain fatty aci is the only part of a fatty aci that is glucogenic. Oxidation of Fatty Acids Produces a Large Quantity of ATP Each cyce of β-oxiation generates one moecue of FADH2 an one of NADH. The breakown of 1 mo of the C16 fatty aci, pamitate, requires seven cyces an prouces 8 mo of acety- CoA. Oxiation of the reucing equivaents via the respiratory chain eas to the synthesis of 28 mo of ATP (Table 22–1 an see Chapter 13) an oxiation of acety-CoA via the citric aci FIGURE 22–3 β-Oxidation of fatty acids. Long-chain acyl- cyce prouces 80 mo of ATP (see Tabe 22–1 an Chapter 16). CoA is cycled through reactions 2 to 5 , acetyl-CoA being split off, The breakown of 1 mo of pamitate, therefore, yies a gross each cycle, by thiolase (reaction 5 ). When the acyl radical is only tota of 108 mo of ATP. However, two high-energy phosphates four carbon atoms in length, two acetyl-CoA molecules are formed in reaction 5. are use in the initia activation step (see Figure 22–3), thus there is a net gain of 106 mo of ATP per moe of pamitate use 220 SECTION V Metabolism of Lipids TABLE 22–1 Generation of ATP From the Complete Oxidation of a C16 Fatty Acid Amount Product Formed (mol)/mol ATP Formed (mol)/ Total ATP Formed ATP Used (mol)/ Step Product Palmitate mol Product (mol)/mol Palmitate mol Palmitate Activation – 2 β-Oxidation FADH2 7 1.5 10.5 – β-Oxidation NADH 7 2.5 17.5 – Citric acid cycle Acetyl-CoA 8 10 80 – Total ATP formed (mol)/mol palmitate 108 Total ATP used (mol)/mol palmitate 2 The table shows how the oxidation of 1 mol of the C16 fatty acid, palmitate, generates 106 mol of ATP (108 formed in total—2 used in the activation step). (see Tabe 22–1), or 106 × 30.5* = 3233 kJ. This represents 33% KETOGENESIS OCCURS WHEN of the free energy of combustion of pamitic aci. THERE IS A HIGH RATE OF FATTY ACID OXIDATION IN THE LIVER Peroxisomes Oxidize Very-Long-Chain Uner metaboic conitions associate with a high rate of Fatty Acids fatty aci oxiation, the iver prouces consierabe quantities A moifie form of β-oxiation is foun in peroxisomes of acetoacetate an d-3-hyroxybutyrate (3-hyroxybutyrate or an eas to the breakown of very-ong-chain fatty acis β-hyroxybutyrate). Acetoacetate continuay unergoes spon- (eg, C20, C22) with the formation of acety-CoA an H 2O2, taneous ecarboxyation to yie acetone. These three sub- which is broken own by cataase (see Chapter 12). This sys- stances are coectivey known as the ketone boies (aso cae tem, however, is not inke irecty to phosphoryation an acetone boies or [incorrecty*] “ketones”) (Figure 22–5). the generation of ATP. The peroxisoma enzymes are inuce Acetoacetate an 3-hyroxybutyrate are interconverte by the by high-fat iets an in some species by hypoipiemic rugs mitochonria enzyme d-3-hyroxybutyrate ehyrogenase; such as cofibrate. the equiibrium is controe by the mitochonria [NAD+]/ Another roe of peroxisoma β-oxiation is to shorten [NADH] ratio, that is, the reox state. The concentration of the sie chain of choestero in bie aci formation (see tota ketone boies in the boo of we-fe mammas oes Chapter 26). Peroxisomes aso take part in the synthesis of not normay excee 0.2 mmo/L. However, in ruminants, ether gyceroipis (see Chapter 24), choestero, an oi- 3-hyroxybutyrate is forme continuousy from butyric aci cho (see Figure 26–2). (a prouct of rumina fermentation) in the rumen wa. In nonruminants, the iver appears to be the ony organ that as significant quantities of ketone boies to the boo. Extra- Oxidation of Unsaturated Fatty Acids hepatic tissues utiize acetoacetate an 3-hyroxybutyrate