Guyton Chapter 31: Acid-Base Regulation PDF
Document Details
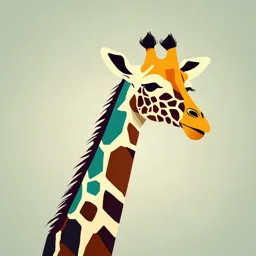
Uploaded by VerifiablePrologue3527
Tags
Summary
This chapter from Guyton's textbook delves into the mechanisms of acid-base regulation in the human body. It covers the roles of kidneys, lungs, and buffering systems in maintaining a stable hydrogen ion concentration. The chapter also explains the definitions of acids, bases, and pH.
Full Transcript
CHAPTER 31 UNIT V Acid–Base Regulation The regulation of hydrogen ion (H+) balance is simila...
CHAPTER 31 UNIT V Acid–Base Regulation The regulation of hydrogen ion (H+) balance is similar in to as acids. An example is hydrochloric acid (HCl), which some ways to the regulation of other ions in the body. For ionizes in water to form hydrogen ions (H+) and chloride example, there must be a balance between the intake or ions (Cl−). Likewise, carbonic acid (H2CO3) ionizes in production of H+ and the net removal of H+ from the body water to form H+ and bicarbonate ions (HCO3−). to achieve homeostasis. And, as is true for other ions, the A base is an ion or a molecule that can accept an kidneys play a key role in regulating H+ removal from the H+. For example, HCO3− is a base because it can com- body. However, precise control of extracellular fluid H+ bine with H+ to form H2CO3. Likewise, HPO4= is a base concentration involves much more than the simple elimi- because it can accept an H+ to form H2PO4−. The pro- nation of H+ by the kidneys. Multiple acid–base buffer- teins in the body also function as bases because some of ing mechanisms involving the blood, cells, and lungs also the amino acids that make up proteins have net negative are essential in maintaining normal H+ concentrations in charges that readily accept H+. The protein hemoglobin extracellular and intracellular fluids. in the red blood cells and proteins in the other cells of In this chapter, we consider the mechanisms that regu- the body are among the most important of the body’s late H+ concentration, with special emphasis on renal H+ bases. secretion and renal reabsorption, production, and excre- The terms base and alkali are often used synony- tion of bicarbonate ions (HCO3−), one of the key compo- mously. An alkali is a molecule formed by the combi- nents of acid–base control systems in the body fluids. nation of one or more of the alkaline metals—such as sodium, potassium, and lithium—with a highly basic ion such as a hydroxyl ion (OH−). The base portion of these HYDROGEN ION CONCENTRATION IS molecules reacts quickly with H+ to remove it from solu- PRECISELY REGULATED tion and are, therefore, typical bases. For similar reasons, Precise H+ regulation is essential because the activities of the term alkalosis refers to the excess removal of H+ from almost all enzyme systems in the body are influenced by the body fluids, in contrast to the excess addition of H+, H+ concentration. Therefore, changes in H+ concentra- which is referred to as acidosis. tion alter virtually all cell and body functions. Compared with other ions, the H+ concentration Strong and Weak Acids and Bases. A strong acid, such of the body fluids normally is kept at a low level. For as HCl, rapidly dissociates and releases especially large example, the concentration of sodium in extracellular amounts of H+ in solution. Weak acids such as H2CO3 are fluid (142 mEq/L) is about 3.5 million times as great as less likely to dissociate their ions and, therefore, release the normal concentration of H+, which averages only H+ with less vigor. A strong base is one that reacts rap- 0.00004 mEq/L. Equally important, the normal variation idly and strongly with H+ and, therefore, quickly removes in H+ concentration in extracellular fluid is only about H+ from a solution. A typical example is OH−, which re- one millionth as great as the normal variation in sodium acts with H+ to form water (H2O). A typical weak base ion (Na+) concentration. Thus, the precision with which is HCO3− because it binds with H+ much more weakly H+ is regulated emphasizes its importance to the various than OH−. Most acids and bases in the extracellular fluid cell functions.! that are involved in normal acid–base regulation are weak acids and bases. The most important ones that we discuss are carbonic acid (H2CO3) and HCO3− base.! ACIDS AND BASES—DEFINITIONS AND MEANINGS Normal H+ Concentration and pH of Body Fluids and A hydrogen ion is a single free proton released from a Changes That Occur in Acidosis and Alkalosis. The hydrogen atom. Molecules containing hydrogen atoms blood H+ concentration is normally maintained with- that can release hydrogen ions in a solution are referred in tight limits around a normal value of about 0.00004 403 UNIT V The Body Fluids and Kidneys mEq/L (40 nEq/L). Normal variations are only about 3 to An extreme example of an acidic body fluid is the HCl 5 nEq/L but, under extreme conditions, the H+ concen- secreted into the stomach by the oxyntic (parietal) cells tration can vary from as low as 10 nEq/L to as high as 160 of the stomach mucosa, as discussed in Chapter 65. The nEq/L without resulting in death. H+ concentration in these cells is about 4 million times Because H+ concentration normally is low, and because greater than the hydrogen concentration in blood, with a these small numbers are cumbersome, it is customary to pH of 0.8. In the remainder of this chapter, we discuss the express H+ concentration on a logarithm scale using pH regulation of extracellular fluid H+ concentration.! units. pH is related to the actual H+ concentration by the following formula (H+ concentration [H+] is expressed in DEFENDING AGAINST CHANGES IN H+ equivalents per liter): CONCENTRATION: BUFFERS, LUNGS, pH = log 1 = − log H + AND KIDNEYS H+ Three primary systems regulate the H+ concentration in For example, normal [H+] is 40 nEq/L (0.00000004 the body fluids: (1) the chemical acid–base buffer systems Eq/L). Therefore, the normal pH is as follows: of the body fluids, which immediately combine with an acid or a base to prevent excessive changes in H+ con- pH = − log[0.00000004] centration; (2) the respiratory center, which regulates the pH = 7.4 removal of CO2 (and, therefore, H2CO3) from the extra- From this formula, one can see that pH is inversely cellular fluid; and (3) the kidneys, which can excrete acidic related to the H+ concentration; therefore, a low pH cor- or alkaline urine, thereby readjusting the extracellular responds to a high H+ concentration, and a high pH cor- fluid H+ concentration toward normal during acidosis or responds to a low H+ concentration. alkalosis. The normal pH of arterial blood is 7.4, whereas the When there is a change in H+ concentration, the buffer pH of venous blood and interstitial fluids is about 7.35 systems of the body fluids react within seconds to mini- because of the extra amounts of carbon dioxide (CO2) mize these changes. Buffer systems do not eliminate H+ released from the tissues to form H2CO3 in these fluids from or add H+ to the body but only keep them tied up (Table 31-1). Because the normal pH of arterial blood is until balance can be re-established. 