Principles of MRI PDF
Document Details
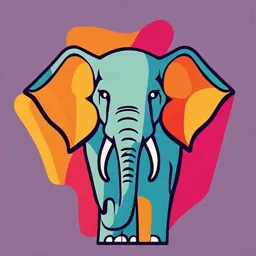
Uploaded by TopUnity
The Islamic University
Dr. Hayder Jasim Taher
Tags
Summary
This presentation details the principles of MRI, covering atomic structure, proton behavior in magnetic fields, magnetization, and image formation. It covers topics such as longitudinal and transverse magnetization, relaxation times (T1 and T2), and proton density (PD) images. The presentation is designed for students in postgraduate medical imaging programs.
Full Transcript
Principles of MRI Dr. Hayder Jasim Taher PhD of Medical Imaging Outline of my presentation Atomic structure. Motion of atom. Protons in MR imaging. Longitudinal Magnetization. Transverse Magnetization. MR Signal. Localization of the Signal. Basic four steps of MR imaging. Longitudinal Rel...
Principles of MRI Dr. Hayder Jasim Taher PhD of Medical Imaging Outline of my presentation Atomic structure. Motion of atom. Protons in MR imaging. Longitudinal Magnetization. Transverse Magnetization. MR Signal. Localization of the Signal. Basic four steps of MR imaging. Longitudinal Relaxation. Transverse Relaxation T1, T2, PD Weighted Image. Atomic structures Atomic structures: - All things are made of atoms. Atoms are organized into molecules, which are two or more atoms arranged together. The most abundant atom in the human body is hydrogen, but there are other elements such as oxygen, carbon, and nitrogen. Hydrogen is most commonly found in molecules of water (where two hydrogen atoms are arranged with one oxygen atom; H2 O) and fat (where hydrogen atoms are arranged with carbon and oxygen atoms; the number of each depends on the type of fat). The atom consists of a central nucleus and orbiting electrons, electrons are particles that spin around the nucleus. (Figure 1). The nucleus is very small, one millionth of a billionth of the total volume of an atom, but it contains all the atom’s mass. This mass comes mainly from particles called nucleons, which are subdivided into protons and neutrons. Protons have a positive electrical charge, neutrons have no net charge, and electrons are negatively charged. Motion of atom. Fig.1: The atom Protons in MR imaging Protons in MR imaging : - Protons are positively charged and have rotatory movement called spin. Any moving charge generates current. Every current has a small magnetic field around it. So, every spinning proton hasa small magnetic field around it, also called magnetic dipole moment. Normally the protons in human body (outside the magnetic field) move randomly in any direction. When external magnetic field is applied, i.e. patient is placed in the magnet, these randomly moving protons align (i.e. their magnetic moment align) and spin in the direction of external magnetic field. Some of them align parallel and others anti- parallel to the external magnetic field. When a proton aligns along external magnetic field, not only it rotates around itself (called spin) but also its axis of rotation moves forming a ‘cone’. This movement of the axis of rotation ofa proton is called as precession (Fig. 2). Protons in MR imaging The number of precessions of a proton per second is called precession frequency. It is measured in Hertz. Precession frequency is directly proportional to strength of external magnetic field. Stronger the external magnetic field, higher is the precession frequency. This relationship is expressed by Larmor’s equation— Wo = γ Bo Where wo = Precession frequency in Hz Bo = Strength of external magnetic field in Tesla γ = Gyromagnetic ratio, which is specific to Fig.2: Spin versus precession particular nucleus. Longitudinal Magnetization Longitudinal Magnetization: - Let us go one step further and understand what happens when protons align under the influence of external magnetic field. For the orientation in space consider X, Y, and Z axes system. External magnetic field is directed along the Z-axis. Conventionally, the Z-axis is the long axis of the patient as well as bore of the magnet. Protons align parallel and antiparallel to external magnetic field, i.e. along positive and negative sides of the Z-axis. Forces of protons on negative and positive sides cancel each other. However, there are always more protons spinning on the positive side or parallel to Z-axis than negative side. So, after canceling each other’s forces there are a few protons on positive side that retain their forces. Forces of these protons add up together to form a magnetic vector along the Z-axis. This is called as longitudinal magnetization (Fig. 3). Longitudinal magnetization thus formed along the external magnetic field cannot be measured directly. For the measurement it has to be transverse. Longitudinal Magnetization Fig. 3: Longitudinal magnetization Transverse Magnetization Transverse Magnetization:- when patient is placed in the magnet , longitudinal magnetization is formed along the Z-axis. The next step is to send radiofrequency (RF) pulse. The precessing protons pick up some energy from the radiofrequency pulse. Some of these protons go to higher energy