Haematology PDF
Document Details
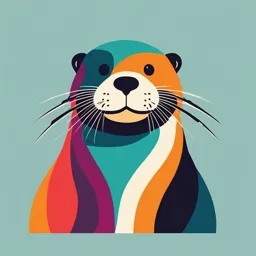
Uploaded by RobustPoisson
Tags
Summary
This document provides an overview of haematology, focusing on the learning outcomes, introduction to the subject, haematopoiesis, blood components, and the role of the red blood cells.
Full Transcript
PART ONE LEARNING OUTCOMES: 1) Explain the basic principles of red cell production in the bone marrow 2) Explain the role of the red cell membrane, metabolic and structural proteins in red cell function and pathology 3) Explain the role of haemoglobin in oxygen transport, and how/the effect of ha...
PART ONE LEARNING OUTCOMES: 1) Explain the basic principles of red cell production in the bone marrow 2) Explain the role of the red cell membrane, metabolic and structural proteins in red cell function and pathology 3) Explain the role of haemoglobin in oxygen transport, and how/the effect of haemoglobin abnormalities occur in human disease 4) Explain the principles of iron metabolism and the relevance of hepcidin INTRODUCTION TO HAEMATOLOGY: The haematological system refers to the blood, blood vessels and the blood-forming organs. Haematologists often wear two hats: clinicians who diagnose and treat conditions and working in the laboratory. HAEMATOPOIESIS PRODUCES THE BLOOD: Haematopoiesis is the process of blood cell formation from a precursor pluripotent haematopoietic stem cell (HSC) in the bone marrow. Blood is a composite body fluid that serves multiple purposes: ○ Blood is the primary mechanism by which oxygen and nutrients are delivered to body tissues and waste products like carbon dioxide and metabolic byproducts are removed. ○ Blood helps regulate body temperature by distributing heat, maintains pH balance through buffer systems and regulates fluid balance in tissues via osmotic pressure. ○ Bloods provide a medium by which WBCs, platelets and antibodies can provide defence against infections and perturbations to homeostasis. ACELLULAR COMPONENT OF BLOOD: ○ Plasma (55% of blood volume) refers to the liquid portion of blood, composed of 90% water and 10% proteins (e.g. albumin, fibrinogen, globulins), electrolytes, hormones and waste products. The rest of the blood is made up of cellular entities. CELLULAR COMPONENTS OF BLOOD: ○ Red blood cells (RBCs) or erythrocytes (45% of blood volume) contain the protein, haemoglobin (Hb). Hb is responsible for binding and transporting oxygen from the lungs to the tissues, and returning carbon dioxide from the tissues to the lungs for exhalation. RBCs are produced in the bone marrow via erythropoiesis and typically have a lifespan of about 120 days. ○ White blood cells (WBCs) or leukocytes (less than 1% of blood volume) are responsible for the body’s immune response, and include neutrophils, lymphocytes, monocytes, eosinophils and basophils. ○ Platelets or thrombocytes (less than 1% of blood volume) are anucleate cells that support blood clotting through their interaction with clotting factors. THERE ARE THREE MAJOR TYPES OF HAEMATOPOIESIS: Erythropoiesis, through which, RBCs are synthesised. Myelopoiesis, through which, WBCs are synthesised. Thrombopoiesis or megakaryopoiesis, through which, platelets are synthesised. Universally speaking, haematopoiesis is regulated by Hematopoietic Growth Factors (e.g. erythropoietin and thrombopoietin) and cytokines that stimulate the proliferation and differentiation of specific blood cell lineages. These growth factors are produced primarily by stromal cells but also in organs such as the kidney and liver. Haematopoietic Growth Factors attach to cell receptors and trigger a phosphorylation cascade that activates transcription factors in the cell nucleus, subsequently modulating gene expression. FOR THIS UNIT, ERYTHROPOIESIS WILL BE THE FOCUS: Erythropoiesis takes place in the bone marrow and is regulated by erythropoietin (EPO), a renal hormone. When tissues experience hypoxia, Hypoxia-Inducible Factor (HIF) is stabilised, inducing EPO production and