as respiratory substrates. Acetone is a waste prouct which, as it Occurs by a Modified β-Oxidation is voatie, can be excrete via the ungs. Because there is active Pathway synthesis but itte utiization of ketone boies in the iver, The CoA esters of unsaturate fatty acis are egrae by the whie they are use but not prouce in extrahepatic tissues, enzymes normay responsibe for β-oxiation unti there is a there is a net fow of the compouns to the extrahepatic tissues cis oube bon in the Δ3 or Δ4 position (Figure 22–4). A Δ3- (Figure 22–6). cis compoun is isomerize (Δ 3cis → Δ2-trans-enoyl-CoA isomerase) to the corresponing Δ2-trans-CoA stage of β-oxiation for subsequent hyration an oxiation. Any Acetoacetyl-CoA Is the Substrate for Δ4-cis-acy-CoA either remaining, as in the case of inoeic Ketogenesis aci (shown in Figure 22–4), or entering the pathway at this The enzymes responsibe for ketone boy formation (ketogen- point after conversion by acy-CoA ehyrogenase to Δ 2- esis) are associate mainy with the mitochonria. Acetoace- trans-Δ 4-cis-ienoy-CoA, is then metaboize as inicate ty-CoA is forme when two acety-CoA moecues prouce in Figure 22–4. via fatty aci breakown conense to form acetoacety-CoA *The term ketones shou not be use as there are ketones in boo *ΔG for the ATP reaction, as expaine in Chapter 11. that are not ketone boies, for exampe, pyruvate an fructose. CHAPTER 22 Oxidation of Fatty Acids: Ketogenesis 221 O CH3 C CH3 CO2 Acetone us eo tan on Sp O CH3 C CH2 COO– Acetoacetate D-3-Hydroxybutyrate dehydrogenase NADH + H+ OH NAD+ CH3 CH CH2 COO– D-3-Hydroxybutyrate FIGURE 22–5 Interrelationships of the ketone bodies. d-3-Hydroxybutyrate dehydrogenase is a mitochondrial enzyme. by a reversa of the thiolase reaction (see Figure 22–3), an may aso arise irecty from the termina four carbons of a fatty aci uring β-oxiation (Figure 22–7). Conensation of acetoacety-CoA with another moecue of acety-CoA by 3-hyroxy-3-methylglutaryl-CoA (HMG-CoA) synthase forms HMG-CoA. HMG-CoA lyase then causes acety-CoA to spit off from the HMG-CoA, eaving free acetoacetate. Both enzymes must be present in mitochonria for ketogenesis to take place. In mammas, ketone boies are forme soey in the iver an in the rumen epitheium. 3-Hyroxybutyrate is forme from acetoacetate (see Figure 22–7) an is quantita- tivey the preominant ketone boy present in the boo an urine in ketosis. Ketone Bodies Serve as a Fuel for Extrahepatic Tissues Whie an active enzymatic mechanism prouces acetoacetate from acetoacety-CoA in the iver, acetoacetate once forme can ony be reactivate by inkage to CoA irecty in the cyto- so, where it is use in a ifferent, much ess active pathway as a precursor in choestero synthesis (see Chapter 26). This accounts for the net prouction of ketone boies by the iver. In extrahepatic tissues, acetoacetate is activate to aceto- acety-CoA by succinyl-CoA-acetoacetate-CoA transferase. CoA is transferre from succiny-CoA to form acetoacety- FIGURE 22–4 Sequence of reactions in the oxidation of CoA (Figure 22–8). In a reaction requiring the aition of unsaturated fatty acids, for example, linoleic acid. β-Oxidation a CoA, two acety-CoA moecues are forme by the spitting proceeds as for saturated fatty acids until there is a cis double bond in the Δ3 position. This is then isomerized to the corresponding Δ2-trans of acetoacety-CoA by thioase an these are oxiize in the compound allowing one cycle of β-oxidation to proceed, producing citric aci cyce. 3-Hyroxybutyrate is utiize by conversion the Δ2- trans- Δ4-cis derivative. Δ4-cis-fatty