level and start precessing antiparallel (along negative side of the Z-axis). The imbalance results in tilting of the magnetization into the transverse (X-Y) plane. This is called as transverse magnetization (Fig.4). In short, RF pulse causes titling of the magnetization into transverse plane. The precession frequency of protons should be same as RF pulse frequency for the exchange of energy to occur between protons and RF pulse. When RF pulse and protons have the same frequency protons can pick up some energy from the RF pulse. This phenomenon is called as “resonance”- the R of MRI. RF pulse not only causes protons to go tohigher energy level but also makes them precess Transverse Magnetization Fig. 4: Transverse magnetization. Magnetization vector is flipped in transverse plane by the 90-degree RF pulse. MR Signal MR Signal: - Transverse magnetization vector has a precession frequency. It constantly rotates at Larmor frequency in the transverse plane and induces electric current while doing so. The receiver RF coil receives this current as MR signal (Fig. 5). The strength of the signal is proportional to the magnitude of the transverse magnetization. MR signals are transformed into MR image by computers using mathematical methods such as Fourier Transformation. MR Signal Fig. 5: MR signal. Localization of the Signal Localization of the Signal: - Three more magnetic fields are superimposed on the main magnetic field along X, Y, and Z axes to localize from where in the body signals are coming. These magnetic fields have different strength in varying location hence these fields are called “gradient fields” or simply “gradients”. The gradient fields are produced by coils called as gradient coils. The three gradients are— 1.Slice selection gradient 2.Phase encoding gradient Slice Selection Gradient -Slice Selection Gradient: - Slice selection gradient has gradually increased magnetic field strength from one end to another. It determines the slice position. Slice thickness is determined by the bandwidth of RF pulse. Bandwidth is the range of frequencies. Wider the bandwidth thicker is the slice. -Phase Encoding and Frequency Encoding Gradients These gradients are used to localize the point in a slice from where signal is coming. They are applied perpendicular to each other and perpendicular to the slice selection gradient (Fig. 6). Slice Selection Gradient 1. Z-axis—Slice selection gradient 2. Y-axis—Frequency encoding gradient 3.X-axis—Phase encoding Fig. 6: Frequency and phase encoding gradients gradient. Slice Selection Gradient In a usual sequence, slice selection gradient is turned on at the time of RF pulse. Phase encoding gradient is turned on for a short time after slice selection gradient. Frequency encoding or readout gradient is on in the end at the time of signal reception. Information from all three axes is sent to computers to get the particular point in that slice from which the signal is coming. Basic four steps of MR imaging 1. Patient is placed in the magnet— All randomly moving protons in patent’s body align and precess along the external magnetic field. Longitudinal magnetization is formed along the Z-axis. 2. RF pulse is sent— Precessing protons pick up energy from RF pulse to go to higher energy level and precess in phase with each other. This results in reduction in longitudinal magnetization and formation of transverse magnetization in X-Y plane. 3. MR signal is received— The transverse magnetization vector precesses in transverse plane and generates current. This current is received as signal by the RF coil. 4. Image formation—MR signal received by the coil is transformed into image by complex mathematical process such as Fourier Transformation by computers. T1, T2 Relaxations and Image Weighting T1, T2 Relaxations and Image Weighting: Relaxation means recovery of protons back towards equilibrium after been disturbed by RF excitation. Relaxation times of protons such as T1 and T2, and number of protons in tissues (proton density) are the main determinant of the contrast in an MR image. What happens when RF pulse is switched off? When RF pulse is switched off, LM starts increasing along Z-axis and TM starts reducing in the transverse plane. The process of recovery of LM is called Longitudinal Relaxation while reduction in the magnitude of Longitudinal Relaxation Longitudinal Relaxation: When RF pulse is switched off, spinning protons start losing their energy. The low energy protons tend to alignalong the Z-axis. As more and more protons align along the positive side of the Z-axis there is gradual increase in the magnitude (recovery) of the LM (Fig. 7). The energy released by the protons is transferred to the surrounding (the crystalline lattice of molecules) hence the longitudinal relaxation is also called as ‘spin-lattice’ relaxation. The time taken by LM to recover to its original value after RF pulse is switched off is called longitudinal relaxation time or T1 which is the time when LM reaches back to 63% of its original value. Fig. 7: Longitudinal relaxation (T1) Transverse Relaxation Transverse