increasing the rate of erythropoiesis. When oxygen levels are high, EPO production decreases. Erythropoiesis requires: iron (for Hb production), vitamin B12 and folate (for DNA synthesis during RBC maturation) and copper and pyridoxine (vitamin B6) which are involved in the incorporation of iron into Hb. B12 deficiency or folate deficiency can lead to megaloblastic anaemia. Thyroid hormones have a stimulatory effect on erythropoiesis. Haemolysis is the process by which RBCs are broken down. ○ Haemolysis usually takes place after 120 days, with removal of old RBCs extravascularly by macrophages of the reticuloendothelial (RE) system. Haemolytic anaemia occurs when RBC destruction is not adequately compensated by an increase in erythropoiesis. This RBC destruction can be extravascularly or intravascularly-mediated (though intravascular haemolysis is almost always pathological). INSIDE THE RBC, THERE IS HAEMOGLOBIN: Haemoglobin is a protein in RBCs responsible for: oxygen transport from the lungs to tissues and carbon dioxide transport from the tissues back to the lungs. ○ Each Hb molecule consists of 4 globin chains (2 alpha chains and 2 beta-chains) and 4 haem groups (each containing iron in its ferrous state (Fe2+) which bind one oxygen atom each. To produce Hb, Heme synthesis and Globin chain synthesis must occur. Haem synthesis occurs in the RBC mitochondria through a series of reactions involving δ-aminolevulinic (ALA) synthase with vitamin B6 (pyridoxal phosphate) as a cofactor. Globin chain synthesis occurs in ribosomes. ○ The combination of haem and globin chains forms the functional Hb molecule. Haemoglobinopathies arise from production of structurally abnormal Hb or from synthesis of insufficient amounts of normal alpha-globin or beta-globin chains (alpha-thalassaemia or beta-thalassaemia). Alpha- and beta-thalassaemias are most common among people of Asian or Mediterranean descent and can range from carrier states to severe forms causing death in utero or chronic disease during childhood. ○ Poorly functioning globin chains lead to precipitation of unstable Hb and subsequently ineffective erythropoiesis. Thalassaemia assessment is very important in preconception and natal settings. HAEMOGLOBIN IS IMPORTANT BECAUSE IT CARRIES OXYGEN: Since Hb has four subunits, each Hb molecule can carry four oxygen molecules at full capacity. In the lungs, where oxygen concentration is high, oxygen molecules diffuse into the RBCs and bind to the iron ion (Fe2+) in each haem group. ○ The first oxygen molecule binds to one haem group, inducing a conformation changing in the Hb molecule. ○ This conformational change increases the affinity of the remaining Haem groups for oxygen, making it easier for the subsequent oxygen molecules to bind. This is called cooperative binding. In tissues where oxygen concentration is low, and carbon dioxide is higher, oxygen is released from Hb. This oxygen unloading in the tissues is influenced by the following factors: Partial pressure of oxygen (pO2): At high pO2 such as in the lungs, Hb is almost fully saturated; at lower pO2, such as in the tissues, oxygen is released. This ensures that Hb efficiently picks up oxygen in the lungs and releases it where needed in the tissues. 2,3-diphosphoglycerate (2,3-DPG): is a metabolite produced by RBCs that binds to Hb, and stabilises the deoxygenated form, lowering its affinity for oxygen. Increased 2,3-DPG levels (e.g. during hypoxia or exercise) help unload oxygen more efficiently in tissues. Temperature: Higher body temperatures, often seen during exercise, decrease Hb’s affinity for oxygen, promoting oxygen unloading. pH (Bohr effect): As tissues produce carbon dioxide, it combines with water to form carbonic acid which dissociates into hydrogen ions (H+) and bicarbonate. The increased H+ concentration lowers the pH, reducing Hb’s affinity for oxygen and promoting its release to the tissues. THE OXYGEN DISSOCIATION CURVE GRAPHICALLY REPRESENTS THE RELATIONSHIP BETWEEN THE