acids or fatty acids forming to acetoacetate by the reversa of the reaction by which it is Δ4-cis-enoyl-CoA enter the pathway here. A reduction step forming forme in the iver, generating an NADH in the process (see Δ3-trans-enoyl-CoA followed by an isomerization to the Δ2-trans form Figure 22–8). Thus, 1 mo of acetoacetate or 3-hyroxbutyrate is required to enable β-oxidation to then go to completion. NADPH for the dienoyl-CoA reductase step is supplied by intramitochondrial yies 19 or 21.5 mo of ATP, respectivey, by these pathways. sources such as glutamate dehydrogenase, isocitrate dehydrogenase, If the boo eve of ketone boies rises to a concentration of and NAD(P)H transhydrogenase. ~12 mmo/L, the oxiative machinery becomes saturate an 222 SECTION V Metabolism of Lipids Extrahepatic Liver Blood tissues Acyl-CoA FFA Glucose Glucose Acyl-CoA Acetyl-CoA Urine Acetyl-CoA Ketone Ketone Ketone bodies bodies bodies Acetone Citric Citric acid acid cycle cycle Lungs 2CO2 2CO2 FIGURE 22–6 Formation, utilization, and excretion of ketone bodies. (The main pathway is indicated by the solid arrows.) FIGURE 22–7 Pathways of ketogenesis in the liver. (FFA, free fatty acids.) CHAPTER 22 Oxidation of Fatty Acids: Ketogenesis 223 FIGURE 22–8 Transport of ketone bodies from the liver and pathways of utilization and oxidation in extrahepatic tissues. CoA transferase, succinyl-CoA-acetoacetate-CoA transferase. The breakdown of acetoacetyl-CoA by thiolase produces two acetyl-CoA molecules and requires the addition of one CoA (not shown). at this stage, a arge proportion of oxygen consumption may be to increase. Malonyl-CoA, the initia intermeiate in fatty accounte for by their oxiation. aci biosynthesis (see Figure 23–1) is a potent inhibitor In moerate ketonemia, the oss of ketone boies via the of CPT-I (Figure 22–10). In the fe state, therefore, FFAs urine is ony a few percent of the tota ketone boy prouc- tion an utiization. Since there are rena thresho-ike effects (there is not a true thresho) that vary between species an iniviuas, measurement of the ketonemia, not the ketonuria, is the preferre metho of assessing the severity of ketosis. KETOGENESIS IS REGULATED AT THREE CRUCIAL STEPS 1. Ketosis oes not occur in vivo uness there is an increase in the eve of circuating FFAs arising from ipoysis of tria- cygycero in aipose tissue. FFAs are the precursors of ketone boies in the liver. Both in fe an in fasting coni- tions, the iver extracts ~30% of the FFAs passing through it, so that at high concentrations the fux passing into the organ is substantia. Thus, the factors regulating mobili- zation of FFA from aipose tissue are important in con- trolling ketogenesis (Figures 22–9 an 25–8). 2. After uptake by the iver, FFAs are either oxiize to CO2 or ketone boies or esterifie to triacygycero an phos- phoipi (acygyceros). There is reguation of entry of fatty acis into the oxiative pathway by carnitine palmitoyl- transferase-I (CPT-I) (see Figure 22–1), an the remainer FIGURE 22–9 Regulation of ketogenesis. 1 to 3 show of the fatty aci taken up is esterifie. CPT-I activity is ow three crucial steps in the pathway of metabolism of free fatty acids in the fe state, eaing to epression of fatty aci oxia- (FFA) that determine the extent of ketogenesis. (CPT-I, carnitine tion, an high in starvation, aowing fatty aci oxiation palmitoyltransferase-I.) 224 SECTION V Metabolism of Lipids FIGURE 22–10 Regulation of long-chain fatty acid oxidation in the liver. (FFA, free fatty acids; VLDL, very-low-density lipoprotein.) Positive ( ) and negative () regulatory effects are represented by broken arrows and substrate flow by solid arrows. enter the iver ce in ow concentrations an are neary a that aows the iver to oxiize increasing quantities of fatty esterifie to acygyceros an transporte out of the iver acis within the constraints of a tighty coupe system of in very-low-ensity lipoprotein (VLDL). However, as the oxiative phosphoryation. concentration of FFA increases with the onset of starvation, A fa in the concentration of oxaoacetate, particuary within acety-CoA carboxyase is inhibite irecty by acy-CoA, the mitochonria, can impair the abiity of the citric aci an (maony-CoA) ecreases, reeasing the inhibition of cyce to metaboize acety-CoA an ivert fatty aci oxia- CPT-I an aowing more acy-CoA to be β-oxiize. These tion towar ketogenesis. Such a fa may occur because of an events are reinforce in starvation by a ecrease in the increase in the (NADH)/(NAD+) ratio cause when increase (insulin)/(glucagon) ratio. Thus, β-oxiation from FFA β-oxiation aters the equiibrium between oxaoacetate an is controe by the CPT-I gateway into the mitochonria, maate so that the concentration of oxaoacetate is ecrease, an the baance of the FFA uptake not oxiize is esterifie. an aso when guconeogenesis is eevate ue to ow boo 3. In turn, the acety-CoA forme in β-oxiation is oxiize gucose eves. The activation by acety-CoA of pyruvate car- in the citric aci cyce, or it enters the pathway of ketogen- boxyase, which catayzes the conversion of pyruvate to oxa- esis via acetoacety-CoA to form ketone boies. As the eve oacetate, partiay aeviates this probem, but in conitions of serum FFA is raise, proportionatey more of the acety- such as starvation an untreate iabetes meitus, ketone CoA prouce from their breakown is converte to ketone boies are overprouce an cause ketosis. boies an ess is oxiize via the citric aci cyce to CO2. The partition of acety-CoA between the ketogenic pathway CLINICAL ASPECTS an the pathway of oxiation to CO2 is reguate so that the tota free energy capture in ATP which resuts from the Impaired Oxidation of Fatty Acids oxiation of FFA remains constant as their concentration in the serum changes. This may be appreciate when it is rea- Gives Rise to Diseases Often ize that compete oxiation of 1 mo of pamitate invoves Associated With Hypoglycemia a net prouction of 106 mo of ATP via β-oxiation an Carnitine eficiency can occur particuary in the newborn— the citric aci cyce (see earier), whereas ony 26 mo of an especiay in preterm infants—owing to inaequate ATP are prouce when acetoacetate is the en prouct biosynthesis or rena eakage. Losses can aso occur in hemo- an ony 16 mo when 3-hyroxybutyrate is the en pro- iaysis. This suggests there may be a vitamin-ike ietary uct. Thus, ketogenesis may be regare as a mechanism requirement for carnitine in some iniviuas. Symptoms of CHAPTER 22 Oxidation of Fatty Acids: Ketogenesis 225 eficiency incue hypogycemia, which is a consequence of epetion of avaiabe carbohyrate coupe with mobiization impaire fatty aci oxiation, an ipi accumuation with of FFA. An exaggeration of this genera pattern of metaboism muscuar weakness. Treatment is by ora suppementation prouces the pathoogic states foun in iabetes mellitus, the with carnitine. type 2 form of which is increasingly common in Western Inherite CPT-I eficiency affects ony the iver, resuting countries; twin lamb isease; an ketosis in lactating cattle. in reuce fatty aci oxiation an ketogenesis, with hypo- Nonpathoogic forms of ketosis are foun uner conitions of gycemia. CPT-II eficiency