7.4, a person is considered to have acidemia when the pH The second line of defense, the respiratory system, acts falls significantly below this value and alkalemia when the within a few minutes to eliminate CO2 and, therefore, pH rises above 7.4. The lower limit of pH at which a per- H2CO3 from the body. son can live more than a few hours is about 6.8, and the These first two lines of defense keep the H+ concen- upper limit is about 8.0. tration from changing too much until the more slowly Intracellular pH usually is slightly lower than plasma responding third line of defense, the kidneys, can elimi- pH because cell metabolism produces acid, especially nate the excess acid or base from the body. Although the H2CO3. Depending on the type of cells, the pH of intracel- kidneys are relatively slow to respond compared with the lular fluid has been estimated to range between 6.0 and 7.4. other defenses, over a period of hours to several days, they Hypoxia of the tissues and poor blood flow to the tissues are by far the most powerful of the acid–base regulatory can cause acid accumulation and decreased intracellular systems.! pH. The terms acidosis and alkalosis describe the pro- cesses that lead to acidemia and alkalemia, respectively. BUFFERING OF H+ IN THE BODY FLUIDS The pH of urine can range from 4.5 to 8.0, depend- ing on the acid–base status of the extracellular fluid. As A buffer is any substance that can reversibly bind H+. The discussed later, the kidneys play a major role in correcting general form of the buffering reaction is as follows: abnormalities of extracellular fluid H+ concentration by ⎯⎯ Buffer + H+ ← ⎯ → H Buffer ⎯ excreting acids or bases at variable rates. In this example, a free H+ combines with the buffer to Table 31-1 pH and H+ Concentration of Body Fluids form a weak acid (H buffer) that can either remain as an unassociated molecule or dissociate back to the buffer and H+ Concentration (mEq/L) pH H+. When the H+ concentration increases, the reaction is Extracellular Fluid forced to the right, and more H+ binds to the buffer, as Arterial lood −5 long as buffer is available. Conversely, when the H+ con- eno s lood −5 centration decreases, the reaction shifts toward the left, nterstitial id −5 and H+ is released from the buffer. In this way, changes in ntra ell lar id −3 to −5 H+ concentration are minimized. −2 −5 The importance of the body fluid buffers can be Urine to quickly realized if one considers the low concentration Gastric HCl of H+ in the body fluids and the relatively large amounts 404 Chapter 31 Acid–Base Regulation of acids produced by the body each day. About 80 mil- HCO3− to form the very weak acid H2CO3, which in turn liequivalents of H+ is ingested or produced each day by forms CO2 and H2O. The excess CO2 greatly stimulates metabolism, whereas the H+ concentration of the body respiration, which eliminates the CO2 from the extracel- fluids normally is only about 0.00004 mEq/L. With- lular fluid. out buffering, the daily production and ingestion of The opposite reactions take place when a strong base, acids would cause lethal changes in the body fluid H+ such as sodium hydroxide (NaOH), is added to the bicar- UNIT V concentration. bonate buffer solution. The action of acid–base buffers can perhaps best be NaOH + H2 CO3 → NaHCO3 + H2 O explained by considering the buffer system that is quanti- tatively the most important in the extracellular fluid—the In this case, the OH− from the NaOH combines with bicarbonate buffer system.! H2CO3 to form additional HCO3−. Thus, the weak base NaHCO3 replaces the strong base NaOH. At the same time, the concentration of H2CO3 decreases (because it BICARBONATE BUFFER SYSTEM reacts with NaOH), causing more CO2 to combine with The bicarbonate buffer system consists of a water solution H2O to replace the H2CO3: that contains two ingredients: (1) a weak acid, H2CO3; and (2) a bicarbonate salt, such as sodium bicarbonate CO2 + H2O ⎯⎯ → H2CO3 ⎯⎯ → ↑ HCO 3− + H+ (NaHCO3). + + H2CO3 is formed in the body by the reaction of CO2 NaOH Na with H2O: Carbonic CO2 + H2O ← anhydrase ⎯⎯⎯⎯ ⎯⎯⎯⎯ ⎯ → H2CO3 The net result, therefore, is a tendency for the CO2 levels in the blood to decrease, but the decreased CO2 in This reaction is slow, and exceedingly small amounts the blood inhibits respiration and decreases the rate of of H2CO3 are formed unless the enzyme carbonic anhy- CO2 expiration. The rise in blood HCO3− concentration drase is present. This enzyme is especially abundant in that occurs is compensated for by increased renal excre- the walls of the lung alveoli, where CO2 is released; car- tion of HCO3−. bonic anhydrase is also present in the epithelial cells of the renal tubules, where CO2 reacts with H2O to form Quantitative Dynamics of the Bicarbonate Buffer System H2CO3. All acids, including H2CO3, are ionized to some extent. H2CO3 ionizes weakly to form small amounts of H+ From mass balance considerations, the concentrations of and HCO3−: H+ and HCO3− are proportional to the concentration of H2CO3 ←⎯ → ⎯⎯ H + HCO3 + − H2CO3: H2CO3 ←⎯ → ⎯⎯ H + HCO3 + − The second component of the system, bicarbonate salt, occurs predominantly as NaHCO3 in the extracellular For any acid, the concentration of the acid relative to its fluid. NaHCO3 ionizes almost completely to form HCO3− dissociated ions is defined by the dissociation constant, K′. and Na+, as follows: H+ × HCO3− K′ = NaHCO3 ⎯ ←⎯ ⎯→ Na + HCO3 + − H2CO3 Now, putting the entire system together, we have the This equation indicates that in an H2CO3 solution, the following: amount of free H+ is equal to H2CO3 ⎯⎯ CO2 + H2O ← ⎯ → H2CO3 ←⎯ → H+ = K′ × ⎯ H + HCO3 + − ⎯ ⎯ HCO3− + Na+ The concentration of undissociated H2CO3 cannot be measured in solution because it rapidly dissociates Because of the weak dissociation of H2CO3, the H+ into CO2 and H2O or into H+ and HCO3−. However, the concentration is extremely low. CO2 dissolved in the blood is directly proportional to the When a strong acid such as HCl is added to the bicar- amount of undissociated H2CO3. Therefore, Equation 2 can bonate buffer solution, the increased H+ released from the be rewritten as follows: acid (HCl → H+ + Cl−) is buffered by HCO3−: CO2 H+ = K × ↑ H+ + HCO3− → H2CO3 → CO2 + H2O HCO3− The dissociation constant (K) for Equation 3 is only As a result, more H2CO3 is formed, causing increased about 1/400 of the dissociation constant (K′) of Equation 2 CO2 and H2O production. From these reactions, one because the proportionality ratio between H2CO3 and CO2 can see that H+ from the strong acid HCl reacts with is 1:400. 405 UNIT V The Body Fluids and Kidneys Equation 3 is written in terms of the total amount of kidneys, whereas the P2 in extracellular fluid is con- CO2 dissolved in solution. However, most clinical labo- trolled by the rate of respiration. By increasing the rate ratories measure the blood CO2 tension (P%2) rather of respiration, the lungs remove CO2 from the plasma than the actual amount of CO2. Fortunately, the amount and, by decreasing respiration, the lungs elevate P%2. of CO2 in the blood is a linear function of P%2 multi- Normal physiological acid–base homeostasis results plied by the solubility coefficient for CO2; under physi- from the coordinated efforts of both the lungs and kid- ological conditions, the solubility coefficient for CO2 is neys, and acid–base disorders occur when one or both 0.03 mmol/mm Hg at body temperature. This means of these control mechanisms are impaired, thus altering that 0.03 millimole of H2CO3 is present in the blood for either the HCO3− concentration or P%2 of extracellular each mm Hg P%2 measured. Therefore, Equation 3 can fluid. be rewritten as When disturbances of acid–base balance result from a primary change in extracellular fluid HCO3− concentra- (0.03 × Pco2 ) tion, they are referred to as metabolic acid–base disorders. H+ = K × HCO3− Therefore, acidosis caused by a primary decrease in HCO3− concentration is termed metabolic acidosis, whereas alka- Henderson-Hasselbalch Equation. As discussed earlier, losis caused by a primary increase in HCO3− concentra- it is customary to express the H+ concentration in pH units tion is called metabolic alkalosis. Acidosis caused by an rather than in actual concentrations. Recall that pH = −log H+. increase in P%2 is called respiratory acidosis, whereas The dissociation constant (pK) can be expressed in a alkalosis caused by a decrease in P%2 is termed respira- similar manner. tory alkalosis.! Bicarbonate Buffer System Titration Curve. Figure pK = −log K 31-1 shows the changes in pH of the extracellular fluid Therefore, we can express the H+ concentration in when the ratio of HCO3− to CO2 in extracellular fluid is al- Equation 4 in pH units by taking the negative logarithm of tered. When the concentrations of these two components that equation, which yields the following: are equal, the right-hand portion of Equation 8 becomes the log of 1, which is equal to 0. Therefore, when the two ( 0.03 × Pco2 ) − log H + = − log pK − log components of the buffer system are equal, the pH of the HCO3− solution is the same as the pK (6.1) of the bicarbonate buffer system. When base is added to the system, part of the dissolved CO2 is converted into HCO3−, causing an Therefore, increase in the ratio of HCO3− to CO2 and increasing the (0.03× Pco2 ) pH, as is evident from the Henderson-Hasselbalch equa- pH = pK− log tion. When acid is added, it