PARTIAL PRESSURE OF OXYGEN (pO2) AND THE PERCENTAGE OF HAEMOGLOBIN SATURATION (% SATURATION): Key features of the oxygen-dissociation curve include: A sigmoidal shape which reflects the cooperative binding of oxygen to Hb. ○ As each molecule of oxygen binds to Hb, the affinity for the next oxygen molecule increases. This results in a rapid increase in Hb saturation as oxygen binds to the first few Haem groups. The x-axis which represents pO2 (in mmHg). In the lungs, pO2 is high. In the tissues, pO2 is lower. The y-axis which represents oxygen saturation of Hb (%) which indicates how much of the Hb is carrying oxygen. A left shift occurs when Hb has a higher affinity for oxygen, meaning it holds onto oxygen more tightly and is less likely to release it in tissues. ○ Recall that factors that cause a left shift include: decreased temperature, decreased 2,3-DPG, decreased [H+] and carbon monoxide (which binds to Hb with a higher affinity than oxygen, preventing oxygen release) A right shift occurs when Hb has a lower affinity for oxygen, meaning it releases oxygen more readily in the tissues. ○ Recall that factors that cause a right shift include: increased temperature, increased 2,3-DPG, increased [H+] and increased CO2. The P50 value refers to the partial pressure of oxygen at which Hb is 50% saturated with oxygen. ○ For normal adult Hb, the P50 is about 30 mmHg. In a left shift, P50 decreases, indicating higher affinity and need for less oxygen to achieve 50% saturation. In a right shift, P50 increases, indicating lower affinity and need for more oxygen to achieve 50% saturation. HOW RED BLOOD CELLS ARE ABLE TO FULFIL THEIR FUNCTIONS: RBCs are designed to perform a critical function in gas exchange, which involves transporting oxygen from the lungs to tissues and carrying carbon dioxide back to the lungs for exhalation. To do this effectively, RBCs must meet several conditions: ○ Travel through microcirculation: RBCs travel through very small blood vessels called capillaries, some of which have diameters as narrow as 3.5um. RBCs themselves are 7-8um in diameter but they are flexible enough to squeeze through the narrow vessels without breaking. This is possible due to their flexible biconcave disc shape. The biconcave shape increases their surface area for gas exchange and allows them to deform when moving through tight spaces. ○ Maintenance of Hb in the reduced (ferrous) state: Hb must be kept in the reduced ferrous state (Fe2+) to bind oxygen properly. If Hb is oxidised to Fe3+, Hb becomes methemoglobin, which cannot effectively transport oxygen. Therefore, RBCs rely on reducing agents such as NADH and NADPH to keep Hb in the right state for oxygen binding. ○ Osmotic equilibrium: Despite the high concentration of Hb inside the RBC, the RBC maintains osmotic balance with their surroundings. This is essential for preventing the cell from swelling and bursting or shrinking and becoming less functional. The structure of the RBC membrane and its active transport mechanisms help achieve this osmotic balance. ○ Energy production via anaerobic glycolysis: RBCs generate energy through anaerobic glycolysis, meaning they do not rely on oxygen to produce energy. This is important because using oxygen would interfere with their main job of transporting and delivering oxygen. ○ Generating “reducing power” via NADH and NADPH: To maintain Hb in a reduced state and protect the cell from oxidative damage, RBCs generate reducing agents such as NADH (from glycolysis) and NADPH (from the pentose phosphate pathway) to neutralise ROS and keep Hb in its Hb form, and not the methemoglobin form that cannot carry oxygen. RED CELL METABOLISM AND ASSOCIATED PATHOLOGY: Red cell metabolism involves two major pathways: the Embden-Meyerhof Pathway and the Hexose Monophosphate Shunt (pentose phosphate) Pathway. ○ The Embden-Meyerhof Pathway (a form of glycolysis): Is the primary metabolic route through which RBCs generate ATP, NADH and 2,3-DPG. ATP is needed for maintaining