affects primariy skeeta musce high-fat feeing an after severe exercise in the postabsorptive an, when severe, the iver. The sufonyurea rugs (glyburie state. [glibenclamie] an tolbutamie), use in the treatment Acetoacetic an 3-hyroxybutyric acis are both moer- of Type 2 iabetes meitus, reuce fatty aci oxiation an, atey strong acis an are buffere when present in boo or therefore, hypergycemia by inhibiting CPT-I. other tissues. However, their continua excretion in quantity Inherite efects in the enzymes of β-oxiation an keto- progressivey epetes the akai reserve, causing ketoaciosis. genesis aso ea to nonketotic hypogycemia, coma, an fatty This may be fata in uncontroe iabetes mellitus. iver. Defects have been ientifie in ong- an short-chain 3-hyroxyacy-CoA ehyrogenase (eficiency of the ong- chain enzyme may be a cause of acute fatty liver of pregnancy). SUMMARY 3-Ketoacyl-CoA thiolase an HMG-CoA lyase eficiency Fatty aci oxiation in mitochonria eas to the generation aso affect the egraation of eucine, a ketogenic amino aci of arge quantities of ATP by a process cae β-oxiation that (see Chapter 29). ceaves acety-CoA units sequentiay from fatty acy chains. Jamaican vomiting sickness is cause by eating the unripe The acety-CoA is oxiize in the citric aci cyce, generating fruit of the akee tree, which contains the toxin hypoglycin. further ATP. This inactivates meium- an short-chain acy-CoA ehy- The ketone boies (acetoacetate, 3-hyroxybutyrate, an rogenase, inhibiting β-oxiation an causing hypogycemia. acetone) are forme in hepatic mitochonria when there is a Dicarboxylic aciuria is characterize by the excretion of high rate of fatty aci oxiation. The pathway of ketogenesis C6—C10 ω-icarboxyic acis an by nonketotic hypogycemia, invoves synthesis an breakown of HMG-CoA by two key enzymes: HMG-CoA synthase an HMG-CoA yase. an is cause by a ack of mitochonria meium-chain acyl- CoA ehyrogenase. Refsum isease is a rare neuroogic Ketone boies are important fues in extrahepatic tissues. isorer cause by a metaboic efect that resuts in the accu- Ketogenesis is reguate at three crucia steps: (1) contro muation of phytanic aci, which is foun in airy proucts of FFA mobiization from aipose tissue; (2) the activity of an ruminant fat an meat. Phytanic aci is thought to have carnitine pamitoytransferase-I in iver, which etermines the pathoogic effects on membrane function, protein preny- proportion of the fatty aci fux that is oxiize rather than esterifie; an (3) partition of acety-CoA between the pathway ation, an gene expression. Zellweger (cerebrohepatorenal) of ketogenesis an the citric aci cyce. synrome occurs in iniviuas with a rare inherite absence of peroxisomes in a tissues. They accumuate C26—C38 poy- Diseases associate with impairment of fatty aci oxiation ea to hypogycemia, fatty infitration of organs, an enoic acis in brain tissue an aso exhibit a generaize oss of hypoketonemia. peroxisoma functions. The isease causes severe neuroogic symptoms, an most patients ie in the first year of ife. Ketosis is mi in starvation but severe in iabetes meitus an ruminant ketosis. Ketoacidosis Results From Prolonged Ketosis REFERENCES Higher than norma quantities of ketone boies present in Ejami AS: Lipid Biochemistry: For Medical Sciences. iUniverse, the boo or urine constitute ketonemia (hyperketonemia) or 2015. ketonuria, respectivey. The overa conition is cae ketosis. Gurr MI, Harwoo JL, Frayn KN, et a: Lipids, Biochemistry, The basic form of ketosis occurs in starvation an invoves Biotechnology and Health. Wiey-Backwe 2016.