is buffered by HCO3−, which HCO − 3 is then converted into dissolved CO2, decreasing the ratio of HCO3− to CO2 and decreasing the pH of the extracel- Rather than work with a negative logarithm, we can lular fluid.! change the sign of the logarithm and invert the numerator Buffer Power Determined by Amount and Relative and denominator in the last term, using the law of loga- Concentrations of Buffer Components. From the titration rithms, to yield the following: curve in Figure 31-1, several points are apparent. First, HCO3− the pH of the system is the same as the pK when each pH = pK+ log (0.03 × Pco2 ) of the components (HCO3− and CO2) constitutes 50% of the total concentration of the buffer system. Second, For the bicarbonate buffer system, the pK is 6.1, and the buffer system is most effective in the central part of Equation 7 can be written as follows: HCO3− 0 100 pH = 6.1 + log Normal 0.03 × Pco2 operating Percent of buffer in form of Percent of buffer in form of point in body Equation 8 is the Henderson-Hasselbalch equation and, 25 75 H2CO3 or CO2 with it, one can calculate the pH of a solution if the molar Base added Acid added concentration of HCO3− and the P%2 are known. HCO3– From the Henderson-Hasselbalch equation, it is appar- 50 50 pK ent that an increase in HCO3− concentration causes the pH to rise, shifting the acid–base balance toward alkalosis. An 75 25 increase in P%2 causes the pH to decrease, shifting the acid–base balance toward acidosis. The Henderson-Hasselbalch equation, in addition to 100 0 defining the determinants of normal pH regulation and 4 5 6 7 8 acid–base balance in the extracellular fluid, provides in- pH sight into the physiological control of the acid and base Figure 31-1. Titration curve for bicarbonate buffer system showing composition of the extracellular fluid. As discussed lat- the pH of extracellular fluid when the percentages of buffer in the er, the HCO3− concentration is regulated mainly by the form of HCO3− and CO2 (or H2CO3) are altered. 406 Chapter 31 Acid–Base Regulation the curve, where the pH is near the pK of the system. The phosphate buffer system has a pK of 6.8, which This phenomenon means that the change in pH for any is not far from the normal pH of 7.4 in the body fluids, given amount of acid or base added to the system is least allows the system to operate near its maximum buffer- when the pH is near the pK of the system. The buffer sys- ing power. However, its concentration in the extracellular tem is still reasonably effective for 1.0 pH unit on either fluid is low, at only about 8% of the concentration of the side of the pK, which, for the bicarbonate buffer system, bicarbonate buffer. Therefore, the total buffering power of UNIT V extends from a pH of about 5.1 to 7.1 units. Beyond these limits, the buffering power rapidly diminishes. And, when the phosphate system in the extracellular fluid is much all the CO2 has been converted into HCO3−, or when all less than that of the bicarbonate buffering system. the HCO3− has been converted into CO2, the system has In contrast to its minor role as an extracellular buffer, no more buffering power. the phosphate buffer is especially important in the tubu- The absolute concentration of the buffers is also an lar fluids of the kidneys for two reasons: (1) phosphate important factor in determining the buffer power of a sys- usually becomes greatly concentrated in the tubules, tem. With low concentrations of the buffers, only a small thereby increasing the buffering power of the phosphate amount of acid or base added to the solution changes the system; and (2) the tubular fluid usually has a consider- pH considerably.! ably lower pH than the extracellular fluid, bringing the Bicarbonate Buffer System Is the Most Important operating range of the buffer closer to the pK (6.8) of the Extracellular Buffer. From the titration curve shown in system. Figure 31-1, one would not expect the bicarbonate buffer The phosphate buffer system is also important in system to be powerful, for two reasons. First, the pH of the extracellular fluid is about 7.4, whereas the pK of the bicar- buffering intracellular fluid because the concentration bonate buffer system is 6.1, which means that there is about of phosphate in this fluid is many times higher than in 20 times as much of the bicarbonate buffer system in the the extracellular fluid. Also, the pH of intracellular fluid form of HCO3− as in the form of dissolved CO2. For this is lower than that of extracellular fluid and, therefore, is reason, this system operates on the portion of the buffering usually closer to the pK of the phosphate buffer system curve where the slope is low, and the buffering power is compared with the extracellular fluid.! poor. Second, the concentrations of the two elements of the bicarbonate system, CO2 and HCO3−, are not high. Despite these characteristics, the bicarbonate buffer PROTEINS ARE IMPORTANT system is the most powerful extracellular buffer in the INTRACELLULAR BUFFERS body. This apparent paradox is due mainly to the fact that Proteins are among the most plentiful buffers in the body the two elements of the buffer system, HCO3− and CO2, are regulated, respectively, by the kidneys and lungs, as dis- because of their high concentrations, especially in the cussed later. As a result of this regulation, the pH of the cells. The pH of the cells, although slightly lower than extracellular fluid can be precisely controlled by the relative in the extracellular fluid, nevertheless changes approxi- rate of removal and addition of HCO3− by the kidneys and mately in proportion to extracellular fluid pH changes. rate of removal of CO2 by the lungs.! There is a slight diffusion of H+ and HCO3− through the cell membrane, although these ions require several hours to reach equilibrium with the extracellular fluid, PHOSPHATE BUFFER SYSTEM except for the rapid equilibrium that occurs in the red Although the phosphate buffer system is not a major blood cells. CO2, however, can rapidly diffuse through extracellular fluid buffer, it plays an important role in buff- all the cell membranes. This diffusion of the elements of ering renal tubular fluid and intracellular fluids. the bicarbonate buffer system causes the pH in intracel- The main elements of the phosphate buffer system are lular fluid to change when there are changes in extra- H2PO4− and HPO4=. When a strong acid such as HCl is cellular pH. For this reason, the buffer systems in the added to a mixture of these two substances, the hydrogen cells help prevent changes in the pH of the extracellular is accepted by the base HPO4= and converted to H2PO4−: fluid but may take several hours to become maximally effective. HCl + Na2HPO4 → NaH2PO4 + NaCl In the red blood cell, hemoglobin (Hb) is an important buffer, as follows: The result of this reaction is that the strong acid, HCl, ⎯⎯ H+ + Hb ← ⎯ → HHb ⎯ is replaced by an additional amount of a weak acid, NaH2PO4, and the decrease in pH is minimized. Approximately 60% to 70% of the total chemical buff- When a strong base, such as NaOH, is added to the ering of the body fluids is inside the cells, and most of this buffer system, the OH− is buffered by the H2PO4− to form buffering results from the intracellular proteins. How- additional amounts of HPO4= + H2O: ever, except for the red blood cells, the slow rate at which NaOH + NaH2PO4 → Na2HPO4 + H2O H+ and HCO3− move through the cell membranes often delays the maximum ability of the intracellular proteins In this case, a strong base, NaOH, is traded for a weak to buffer extracellular acid–base abnormalities for several base, Na2HPO4, causing only a slight increase in pH. hours. UNIT V The Body Fluids and Kidneys In addition to the high concentration of proteins in the !0.3 cells, another factor that contributes to their buffering !0.2 pH change in body fluids power is the fact that the pKs of many of these protein !0.1 systems are fairly close to intracellular pH. 0 Normal "0.1 Isohydric Principle: All Buffers in a Common Solution Are in Equilibrium With the Same H+ Concentration "0.2 We have been discussing buffer systems as though they op- "0.3 erate individually in the body fluids. However, they all work "0.4 together because H+ is common to the reactions of all these "0.5 