RBC membrane integrity and shape by powering ion pumps that control the intracellular osmolarity. Without ATP, the RBCs become rigid, leading to abnormalities like crenation (especially common in pyruvate kinase (PK)) deficiency). In PK deficiency, RBCs undergo haemolysis more quickly as they lose flexibility, exhibit structural deformities and possess increased sensitivity to oxidative stress, and are ultimately destroyed in the spleen. ○ The Hexose Monophosphate (pentose phosphate) Pathway: Generates NADPH, essential for protecting RBCs from oxidative damage by maintaining adequate levels of reduced glutathione. Glucose-6-phosphate dehydrogenase (G6PD) deficiency can affect this pathway, resulting in RBCs being highly sensitive to oxidative stress. This deficinecy can lead to blistering and breakdown of RBCs upon exposure to oxidant stress, such as certain medications or infections. THE ROLE OF THE MEMBRANE, METABOLIC AND STRUCTURAL PROTEINS IN RBC FUNCTION AND PATHOLOGY: The RBC membrane is composed of a lipid-bilayer and a membrane skeleton made up of proteins like spectrin and actin. These proteins are responsible for: Maintaining RBC shape and flexibility which is important for the RBCs to pass through narrow capillaries. Any defect in these structural proteins, such as in hereditary spherocytosis or elliptocytosis, can lead to RBC deformity. These cells are more easily destroyed by the spleen, resulting in haemolytic anaemia. Clinical manifestations of these disorders include jaundice, splenomegaly, fatigue, gallstones, pallor and cholecystitis due to increased RBC turnover. THE ROLE OF IRON IN HEALTHY RBCs: Iron is required for the formation of functional Hb. Iron requirements are variable depending on age and sex of the patient. Iron is absorbed in the duodenum and enters the bloodstream where it binds to transferrin (Tf) for transport. ○ Iron absorption is inhibited by calcium, tannins (in tea and coffee) and phytates (in cereals). ○ Iron absorption is enhanced by vitamin C (ascorbic acid), haem iron (from animal sources) and legume fermentation. Iron deficiency is the most common cause of anaemia. Anaemia is when the body does not have sufficient RBCs to carry adequate oxygen to the tissues. Iron is stored as ferritin or hemosiderin in the reticuloendothelial system (RES) liver, spleen, and bone marrow. Hepcidin (hepatic bactericidal protein) is a negative regulator of iron metabolism. Hepcidin is a hormone produced by the liver that causes internalisation and degradation of ferroportin (FPN). ○ FPN exports iron into the blood (present on the basolateral membrane of intestinal cells and hepatocytes, as well as the surface of macrophages). When there is inflammation, infection or high iron levels, hepcidin levels increase, leading to reduced iron availability When there is hypoxia, anaemia, ineffective erythropoiesis, decreased iron levels and increased EPO, hepcidin levels decrease, leading to increased iron availability in the blood. In states of chronic inflammation, IL-6 and IL-1 are released by immune cells, resulting in activation of the JAK/STAT pathway, including in hepatocytes. Activation of JAK/STAT in hepatocytes leads to upregulation of hepcidin production. By inhibiting FPN, the protein that transports iron from inside cells into the bloodstream, hepcidin effectively sequesters iron, preventing its release from storage sites and reducing its absorption. ○ Reduced absorption is because iron gets trapped inside enterocytes and eventually gets lost when these cells are shed into the digestive tract, preventing the iron from entering the body’s circulation. Similarly, iron stored in macrophages is locked inside and cannot be released into the bloodstream for use in RBC production or other functions. ○ While hepcidin’s effects are meant to help prevent bacterial infection, when hepcidin levels are high (as in chronic inflammation), iron absorption from the diet is reduced and iron recycling from macrophages is