Relaxation: The transverse magnetization represents composition of magnetic forces of protons precessing at similar frequency. More the number of protons precessing at the same frequency(in-phase) stronger will be the TM. So, right after the 90-deg RF pulse the net magnetization vector (now called transverse magnetization) is rotating in the X-Y plane around the Z-axis at the Larmor frequency. The refore, the vectors all points in the same direction because they are in-phase. However, they don’t stay like this. The transverse magnetization starts decreasing in magnitude immediately as protons start going out of phase. This process of de- phasing and reduction in the amount of transverse magnetization is called transverse relaxation. The time taken by TM to reduce to its original value is transverse relaxation time or T2 which is the time Transverse Relaxation Fig. 8: Transverse relaxation T2* (T2 star) T2* (T2 star): In addition to the magnetic field inhomogeneity intrinsic to the tissues causing spin-spin relaxation, inhomogeneity of the external magnetic field (B0) also causes decay of the TM. Decay of the TM caused by combination of spin-spin relaxation and inhomogeneity of external magnetic field is called T2* relaxation. Dephasing effects of external magnetic field inhomogeneity are eliminated by 180° RF pulses used in spin-echo sequence. Hence there is ‘true’ T2 relaxation in a spin-echo sequence. The T2* relaxation is seen in gradient-echo sequence as there is no 180° RF pulse in gradient-echo sequence. T2* is shorter than T2(Fig. 9). T2* (T2 star) Fig. 9: T2* curve TR and TE TR and TE: - - TR (Time to Repeat) is the time interval between start of one RF pulse and start of the next RF pulse. For a spin-echo sequence time interval between beginnings of 90-degree pulses is the TR. - TE (Time to Echo) is the time interval between start of RF pulse and reception of the signal (echo). (fig.10) TI: TI is Time of Inversion. It is the time between inverting 180- degree pulse and 90-degree pulse in Inversion Recovery (IR) sequence. TI determines the image contrast in IR sequences. Fig. 10: Spin Echo (SE) sequence. T1 Weighted Image T1 Weighted Image: The magnitude of LM indirectly determines the strength of MR signal. Tilting of stronger LM by 90-degree RF pulse will result into greater magnitude of TM and stronger MR signal. The tissues with short T1 regain their maximum LM in short-time after RF pulse is switched off. When the next RF pulse is sent, TM will be stronger, and resultant signal will also be stronger. Therefore, material with short T1 have bright signal on T1 weighted images. T1 Weighted Image Figs 11.A and B: T1- weighted image. (A) At short TR the difference between LM of tissue A (with short T1) and of tissue B (long T1) is more as compared to long TR. This results in more difference in signal intensity (contrast) between A and B at short TR. The contrast on the short TR image is because of T1 differences of tissues. Hence it is T1- weighted image. (B) T1- T2 Weighted Image T2 Weighted Image: Immediately after its formation TM has greatest magnitude and produces strongest signal. Thereafter it starts decreasing in magnitude because of dephasing, gradually reducing the intensity of received signal. Different tissues, depending on their T2, have variable time for which TM will remain strong enough to induce useful signal in the receiver coil. Tissues or material with longer T2, such as water, will retain their signal for longer time. Tissues with short T2 will lose their signal earlier after RF pulse is turned off. How does one make images T2 weighted? The images are made T2-wighted by keeping the TE longer. At short TE, tissues with long as well as short T2 have strong signal. Therefore, on the images acquired at short echo time, there will not be significant signal intensity difference between tissues with short and long T2. At longer TE, only those tissues with long T2 will have sufficiently strong signal and the signal difference between tissues with short and long T2 will be pronounced (Fig.12). So, the image with long TE is T2- weighted since the signal difference amongst tissues (contrast) is determined T2 Weighted Image Figs 12.A and B: T2-weighted image. (A) Tissue B has short T2 that results into early loss of magnitude of TM and reduction in signal. At short TE, there is no significant difference between magnitude of TM of A and B. At long TE, signal difference between A and B is more because tissue B will lose most of its signal while tissue A will still have good signal. Since the image contrast is because of Proton Density (PD) Image Proton Density (PD) Image: Contrast in the PD image is determined by the density of protons in the tissue. T1 effect is reduced by keeping long TR and T2 effect is reduced by keeping TE short. Hence long TR and short TE give PD- weighted image (Fig. 13). The signal intensity difference amongst tissues is function of the number of protons they have. Fig. 13: Proton-density image. Long TR used for PD image eliminates T1 effects and short TE eliminates T2 effects. Thank