systems. Therefore, whenever there is a change in H+ con- 0.5 1.0 1.5 2.0 2.5 centration in the extracellular fluid, the balance of all the Rate of alveolar ventilation buffer systems changes at the same time. This phenomenon (normal = 1) is called the isohydric principle and is illustrated by the fol- Figure 31-2. Change in extracellular fluid pH caused by an increased lowing formula: or decreased rate of alveolar ventilation, expressed as times normal. HA1 HA 2 HA 3 H+ = K1 × = K2 × = K3 × A1 A2 A3 INCREASING ALVEOLAR VENTILATION K1, K2, and K3 are the dissociation constants of three DECREASES EXTRACELLULAR FLUID H+ respective acids—HA1, HA2, HA3—and A1, A2, A3 are the CONCENTRATION AND RAISES PH concentrations of the free negative ions that constitute the If the metabolic formation of CO2 remains constant, the bases of the three buffer systems. only other factor that affects P%2 in extracellular fluid The implication of this principle is that any condition that changes the balance of one of the buffer systems also is the rate of alveolar ventilation. The higher the alveolar changes the balance of all the others because the buffer sys- ventilation, the lower the P%2. As discussed previously, tems actually buffer one another by shifting H+ back and when CO2 concentration increases, the H2CO3 concen- forth between them.! tration and H+ concentration also increase, thereby low- ering extracellular fluid pH. Figure 31-2 shows the approximate changes in blood RESPIRATORY REGULATION OF ACID– pH that are caused by increasing or decreasing the alveo- BASE BALANCE lar ventilation rate. Note that increasing alveolar ventila- The second line of defense against acid–base distur- tion to about twice normal raises extracellular fluid pH bances is control of extracellular fluid CO2 concentration by about 0.23. If the pH of the body fluids is 7.40 with by the lungs. An increase in ventilation eliminates CO2 normal alveolar ventilation, doubling the ventilation rate from extracellular fluid, which, by mass action, reduces raises the pH to about 7.63. Conversely, a decrease in the H+ concentration. Conversely, decreased ventilation alveolar ventilation to one-fourth normal reduces the pH increases CO2 and H+ concentrations in the extracellular by 0.45. That is, if the pH is 7.4 at a normal alveolar ven- fluid. tilation, reducing the ventilation to one-fourth normal reduces the pH to 6.95. Because the alveolar ventilation rate can change markedly, from as low as 0 to as high as PULMONARY EXPIRATION OF CO2 15 times normal, one can easily understand how much BALANCES METABOLIC FORMATION OF CO2 the pH of the body fluids can be changed by the respira- CO2 is formed continually in the body by intracellular tory system.! metabolic processes. After it is formed, it diffuses from the cells into the interstitial fluids and blood, and the flow- INCREASED H+ CONCENTRATION ing blood transports it to the lungs, where it diffuses into STIMULATES ALVEOLAR VENTILATION the alveoli and then is transferred to the atmosphere by pulmonary ventilation. About 1.2 mol/L of dissolved CO2 Not only does the alveolar ventilation rate influence H+ is normally in the extracellular fluid, corresponding to a concentration by changing P%2 of the body fluids, but P%2 of 40 mm Hg. the H+ concentration affects the rate of alveolar ventila- If the rate of metabolic formation of CO2 increases, the tion. Thus, Figure 31-3 shows that alveolar ventilation rate P%2 of the extracellular fluid is likewise increased. Con- increases four to five times normal as pH decreases from versely, a decreased metabolic rate lowers the P%2. If the the normal value of 7.4 to the strongly acidic value of 7.0. rate of pulmonary ventilation is increased, CO2 is blown Conversely, a rise in plasma pH above 7.4 causes a decrease off from the lungs, and the P%2 in the extracellular fluid in the ventilation rate. The change in ventilation rate per decreases. Therefore, changes in pulmonary ventilation or unit pH change is much greater at reduced levels of pH the rate of CO2 formation by the tissues can change the (corresponding to elevated H+ concentration) compared extracellular fluid P%2.! with increased levels of pH. The reason for this is that as the Chapter 31 Acid–Base Regulation tracellular fluid, and the pH falls from 7.4 to 7.0, the res- Alveolar ventilation (normal = 1) 4 piratory system can return the pH to a value of about 7.2 to 7.3. This response occurs within 3 to 12 minutes. As 3 discussed previously, the respiratory responses to meta- bolic alkalosis are limited by hypoxemia associated with reduced alveolar ventilation.! UNIT V 2 Buffering Power of the Respiratory System. Respira- 1 tory regulation of acid–base balance is a physiological type of buffer system because it acts rapidly and keeps 0 the H+ concentration from changing too much until the 7.0 7.1 7.2 7.3 7.4 7.5 7.6 slowly responding kidneys can eliminate the imbalance. pH of arterial blood In general, the overall buffering power of the respiratory Figure 31-3. Effect of blood pH on the alveolar ventilation rate. system is one to two times as great as the buffering power of all other chemical buffers in the extracellular fluid com- alveolar ventilation rate decreases, as a result of an increase bined. That is, one to two times as much acid or base can in pH (decreased H+ concentration), the amount of oxygen normally be buffered by this mechanism as by the chemi- added to the blood decreases, and the partial pressure of cal buffers.! oxygen (P%2) in the blood also decreases, which stimulates the ventilation rate. Therefore, the respiratory compensa- Impairment of Lung Function Can Cause Respiratory tion for an increase in pH is not nearly as effective as the Acidosis. We have discussed thus far the role of the response to a marked reduction in pH. normal respiratory mechanism as a means of buffering changes in H+ concentration. However, abnormalities Feedback Control of H+ Concentration by the Res- of respiration can also cause changes in H+ concen- piratory System. Because increased H+ concentration tration. For example, an impairment of lung function, stimulates respiration and because increased alveolar such as severe emphysema, decreases the ability of the ventilation decreases H+ concentration, the respiratory lungs to eliminate CO2, which causes a buildup of CO2 system acts as a typical negative feedback controller of H+ in the extracellular fluid and a tendency toward respir- concentration: atory acidosis. Also, the ability to respond to metabolic acidosis is impaired because the compensatory reduc- ↑[H ] → ↑Alveolar ventilation tions in P%2 that would normally occur by means of ↓ increased ventilation are blunted. In these circum- ↓PCO2 stances, the kidneys represent the sole remaining phys- That is, whenever H+ concentration increases above iological mechanism for returning pH toward normal normal, the respiratory system is stimulated, and alveo- after the initial chemical buffering in the extracellular lar ventilation increases. This mechanism decreases the fluid has occurred.! P%2 in extracellular fluid and reduces H+ concentration back toward normal. Conversely, if H+ concentration falls RENAL CONTROL OF ACID–BASE below normal, the respiratory center becomes depressed, BALANCE alveolar ventilation decreases, and H+ concentration increases back toward normal. Although alkalosis tends The kidneys control acid–base balance by excreting acidic to depress the respiratory centers, the response is gen- or basic urine. Excreting acidic urine reduces the amount erally less robust and less predictable than the response of acid in extracellular fluid, whereas excreting basic urine to metabolic acidosis; the hypoxemia associated with removes base from the extracellular fluid. reduced alveolar ventilation eventually activates oxygen- The overall mechanism whereby the kidneys excrete sensitive chemoreceptors that tend to stimulate ventila- acidic or basic urine is as follows. Large amounts of tion and limit the respiratory compensation for metabolic HCO3− are filtered continuously into the tubules and, alkalosis.! if excreted into the urine, remove base from the blood. Large numbers of H+ are also secreted into the tubular Efficiency of Respiratory Control of H+ Concentration. lumen by the tubular epithelial cells, thus removing acid Respiratory control cannot return the H+ concentration from the blood. If more H+ is secreted than HCO3− is fil- all the way back to normal when a disturbance outside tered, there will be a net loss of acid from the extracellu- the respiratory system has altered the pH. Ordinarily, the lar fluid. Conversely, if more HCO3− is filtered than H+ is respiratory mechanism for controlling H+ concentration secreted, there will be a net loss of base. is approximately 50% to 75% effective, corresponding to Each day, the body produces about 80 mEq of nonvola- a feedback gain of 1 to 3 for metabolic acidosis. That is, tile acids, mainly from metabolism of proteins. These acids if the pH is suddenly decreased by adding acid to the ex- are called nonvolatile because they are not H2CO3 and, 409 UNIT V The Body Fluids and Kidneys therefore, cannot be excreted by the lungs. The primary 85% mechanism for removal of these acids from the body is (3672 mEq/day) 4320 renal excretion. The kidneys must also prevent the loss of mEq/day bicarbonate in the urine, a task that is quantitatively more important than the excretion of nonvolatile acids. Each day, the kidneys filter about 4320 mEq of HCO3− (180 L/ >4.9% day × 24 mEq/L); under normal conditions, almost all of (215 10% mEq/day) this is reabsorbed from the tubules, thereby conserving (432 the primary buffer system of the extracellular fluid. mEq/day) As discussed later, both the reabsorption of HCO3− and excretion of H+ are accomplished through the pro- cess of H+ secretion by the tubules. Because HCO3− must react with a secreted H+ to form H2CO3 before it can be reabsorbed, 4320 mEq of H+ must be secreted each day just to reabsorb the filtered HCO3−. Then, an additional 80 mEq of H+ must be secreted to rid the body of the non- volatile acids produced each day for a total of 4400 mEq of H+ secreted into the tubular fluid each day. When there is a reduction in the extracellular fluid H+ concentration (alkalosis), the kidneys usually secrete less H+ (1 mEq/day) and fail to reabsorb all the filtered HCO3−, thereby increas- Figure 31-4. Reabsorption of HCO3− in different segments of the ing the excretion of HCO3−. Because HCO3− normally buf- renal tubule. The percentages of the filtered load of HCO3− absorbed fers H+ in the extracellular fluid, this loss of HCO3− is the by the various tubular segments are shown, as well as the number of milliequivalents reabsorbed per day under normal conditions. same as adding an H+ to the extracellular fluid. Therefore, in alkalosis, the removal of HCO3− raises the extracellular fluid H+ concentration back toward normal. H+ SECRETED BY SECONDARY ACTIVE In acidosis, the kidneys secrete additional H+ and do TRANSPORT IN EARLY TUBULAR not excrete HCO3− into the urine but reabsorb all the fil- SEGMENTS tered HCO3− and produce new HCO3−, which is added back to the extracellular fluid. This action reduces the The epithelial cells of the proximal tubule, the thick seg- extracellular fluid H+ concentration back toward normal. ment of the ascending loop of Henle, and the early distal Thus, the kidneys regulate extracellular fluid H+ con- tubule all secrete H+ into the tubular fluid by sodium- centration through three fundamental mechanisms: (1) hydrogen counter-transport, as shown in Figure 31-5. secretion of H+; (2) reabsorption of filtered HCO3−; and (3) This secondary active secretion of H+ is coupled with production of new HCO3−. All these processes are accom- the transport of Na+ into the cell at the luminal mem- plished through the same basic mechanisms, as discussed brane by the sodium-hydrogen exchanger protein, and in the next few sections.! the energy for H+ secretion against a concentration gra- dient is derived from the sodium gradient favoring Na+ movement into the cell. This gradient is established by SECRETION OF H+ AND REABSORPTION the sodium-potassium adenosine triphosphatase (Na+-K+ OF HCO3− BY THE RENAL TUBULES ATPase) pump in the basolateral membrane. About 95% Hydrogen ion secretion and HCO3− reabsorption occur in of the bicarbonate is reabsorbed in this manner, requir- virtually all parts of the tubules except the descending and ing about 4000 mEq of H+ to be secreted each day by the ascending thin limbs of the loop of Henle. Figure 31-4 tubules. This mechanism, however, can establish a mini- summarizes HCO3− reabsorption along the tubule. Keep mum pH of only about 6.7; the tubular fluid becomes very in mind that for each HCO3− reabsorbed, an H+ must be acidic only in the collecting tubules and collecting ducts, secreted. which can establish a urine pH as low as about 4.5. About 80% to 90% of the HCO3− reabsorption (and Figure 31-5 shows how the process of H+ secretion H+ secretion) occurs in the proximal tubule, so only a achieves HCO3− reabsorption. The secretory process small amount of HCO3− flows into the distal tubules and begins when CO2 either diffuses into the tubular cells or collecting ducts. In the thick ascending loop of Henle, is formed by metabolism in the tubular epithelial cells. another 10% of the filtered HCO3− is reabsorbed, and the Under the influence of the enzyme carbonic anhydrase, remainder of the reabsorption takes place in the distal CO2, combines with H2O to form H2CO3, which disso- tubules and collecting ducts. As discussed previously, the ciates into HCO3− and H+. The H+ is secreted from the mechanism whereby HCO3− is reabsorbed also involves cell into the tubular lumen by sodium-hydrogen counter- tubular secretion of H+, but different tubular segments transport. That is, when Na+ moves from the lumen of the accomplish this task differently. tubule to the interior of the cell, it first combines with a Chapter 31 Acid–Base Regulation Renal Tubular the late segments of the proximal tubule, thick ascending interstitial Tubular cells lumen loop of Henle, and collecting tubules and ducts. fluid Na! ! HCO3– Thus, each time a H+ is formed in the tubular epithelial cells, an HCO3− is also formed and released back into the Na! Na! blood. The net effect of these reactions is “reabsorption” ATP of HCO3− from the tubules, although the HCO3− that UNIT V K! H! HCO3– + H! actually enters the extracellular fluid is not the same as H2CO3 that filtered into the tubules. The reabsorption of filtered Na! HCO3− does not result in net secretion of H+ because H2CO3 Carbonic the secreted H+ combines with the filtered HCO3− and is H2O anhydrase therefore not excreted. CO2 + CO2 CO2 ! H2O HCO3− Is Titrated Against H+ in the Tubules. Under nor- mal conditions, the rate of tubular H+ secretion is about Figure 31-5. Shown are the cellular mechanisms for the following: (1) 4400 mEq/day, and the rate of filtration by HCO3− is about active secretion of H+ into the renal tubule; (2) tubular reabsorption 4320 mEq/day. Thus, the quantities of these two ions enter- of HCO3− by combination with H+ to form H2CO3, which dissociates ing the tubules are almost equal, and they combine with to form CO2 and H2O; and (3) Na+ reabsorption in exchange for H+ secreted. This pattern of H+ secretion occurs in the proximal tubule, each other to form CO2 and H2O. Therefore, it is said that thick ascending segment of the loop of Henle, and early distal tubule. HCO3− and H+ normally “titrate” each other in the tubules. The titration process is not quite exact because there is usually a slight excess of H+ in the tubules to be excreted carrier protein in the luminal border of the cell membrane; in the urine. This excess H+ (≈80 mEq/day) rids the body at the same time, a H+ in the interior of the cells combines of nonvolatile acids produced by metabolism. As dis- with the carrier protein. The Na+ moves into the cell down cussed later, most of this H+ is not excreted as free H+ but a concentration gradient that has been established by the is in combination with other urinary buffers, especially Na+-K+ ATPase pump in the basolateral membrane. The phosphate and ammonia. gradient for Na+ movement into the cell then provides the When there is an excess of HCO3− over H+ in the urine, energy for moving H+ in the opposite direction from the as occurs in metabolic alkalosis, the excess HCO3− can- interior of the cell to the tubular lumen. not be reabsorbed. Therefore, the