blocked, leading to anaemia of chronic inflammation. There is no active excretion pathway for iron. If a patient has been given too much iron, the will have iron overload that they cannot excrete, resulting in iron fixation in organs like the heart. Parameter Definition Iron Fraction of iron in the blood (NOT A GOOD INDICATOR) Transferrin Serum iron transporter molecule Transferrin Sat Ratio of total serum iron to transferrin Ferritin Iron storage protein (BETTER INDICATOR OF IRON STORAGE LEVELS) TIBC (total iron binding Measurement of the total iron concentration in the capacity) sample when fully saturated THE ROLE OF VITAMIN B12 (COBALAMIN) IN HEALTHY RBCs: Vitamin B12 is essential for DNA synthesis, specifically through its role in methionine and folate cycles. In these cycles, Vitamin B12 serves as a cofactor for the enzyme methionine synthase which is involved in the conversion of homocysteine to methionine. Vitamin B12 also helps regenerate tetrahydrofolate (THF) which is important for DNA synthesis in rapidly dividing cells such as RBCs. 2ug/day Vitamin B12 are required, though the requirement is higher in pregnant and lactating women, and lower in children. Vitamin B12 is primarily found in animal-based foods (e.g. meat, dairy, eggs). Individuals who follow strict vegetarian or vegan diets are at higher risk of deficiency. ○ Vitamin B12 absorption requires the presence of Intrinsic Factor (IF), a glycoprotein secreted by parietal cells of the stomach: Ingested Vitamin B12 binds to haptocorrin (HC) in the stomach. In the small intestine, pancreatic enzymes degrade haptocorrin, freeing B12 to bind to IF. The IF-B12 complex is absorbed in the distal ileum via specific receptors (e.g. cubam receptors). After transportation, Vitamin B12 is transported in the bloodstream by transcobalamin (TC) to various tissues, including the liver and bone marrow where it can be stored or utilised. Vitamin B12 is stored primarily in the liver, with reserves sufficient enough to last several years. Vitamin B12 is excreted via the desquamation of epithelial cells from the GI tract, and there is little active excretion through urine. VITAMIN B12 DEFICIENCY AND ITS IMPACT ON RBCs: Causes of Vitamin B12 deficiency include: ○ Pernicious anaemia: an autoimmune condition where the body produces antibodies against parietal cells or intrinsic factor, leading to impaired B12 absorption. ○ Malabsorption syndrome (e.g. Crohn's disease, gastric bypass). ○ Dietary deficiency The body stores large amounts of Vitamin B12 so deficiency symptoms may take years to manifest. Vitamin B12 deficiency can lead to megaloblastic anaemia, characterised by large, immature RBCs due to impaired DNA synthesis. ○ Neurological symptoms of megaloblastic anaemia include peripheral neuropathy and subacute combined degeneration of the spinal cord (affecting proprioception and vibration sensation). THE ROLE OF FOLATE IN HEALTHY RBCs: Folate has an important role in DNA synthesis and amino acid interconversions via single carbon unit transfers. ○ Folate helps convert deoxyuridine into thymidine, an important step in DNA synthesis that is essential for cell replication, particularly in the bone marrow. ○ Folate also participates in the methionine cycle by converting homocysteine to methionine through the methionine synthase + B12 cofactor reaction. 400ug/day of folate is required, though intake needs increase in pregnant and lactating women due to increased DNA synthesis. Folate is found in a variety of foods both plant-based (leafy greens, legumes, citrus fruits) and animal-based (liver and eggs). ○ Before absorption, dietary folate is converted to methyl-tetrahydrofolate (methyl THF). Methyl THF is the active form absorbed in the duodenum and jejunum. Once absorbed, folate is transported as methyl THF to tissues, especially the liver. Folate is stored primarily in the liver, but the body’s folate reserves are relatively small compared to Vitamin B12. This means that folate deficiency is more likely to develop rapidly during periods of insufficient intake. Folate is excreted through the skin and GI tract cell turnover, and small amounts of folate are lost in the urine. FOLATE DEFICIENCY AND ITS IMPACT ON RBCs: Causes of folate deficiency include: ○ Dietary insufficiency, particularly in populations with poor access to folate-rich foods. ○ Malabsorption syndromes, such as coeliac disease. ○ Increased folate demand, such as during pregnancy, lactation, and in conditions with chronic RBC turnover (e.g. haemolytic anaemia). ○ Alcoholism as alcohol interferes with folate metabolism and absorption. Folate deficiency can lead to megaloblastic anaemia, similar to Vitamin B12 deficiency, where the RBCs become large and immature. Folate is especially important during pregnancy to prevent neural tube defects (e.g. spina bifida) in the developing foetus, hence why folate supplementation is recommended during pregnancy. Note that there are important interactions between Vitamin B12 and folate: ○ Vitamin B12 and folate work together in the methionine-synthase reaction required to produce methionine, an important amino acid involved in DNA synthesis and therefore, the production of normal RBCs. In the methionine synthase reaction, homocysteine is converted to methionine with Vitamin B12 acting as a cofactor and folate, in its methyl-THF form, providing the methyl group that is transferred to homocysteine to convert it into methionine. Therefore, if either vitamin B12 or folate is deficient, the methionine-synthase reaction does not occur properly, leading to the accumulation of homocysteine in the blood. ○ Increased homocysteine levels are harmful because they can damage blood vessels, increasing the risk of conditions like heart attack and stroke. In Vitamin B12 deficiency, the methyl trap can occur. Folate, in its methyl-THF is trapped because it cannot transfer its methyl group to homocysteine to help convert it into methionine. Thus, folate cannot be used for any other important reactions. Even if folate levels are normal, folate becomes functionally deficient. Clinically speaking, the above is important because it is essential to check for Vitamin B12 deficiency before treating someone with folate supplements. Folate can correct megaloblastic anaemia but it will not fix the neurological problems caused by Vitamin B12 deficiency. ○ If you were to only give folate supplements to someone who is Vitamin B12 deficient, the neurological symptoms of Vitamin B12 deficiency (like nerve damage and cognitive issues) will continue to worsen, even though their RBC production may improve. ANAEMIA AS A CONDITION THAT HAEMATOLOGISTS FREQUENTLY TREAT: Anaemia is a condition in which there is a reduction in the Hb concentration below the normal range for age and sex. ○ When there are fewer RBCs or less Hb, the body’s tissues receive less oxygen. This can result in symptoms such as fatigue, weakness, pale skin, and shortness of breath. ○ Requires work-up to determine the cause (e.g. history, examination and investigation) ○ Treatment is guided by the aetiology. Anaemia can be classified based on the Mean Corpuscular Volume (MCV) which measures the average size of RBCs. This classification is the first step in identifying the cause of anaemia. Based on MCV, anaemias are classified into: ○ Microcytic anaemia (low MCV): RBCs are smaller than normal. Caused by: ○ Iron-deficiency anaemia (most common) ○ Thalassaemia (a genetic disorder affecting Hb) ○ Anaemia of chronic inflammation (where long-term illness affects RBC production) ○ Normocytic anaemia (normal MCV): RBCs are of normal size, but there are not enough of them. Caused by: ○ Acute blood loss (e.g. from trauma or surgery) ○ Anaemia of chronic disease (e.g. kidney disease or chronic infection) ○ Renal failure (i.e. where the kidneys cannot produce enough EPO) ○ Macrocytic anaemia (high MCV): RBCs are larger than normal. Caused by: ○ Vitamin B12 or folate deficiency. ○ Alcoholism (which can interfere with