excess HCO3− is left in The HCO3− generated in the cell (when H+ dissociates the tubules and eventually excreted into the urine, which from H2CO3) then moves downhill across the basolateral helps correct the metabolic alkalosis. membrane, into the renal interstitial fluid and peritubular In acidosis, there is excess H+ relative to HCO3−, caus- capillary blood. The net result is that for every H+ secreted ing complete reabsorption of the HCO3−; the excess H+ into the tubular lumen, an HCO3− enters the blood.! passes into the urine in combination with urinary buf- fers, especially phosphate and ammonia, and eventually is FILTERED HCO3− IS REABSORBED BY excreted as salts. Thus, the basic mechanism whereby the INTERACTION WITH H+ IN THE TUBULES kidneys correct acidosis or alkalosis is incomplete titra- tion of H+ against HCO3−, leaving one or the other to pass Bicarbonate ions do not readily permeate the luminal mem- into the urine and be removed from the extracellular fluid.! branes of the renal tubular cells; therefore, HCO3− that is filtered by the glomerulus cannot be directly reabsorbed. PRIMARY ACTIVE SECRETION OF H+ Instead, HCO3− is reabsorbed by a special process in which IN THE INTERCALATED CELLS OF LATE it first combines with H+ to form H2CO3, which eventually DISTAL AND COLLECTING TUBULES becomes CO2 and H2O, as shown in Figure 31-5. This reabsorption of HCO3− is initiated by a reaction Beginning in the late distal tubules and continuing in the tubules between HCO3− filtered at the glomerulus through the remainder of the tubular system, the tubular and H+ secreted by the tubular cells. The H2CO3 formed epithelium secretes H+ by primary active transport. The then dissociates into CO2 and H2O. The CO2 can move characteristics of this transport are different from those easily across the tubular membrane; therefore, it instantly discussed for the proximal tubule, loop of Henle, and early diffuses into the tubular cell, where it recombines with distal tubule. H2O, under the influence of carbonic anhydrase, to gen- The mechanism for primary active H+ secretion was erate a new H2CO3 molecule. This H2CO3 dissociates to discussed in Chapter 28 and is shown in Figure 31- form HCO3− and H+; the HCO3− then diffuses through 6. It occurs at the luminal membrane of the tubular cell, the basolateral membrane into the interstitial fluid and is where H+ is transported directly by specific proteins, a taken up into the peritubular capillary blood. The trans- hydrogen-transporting ATPase and a hydrogen-potassium- port of HCO3 across the basolateral membrane is facili- ATPase transporter. The energy required for pumping the tated by two mechanisms: (1) Na+-HCO3− co-transport H+ is derived from the breakdown of ATP to adenosine in the proximal tubules; and (2) Cl−-HCO3− exchange in diphosphate. UNIT V The Body Fluids and Kidneys Renal Tubular This is because the minimal urine pH is about 4.5, cor- interstitial Type A lumen intercalated cell responding to an H+ concentration of 10−4.5 mEq/L, fluid or 0.03 mEq/L. Thus, for each liter of urine formed, a maximum of only about 0.03 mEq of free H+ can be excreted. To excrete the 80 mEq of nonvolatile acid CO2 CO2 + H2O H+ formed by metabolism each day, about 2667 liters of ATP urine would have to be excreted if the H+ remained free H2CO3 in solution. HCO3– + H+ Cl– The excretion of large amounts of H+ (on occasion as much as 500 mEq/day) in the urine is accomplished pri- HCO3– marily by combining the H+ with buffers in the tubular fluid. The most important buffers are phosphate buffer Cl– H+ ATP and ammonia buffer. Other weak buffer systems, such as K+ K+ urate and citrate, are much less important. When H+ is titrated in the tubular fluid with HCO3−, this leads to reabsorption of one HCO3− for each H+ Figure 31-6. Active secretion of H+ through the luminal membrane secreted, as discussed earlier. However, when there is of the type A intercalated epithelial cells of the late distal and collect- excess H+ in the tubular fluid, it combines with buffers ing tubules. Type A cells contain hydrogen–adenosine triphosphatase other than HCO3−, and this leads to generation of new (ATPase) and hydrogen-potassium-ATPase in the luminal membrane and secrete H+ while reabsorbing HCO3− and K+ in acidosis. Note HCO3− that can also enter the blood. Thus, when there that one HCO3− is reabsorbed for each H+ secreted, and one Cl- is is excess H+ in the extracellular fluid, the kidneys not passively secreted along with H+. only reabsorb all the filtered HCO3− but also generate new HCO3−, thereby helping replenish the HCO3− lost Primary active secretion of H+ occurs in special types of from the extracellular fluid in acidosis. In the next two cells called the type A intercalated cells of the late distal tubule sections, we discuss the mechanisms whereby phosphate and in the collecting tubules. Hydrogen ion secretion in these and ammonia buffers contribute to generation of new cells is accomplished in two steps: (1) the dissolved CO2 in this HCO3−. cell combines with H2O to form H2CO3; and (2) the H2CO3 then dissociates into HCO3−, which is reabsorbed into the PHOSPHATE BUFFER SYSTEM CARRIES blood, plus H+, which is secreted into the tubule by means of EXCESS H+ INTO THE URINE AND the hydrogen-ATPase and the hydrogen-potassium-ATPase GENERATES NEW HCO3− transporters. For each H+ secreted, a HCO3− is reabsorbed, similar to the process in the proximal tubules. The main dif- The phosphate buffer system is composed of HPO4=. Both ference is that H+ moves across the luminal membrane by an become concentrated in the tubular fluid because water is active H+ pump instead of by counter-transport, as occurs in normally reabsorbed to a greater extent than phosphate the early parts of the nephron. by the renal tubules. Therefore, although phosphate is not Although secretion of H+ in the late distal tubule an important extracellular fluid buffer, it is much more and collecting tubules accounts for only about 5% of the effective as a buffer in the tubular fluid. total H+ secreted, this mechanism is important in form- Another factor that makes phosphate important as a ing maximally acidic urine. In the proximal tubules, H+ tubular buffer is the fact that the pK of this system is about concentration can be increased only about threefold to 6.8. Under normal conditions, the urine is slightly acidic, fourfold and the tubular fluid pH can be reduced to only and the urine pH is near the pK of the phosphate buf- about 6.7, although large amounts of H+ are secreted by fer system. Therefore, in the tubules, the phosphate buf- this nephron segment. However, H+ concentration can be fer system normally functions near its most effective pH increased as much as 900-fold in the collecting tubules. range. This mechanism decreases the pH of the tubular fluid Figure 31-7 shows the sequence of events whereby H+ to about 4.5, which is the lower limit of pH that can be is excreted in combination with phosphate buffer and the achieved in normal kidneys.! mechanism whereby new HCO3− is added to the blood. The process of H+ secretion into the tubules is the same as described earlier. As long as there is excess HCO3− in COMBINATION OF EXCESS H+ WITH the tubular fluid, most of the secreted H+ combines with PHOSPHATE AND AMMONIA BUFFERS HCO3−. However, once all the HCO3− has been reab- IN THE TUBULE GENERATES “NEW” sorbed and is no longer available to combine with H+, HCO3− any excess H+ can combine with HPO4= and other tubu- When H+ is secreted in excess of the HCO3− filtered lar buffers. After the H+ combines with HPO4= to form into the tubular fluid, only a small part of the excess H2PO4−, it can be excreted as a sodium salt (NaH2PO4), H+ can be excreted in the ionic form (H+) in the urine. carrying with it the excess H+. Chapter 31 Acid–Base Regulation Renal Tubular Renal Proximal Tubular interstitial Tubular cells lumen interstitial tubular cells lumen fluid Na+ + NaHPO4 fluid Na+ Na+ Na+ ATP UNIT V K+ H+ + NaHPO4 Glutamine Glutamine Glutamine HCO3– HCO3– + H+ Cl– H2CO3 NaH2PO4 2HCO3– 2NH4+ Carbonic anhydrase H2O NH4+ NH4+ + Cl– CO2 + CO2 Na+ Na+ Figure 31-7. Buffering of secreted H+ by filtered phosphate (NaH- Figure 31-8. Production and secretion of ammonium ion (NH4+) by PO4). Note that