folate absorption and liver function) ○ Liver disease (which alters RBC production) SPECIFIC TYPES OF ANAEMIA: After using MCV to classify the anaemia broadly, anaemias are then classified based on their underlying cause. These classifications include: ○ Iron-deficiency anaemia (IDA): A specific type of microcytic anaemia caused by a lack of iron, a key component of functional haemoglobin. In early stages of IDA, patients may have normocytic RBCs. As IDA progresses, IDA patients may have microcytic and hypochromic RBCs. Haemolytic anaemia: Refers to the premature destruction of RBCs and can present in different MCV categories depending on stage and severity. Caused because RBCs are destroyed faster than the body can replace them (e.g. autoimmune disorders, mechanical damage, toxins, or hereditary conditions). ○ Early stages may have normocytic RBCs because the body tries to compensate by producing more RBCs. ○ As haemolysis continues, reticulocyte count increases (reticulocytes are larger than mature RBCs), which may give a macrocytic appearance on blood smears. Thus, haemolytic anaemia could fall under normocytic or even macrocytic anaemia, depending on the progression. Haemolytic anaemia may be via intravascular haemolysis where the RBCs are destroyed within the blood vessels, often due to: ○ Autoimmune diseases ○ Mechanical damage ○ Toxins Haemolytic anaemia may be via extravascular haemolysis where the RBCs are destroyed outside the blood vessels, typically in the spleen or liver, due to: ○ Hereditary conditions (e.g. hereditary spherocytosis) where the RBCs are abnormally shaped. ○ Acquired conditions (e.g. autoimmune haemolytic anaemia). When performing blood tests for patients with suspected haemolytic anaemia, the reticulocyte count (a measure of new RBC production) is often elevated because the bone marrow is attempting to compensate for lost RBCs. Autoimmune haemolytic anaemia (AIHA): ○ Involves the production of autoantibodies that cause the body to attack its own RBCs, causing them to be destroyed prematurely. Caused by: ○ Idiopathic reasons ○ Secondary to infection (after Epstein-Barr and other infections that confuse the immune system) ○ Secondary to haematological malignancy, immune disease or post-transfusion/transplant Various types of AIHA exist: ○ Warm autoimmune haemolytic anaemia (WAIHA) (IgG +/- complement) ○ Cold agglutinin disease (CAD) ○ Paroxysmal cold haemoglobinuria (PCH) ○ Mixed-type ○ Drug-induced Warm AIHA involves IgG antibodies which usually attack RBCs in the spleen. It typically occurs at normal body temperatures. Cold AIHA involves IgM antibodies which cause RBCs to be destroyed at colder temperatures, such as in the fingers and toes. ○ AIHA is diagnosed with the direct antiglobulin test (also called the Coombs test) which detects antibodies attached to the RBCs. HAEMOLYTIC MARKERS: To examine haemolysis, consider: ○ Blood films in which you inspect for spherocytes, schistocytes, oxidative change (bites and blister cells), agglutination and target cells ○ Bilirubin levels (Is bilirubin high? Is it bilirubin unconjugated?) ○ Haptoglobin (Is it exhausted?) ○ In conditions like haemolytic anaemia, where red blood cells are being destroyed prematurely and rapidly, LDH levels become elevated in the blood. When red blood cells are destroyed, the contents of the cells, including LDH, are released into the bloodstream. LDH elevation is one of the markers of intravascular haemolysis (destruction of red blood cells within the blood vessels) or extravascular haemolysis (destruction by macrophages in the spleen or liver). ○ Direct antiglobulin or Coombs test which considers whether there are antibodies binding to the RBCs. THE DIRECT AGGLUTINATION TEST (DAT) OR COOMB’S TEST: Used to detect autoantibodies or complement proteins that are bound to the surface of erythrocytes in conditions such as AIHA. ○ The red blood cells of a patient are shown coated with autoantibodies (immune system antibodies that mistakenly target the body’s own cells) or complement proteins (part of the immune system that enhances antibody-mediated responses). This occurs in vivo, meaning it happens within the patient’s body. A diagnostic antibody reagent is introduced. These reagents are designed to target the antibodies or complement proteins that are already attached to the patient’s red blood cells. The reagent could be: Anti-IgG antibodies (if the patient’s red cells are coated with IgG antibodies). Anti-complement antibodies (if complement proteins are attached to the red cells). ○ The diagnostic antibodies bind to the autoantibodies or complement on the red blood cells, causing the cells to clump together (called agglutination). This visible agglutination indicates a positive DAT result, meaning that the patient’s red blood cells are coated with antibodies or complement. FULL BLOOD EXAMINATION AND FILM PARAMETERS: A Full Blood Examination (FBE), also known as a Complete Blood Count (CBC), is a common blood test used to evaluate your overall health and detect a wide range of disorders, including anaemia, infection, and other diseases affecting blood cells. RBC indices: Hb, Hct, MCV, MCH, MCHC, RDW WBC count: WBC count, differential counts for neutrophils, lymphocytes, monocytes, eosinophils and basophils Platelet count: total platelet count Parameter Definition Hb (haemoglobin) Number of grams per litre of blood WBC (white blood cell) Number of WBC per litre of blood Platelets Number of platelets per litre of blood If low, may indicate risk of bleeding. If high, may indicate clotting disorders. Hct (haematocrit) Proportion of total blood volume that is comprise of RBC MCV (mean corpuscular volume) Measurement of the average RBC size, (Hct / RBC) x 10, allowing classification of anaemia as microcytic, normocytic or macrocytic MCH (mean cell haemoglobin) Calculation of the amount of Hb inside RBC, (Hb / RBC) x 10 MCHC (mean cell haemoglobin Calculation of the concentration of Hb inside RBC, (Hb / Hct) x 10 concentration) Average amount of haemoglobin inside an RBC RBC (red blood cell count) Number of RBC per litre of blood RDW (red cell distribution width) Calculation of the variation in RBC size. Higher RDW means more variability in size. Neutrophils Number of neutrophils per litre of blood Neutrophils fight bacterial infection Lymphocytes Number of lymphocytes per litre of blood Play a role in the adaptive response (B cells and T cells) Monocytes Number of monocytes per litre of blood Help break down bacteria and dead tissue Eosinophils Number of eosinophils per litre of blood Help fight parasitic infections and play a role in allergic reactions Basophils Number of basophils per litre of blood Involved in inflammatory reactions and allergic responses FOR YEAR 3B AND NOT ASSESSED IN YEAR A: WHITE BLOOD CELL DISORDERS: White blood cell (WBC) disorders are usually classified as either quantitative (abnormal numbers of WBCs) or qualitative (WBCs do not function properly). Leukocytosis (high WBC count): ○ An increase in WBCs, which can indicate: Infection Inflammation Cancer (like leukaemia, where there is an abnormal increase in WBCs). Leucopenia (low WBC count): ○ A decrease in WBCs, which can occur due to: Bone marrow failure (the bone marrow is not producing enough WBCs). Chemotherapy (which kills rapidly dividing cells, including WBCs). Autoimmune diseases (where the immune system attacks its own WBCs). LEUKAEMIA: Leukaemia is a type of cancer that affects white blood cells. It is classified into two main types based on the speed of progression: Acute leukaemia: Rapid onset and progression. Involves immature white blood cells (called blasts) that multiply rapidly but do not function properly. ○ Acute Myeloid Leukaemia (AML) ○ Acute Lymphoblastic Leukaemia (ALL) Chronic leukaemia: Slower progression. Involves more mature white blood cells that may function somewhat, but not as effectively as normal cells. ○ Chronic Myeloid Leukaemia (CML) ○ Chronic Lymphocytic Leukaemia (CLL)