a new HCO3− is returned to the blood for each NaH- proximal tubular cells. Glutamine is metabolized in the cell, yielding PO4 that reacts with a secreted H+. NH4+ and HCO3−. The NH4+ is secreted into the lumen by a Na+-NH4+ exchanger. For each glutamine molecule metabolized, two NH4+ are produced and secreted, and two HCO3− are returned to the blood. There is one important difference in this sequence of H+ excretion from that discussed previously. In this Renal Tubular case, the HCO3− that is generated in the tubular cell and interstitial Collecting lumen enters the peritubular blood represents a net gain of fluid tubular cells HCO3− by the blood, rather than merely a replacement of filtered HCO3−. Therefore, whenever an H+ secreted into the tubular lumen combines with a buffer other than Na+ NH3 NH3 ATP HCO3−, the net effect is the addition of a new HCO3− to K+ Cl– the blood. This process demonstrates one of the mecha- HCO3 –+ H+ ATP H+ nisms whereby the kidneys can replenish the extracel- lular fluid stores of HCO3−. H2CO3 Under normal conditions, much of the filtered phos- Carbonic NH4+ + Cl– anhydrase phate is reabsorbed, and only 30 to 40 mEq/day are avail- H2O able for buffering H+. Therefore, much of the buffering of CO2 + CO2 excess H+ in the tubular fluid in acidosis occurs through the ammonia buffer system.! Figure 31-9. Buffering of H+ secretion by ammonia (NH3) in the col- lecting tubules. NH3 diffuses into the tubular lumen, where it reacts EXCRETION OF EXCESS H+ AND with secreted H+ to form NH4+, which is then excreted. For each NH4+ excreted, a new HCO3− is formed in the tubular cells and returned GENERATION OF NEW HCO3− BY to the blood. AMMONIA BUFFER SYSTEM A second buffer system in the tubular fluid that is even urine and two HCO3− are reabsorbed into the blood. The more important quantitatively than the phosphate buffer HCO3− generated by this process constitutes new HCO3−. system is composed of ammonia (NH3) and the ammo- In the collecting tubules, the addition of NH4+ to the nium ion (NH4+). Ammonium ion is synthesized from tubular fluids occurs through a different mechanism (Fig- glutamine, which comes mainly from metabolism of ure 31-9). Here, H+ is actively secreted by the tubular amino acids in the liver. The glutamine delivered to the membrane into the lumen, where it combines with NH3 kidneys is transported into epithelial cells of the proximal to form NH4+, which is then excreted. The collecting ducts tubules, thick ascending limb of the loop of Henle, and are permeable to NH3, which can easily diffuse into the distal tubules (Figure 31-8). Once inside the cell, each tubular lumen. However, the luminal membrane of this molecule of glutamine is metabolized in a series of reac- part of the tubules is much less permeable to NH4+; there- tions to ultimately form two NH4+ and two HCO3−. The fore, once the H+ has reacted with NH3 to form NH4+, the NH4+ is secreted into the tubular lumen by a counter- NH4+ is trapped in the tubular lumen and eliminated in transport mechanism in exchange for sodium, which is the urine. For each NH4+ excreted, a new HCO3− is gener- reabsorbed. The HCO3− is transported across the basolat- ated and added to the blood. eral membrane, along with the reabsorbed Na+, into the interstitial fluid and is taken up by the peritubular capil- Chronic Acidosis Increases NH4+ Excretion. One of laries. Thus, for each molecule of glutamine metabolized the most important features of the renal ammonium- in the proximal tubules, two NH4+ are secreted into the ammonia buffer system is that it is subject to physiological UNIT V The Body Fluids and Kidneys control. An increase in extracellular fluid H+ concentra- The reason we subtract HCO3− excretion is that the loss tion stimulates renal glutamine metabolism and, there- of HCO3− is the same as the addition of H+ to the blood. fore, increases formation of NH4+ and new HCO3− to be To maintain acid–base balance, the net acid excretion must used in H+ buffering; a decrease in H+ concentration has equal the nonvolatile acid production in the body. the opposite effect. In acidosis, the net acid excretion increases markedly, Under normal conditions, the amount of H+ eliminated especially because of increased NH4+ excretion, thereby by the ammonia buffer system accounts for about 50% of removing acid from the blood. The net acid excretion also the acid excreted and 50% of the new HCO3− generated equals the rate of net HCO3− addition to the blood. There- by the kidneys. However, with chronic acidosis, the rate of fore, in acidosis, there is a net addition of HCO3− back to NH4+ excretion can increase to as much as 500 mEq/day. the blood as more NH4+ and urinary titratable acid are Therefore, with chronic acidosis, the dominant mechanism excreted. for acid elimination is excretion of NH4+. This process also In alkalosis, titratable acid and NH4+ excretion drop to provides the most important mechanism for generating 0, whereas HCO3− excretion increases. Therefore, in alka- new bicarbonate during chronic acidosis.! losis, there is a negative net acid secretion, which means that there is a net loss of HCO3− from the blood, which is the same as adding H+ to the blood, and that no new QUANTIFYING RENAL ACID–BASE HCO3− is generated by the kidneys.! EXCRETION Based on the principles discussed earlier, we can quantify REGULATION OF RENAL TUBULAR H+ the kidneys’ net excretion of acid or net addition or elimi- SECRETION nation of HCO3− from the blood as follows. Bicarbonate excretion is calculated as urine flow rate As discussed earlier, H+ secretion by the tubular epithe- multiplied by the urinary HCO3− concentration and indi- lium is necessary for HCO3− reabsorption and generation cates how rapidly the kidneys are removing HCO3− from of new HCO3− associated with titratable acid formation. the blood, which is the same as adding an H+ to the blood. Therefore, the rate of H+ secretion must be carefully In alkalosis, the loss of HCO3− helps return the plasma pH regulated if the kidneys are to perform their functions in toward normal. acid–base homeostasis effectively. Under normal condi- The amount of new HCO3− contributed to the blood at tions, the kidney tubules must secrete at least enough H+ any given time is equal to the amount of H+ secreted that to reabsorb almost all the HCO3− that is filtered, and there ends up in the tubular lumen with non-bicarbonate uri- must be enough H+ left over to be excreted as titratable nary buffers. As discussed previously, the primary sources acid or NH4+ to rid the body of the nonvolatile acids pro- of nonbicarbonate urinary buffers are NH4+ and phos- duced each day from metabolism. phate. Therefore, part of the HCO3− added to the blood In alkalosis, the tubular secretion of H+ is reduced to a (and H+ excreted by NH4+) is calculated by measuring level that is too low to achieve complete HCO3− reabsorp- NH4+ excretion (urine flow rate multiplied by urinary tion, enabling the kidneys to increase HCO3− excretion. NH4+ concentration). Titratable acid and ammonia are not excreted in alka- The rest of the non-bicarbonate, non-NH4+ buf- losis because there is no excess H+ available to combine fer excreted in the urine is measured by determining a with nonbicarbonate buffers; therefore, no new HCO3− is value known as titratable acid. The amount of titratable added to the blood in alkalosis. acid in the urine is measured by titrating the urine with During acidosis, the tubular H+ secretion is increased a strong base, such as NaOH, to a pH of 7.4, the pH of sufficiently to reabsorb all the filtered HCO3−, with normal plasma and the pH of the glomerular filtrate. This enough H+ left over to excrete large amounts of NH4+ titration reverses the events that occurred in the tubular and titratable acid, thereby contributing large amounts lumen when the tubular fluid was titrated by secreted of new HCO3− to the total body extracellular fluid. The H+. Therefore, the number of milliequivalents of NaOH most important stimuli for increasing H+ secretion by the required to return the urinary pH to 7.4 equals the num- tubules in acidosis are as follows: (1) an increase in P2 ber of milliequivalents of H+ added to the tubular fluid of the extracellular fluid in respiratory acidosis; and (2) that combined with phosphate and other organic buffers. an increase in H+ concentration of the extracellular fluid The titratable acid measurement does not include H+ in (decreased pH) in respiratory or metabolic acidosis. association with NH4+ because the pK of the ammonia- The tubular cells respond directly to an increase in ammonium reaction is 9.2, and titration with NaOH to a P%2 of the blood, as in respiratory acidosis, with an pH of 7.4 does not remove the H+ from NH4+. increase in the rate of H+ secretion, as follows. The Thus, the net acid excretion by the kidneys can be increased P%2 raises the P%2 of the tubular cells, caus- assessed as follows: ing increased formation of H+ in the tubular cells, which in turn stimulates secretion of H+. The second factor that Net acid excretion = NH4 + excretion + Urinary stimulates H+ secretion is an increase in extracellular fluid titratable acid − HCO3− excretion H+ concentration (decreased pH). Chapter 31 Acid–Base Regulation Table 31-2 Plasma or Extracellular Fluid Factors HCO3− reabsorption and leads to alkalosis. Hyperkale- at n rease or e rease + Secretion and HCO3− mia decreases H+ secretion and HCO3− reabsorption and ea sor tion t e enal les tends to cause acidosis.! Increase H+ Secretion and Decrease H+ Secretion and HCO3− Reabsorption HCO3− Reabsorption RENAL CORRECTION OF ACIDOSIS— ↑PCO2 ↓PCO2 INCREASED EXCRETION OF H+ UNIT V ↑H+, ↓HCO3− ↓H+, ↑HCO3− AND ADDITION OF HCO3− TO THE EXTRACELLULAR FLUID ↓ tra ell lar id ol e ↑ tra ell lar id ol e ↑Angiotensin ↓Angiotensin Now that we have described the mechanisms whereby the kidneys secrete H+ and reabsorb HCO3−, we can explain ↑Aldosterone ↓Aldosterone how the kidneys readjust the pH of the extracellular fluid Hypokalemia Hyperkalemia when it becomes abnormal. Referring to Equation 8, the Henderson-Hasselbalch equation, we can see that acidosis occurs when the ratio A special factor that can increase H+ secretion under of HCO3− to CO2 in the extracellular fluid decreases, some pathophysiological conditions is excessive aldoste- thereby decreasing the pH. If this ratio decreases because rone secretion. Aldosterone stimulates secretion of H+ by of a fall in HCO3−, the acidosis is referred to as metabolic type A intercalated cells of the collecting tubules and ducts. acidosis. If the pH falls because of an increase in P%2, the Therefore, excessive secretion of aldosterone, as in persons acidosis is referred to as respiratory acidosis. with Conn syndrome, increases secretion of H+ into the tubular fluid and, consequently, increases the amount of ACIDOSIS DECREASES HCO3−/H+ RATIO IN HCO3− added back to the blood. This action usually causes RENAL TUBULAR FLUID alkalosis in patients with excessive aldosterone secretion. The tubular cells usually respond to a decrease in H+ Respiratory and metabolic acidosis both cause a decrease concentration (alkalosis) by reducing H+ secretion. The in the ratio of HCO3−/H+ in the renal tubular fluid. As decreased H+ secretion results from decreased extracel- a result, there is excess H+ in the renal tubules, causing lular P%2, as in respiratory alkalosis, or from a decrease complete reabsorption of HCO3− and still leaving addi- in H+ concentration, as in both respiratory and metabolic tional H+ available to combine with the urinary buffers alkalosis. NH4+ and HPO4=. Thus, in acidosis, the kidneys reabsorb Table 31-2 summarizes the major factors that influ- all the filtered HCO3− and contribute new HCO3− through ence H+ secretion and HCO3− reabsorption. Some of formation of NH4+ and titratable acid. these factors are not directly related to the regulation of In metabolic acidosis, an excess of H+ over HCO3− acid–base balance. For example, H+ secretion is coupled occurs in the tubular fluid, primarily because of decreased to Na+ reabsorption by the Na+-H+ exchanger in the extracellular fluid concentration of HCO3− and therefore proximal tubule and the thick ascending loop of Henle. decreased glomerular filtration of HCO3−. Therefore, factors that stimulate Na+ reabsorption, such In respiratory acidosis, the excess H+ in the tubular as decreased extracellular fluid volume and increased fluid is due mainly to the rise in extracellular fluid P%2, angiotensin II (Ang II), may also secondarily increase H+ which stimulates H+ secretion. secretion and HCO3− reabsorption. As discussed previously, with chronic acidosis, regard- Extracellular fluid volume depletion stimulates less of whether it is respiratory or metabolic, there is sodium reabsorption by the renal tubules and increases increased production of NH4+, which further contributes H+ secretion and HCO3− reabsorption through multiple to excretion of H+ and addition of new HCO3− to the mechanisms, including the following: (1) increased Ang extracellular fluid. With severe chronic acidosis, as much II levels, which directly stimulate activity of the Na+- as 500 mEq/day of H+ can be excreted in the urine, mainly H+ exchanger in the renal tubules; and (2) increased in the form of NH4+; this excretion, in turn, contributes up aldosterone levels, which stimulate H+ secretion by to 500 mEq/day of new HCO3− that is added to the blood. the intercalated cells of the cortical collecting tubules. Thus, with chronic acidosis, increased secretion of H+ Therefore, extracellular fluid volume depletion tends by the tubules helps eliminate excess H+ from the body to cause alkalosis due to excess H+ secretion and and increases the quantity of HCO3− in the extracellu- HCO3− reabsorption. lar fluid. This process increases the HCO3− part of the Changes in plasma potassium concentration can bicarbonate buffer system, which, in accordance with the also influence H+ secretion, with hypokalemia stimu- Henderson-Hasselbalch equation, helps raise the extra- lating and hyperkalemia inhibiting H+ secretion in the cellular pH and corrects the acidosis. If the acidosis is proximal tubule. Decreased plasma potassium concen- metabolically mediated, additional compensation by the tration tends to increase H+ concentration in the renal lungs causes a reduction in P%2, also helping correct the tubular cells. This, in turn, stimulates H+ secretion and acidosis. UNIT V The Body Fluids and Kidneys Table 31-3 Extracellular Fluid Characteristics of in the urine. Thus, in alkalosis, HCO3− is removed from Pri ar A id ase ist r an esa the extracellular fluid by renal excretion, which has the pH H+ PCO2 HCO3− same effect as adding an H+ to the extracellular fluid. This process helps return the H+ concentration and pH Normal 40 mEq/L g 24 mEq/L toward normal. Respiratory ↓ ↑ ↑↑ ↑ Table 31-3 shows the overall extracellular fluid char- acidosis acteristics of respiratory and metabolic alkalosis. In Respiratory ↑ ↓ ↓↓ ↓ respiratory alkalosis, there is an increase in extracellular alkalosis fluid pH and a decrease in H+ concentration. The cause of eta oli ↓ ↑ ↓ ↓↓ the alkalosis is decreased plasma P2, caused by hyper- acidosis ventilation. Reduction in P%2 then leads to decreased eta oli ↑ ↓ ↑ ↑↑ renal tubular H+ secretion. Consequently, there is not alkalosis enough H+ in the renal tubular fluid to react with all the aThe primary event is indicated by the double arrows (↑↑ or ↓↓). Note that respiratory acid–base disorders are initiated by an increase HCO3− that is filtered. Therefore, the HCO3− that cannot or decrease in PCO2, whereas metabolic disorders are initiated by react with H+ is not reabsorbed and is excreted in the an increase or decrease in HCO3−. urine. This results in a decreased plasma HCO3− con- centration and correction of the alkalosis. Therefore, the compensatory response to a primary reduction in P2 Table 31-3 summarizes the extracellular fluid char- in respiratory alkalosis is a reduction in plasma HCO3− acteristics associated with respiratory and metabolic concentration, caused by increased renal excretion of acidosis, as well as respiratory and metabolic alkalo- HCO3−. sis, discussed in the next section. Note that in respi- In metabolic alkalosis, there is also decreased plasma ratory acidosis, there is a reduction in pH, an increase H+ concentration and increased pH. The cause of met- in extracellular fluid H+ concentration, and an increase abolic alkalosis, however, is a rise in the extracellular in P%2, which is