GROUP 3 Bones and Cartilage PDF
Document Details
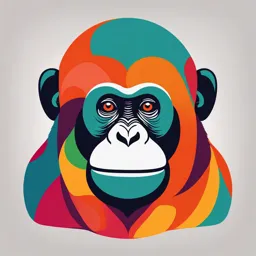
Uploaded by AdjustableCharacterization
Tags
Summary
This document provides an overview of cartilage and bone tissues, including their structure, function, and different types. It details the composition of the extracellular matrix, the roles of chondrocytes, and the processes of development and repair in both tissues. The document also discusses the various types of joints and their importance in the skeletal system.
Full Transcript
GROUP 3 CARTILAGE AND BONE OBJECTIVES The students are expected to: 1. Gain a basic understanding of the structure and types of cartilage in the human body. 2. Explore the composition and functions of both cartilage and bone tissues. 3. Recognize the different cell ty...
GROUP 3 CARTILAGE AND BONE OBJECTIVES The students are expected to: 1. Gain a basic understanding of the structure and types of cartilage in the human body. 2. Explore the composition and functions of both cartilage and bone tissues. 3. Recognize the different cell types involved in cartilage and bone. 4. Understand the processes of cartilage and bone development and repair. 5. Distinguish between various forms of bone tissue. 6. Discuss the general functions of bone in the body. 7. Learn about the different types and roles of joints in the skeletal system. CARTILAGE CARTILAGE? WHAT IS Cartilage is a tough, durable form of supporting connective tissue, characterized by an extracellular matrix (ECM) with high concentrations of GAGs and proteoglycans, interacting with collagen and elastic fibers. Structural features of its matrix make cartilage ideal for a variety of mechanical and protective roles within the adult skeleton and elsewhere Cartilage ECM has a firm consistency that allows the tissue to bear mechanical stresses without permanent distortion. In the respiratory tract, ears, and nose, The physical properties of cartilage depend on electrostatic bonds between type II collagen fibrils, hyaluronan, and the sulfated GAGs on densely packed proteoglycans. Its semi-rigid consistency is attributable to water bound to the negatively charged hyaluronan and GAG chains extending from proteoglycan core proteins, which in turn are enclosed within a dense meshwork of thin type II collagen fibrils. The high content of bound water allows cartilage to serve as a shock absorber, an important functional role. ,cartilage forms the framework supporting softer tissues. Because of its resiliency and smooth, lubricated surface, cartilage provides cushioning and sliding regions within skeletal joints and facilitates bone movements. Cartilage consists of cells called chondrocytes (Gr. chondros, cartilage + kytos, cell) embedded in the ECM which unlike connective tissue proper contains no other cell types. Chondrocytes synthesize and maintain all ECM components and are located in matrix cavities called lacunae All types of cartilage lack vascular supplies and chondrocytes receive nutrients by diffusion from capillaries in surrounding connective tissue (the perichondrium). In some skeletal elements, large blood vessels do traverse cartilage to supply other tissues, but these vessels release few nutrients to the chondrocytes. As might be expected of cells in an avascular tissue, chondrocytes exhibit low metabolic activity. Cartilage also lacks nerves. The perichondrium is a sheath of dense connective tissue that surrounds cartilage in most places, MEDICAL APPLICATION forming an interface between the cartilage and the tissues supported Many genetic conditions in humans by the cartilage. The perichondrium or mice that cause defective harbors the blood supply serving the cartilage, joint deformities, or short cartilage and a small neural limbs are due to recessive component. Articular cartilage, which mutations in genes for collagen type covers the ends of bones in movable II, the aggrecan core protein, the joints and which erodes in the course sulfate transporter, and other of arthritic degeneration, lacks proteins required for normal perichondrium and is sustained by the chondrocyte function. diffusion of oxygen and nutrients from the synovial fluid. S OF CARTILAGE TYPE HYALINE CARTILAGE Hyaline cartilage, the most common of the three types, is homogeneous and semitransparent in the fresh state. In adults hyaline cartilage is located in the articular surfaces of movable joints, in the walls of larger respiratory passages (nose, larynx, trachea, bronchi), in the ventral ends of ribs, where they articulate with the sternum, and in the epiphyseal plates of long bones, where it makes possible longitudinal bone growth. In the embryo, hyaline cartilage forms the temporary skeleton that is gradually replaced by bone. MATRIX The matrix is part of the extracellular material found in connective tissues like cartilage. It is not a cellular component but rather a mixture of proteins, fibers, and other substances that provide structural and biochemical support to the surrounding cells. The dry weight of hyaline cartilage is Aggrecan (250 kDa), with approximately 150 nearly 40% collagen embedded in a firm, GAG side chains of chondroitin sulfate and hydrated gel of proteoglycans and keratan sulfate, is the most abundant structural glycoproteins. In routine proteoglycan of hyaline cartilage. Hundreds histology preparations, the proteoglycan of these proteoglycans are bound noncovalently by link proteins to long make the matrix generally basophilic and polymers of hyaluronan. These proteoglycan the thin collagen fibrils are barely complexes bind further to the surface of discernible. Most of the collagen in type II collagen fibrils (Figure 7–2a). Water hyaline cartilage is type II, although small bound to GAGs in the proteoglycans amounts of minor collagens are also constitutes up to 60%-80% of the weight of present. fresh hyaline cartilage Another important component of cartilage matrix is the structural multiadhesive glycoprotein chondronectin. Like fibronectin in other connective tissues, chondronectin binds specifically to GAGs, collagen, and integrins, mediating the adherence of chondrocytes to the ECM. Staining variations within the matrix reflect local differences in its molecular composition. Immediately surrounding each chondrocyte, the ECM is relatively richer in GAGs than collagen, often causing these areas of territorial matrix to stain differently from the intervening areas of interterritorial matrix. CHONDROCYTES Chondrocytes are the cells responsible for the synthesis and maintenance of the cartilage matrix. They are a specific type of cell found within cartilage tissue. Cells occupy relatively little of the hyaline During routine histologic preparation, cartilage cartilage mass. At the periphery of the cells and matrix may shrink slightly, leading to cartilage, young chondrocytes or irregularly shaped chondrocytes and their chondroblasts have an elliptic shape, with the retraction from the matrix, though in living tissue, chondrocytes fully occupy their lacunae. Due to long axes parallel to the surface. Deeper in the the avascular nature of cartilage, chondrocytes cartilage, they are round and may appear in respire under low-oxygen tension and metabolize groups of up to eight cells that originate from glucose mainly through anaerobic glycolysis. mitotic divisions of a single chondroblast and Nutrients diffuse to chondrocytes from the are called isogenous aggregates. As the cartilage surface, aided by the movement of chondrocytes become more active in secreting water and solutes during tissue compression and collagens and other ECM components, the decompression, which also defines the maximum aggregated cells are pushed apart and occupy thickness of hyaline cartilage, typically found as separate lacunae small, thin plates. MEDICAL APPLICATION In contrast to other forms of cartilage and most other tissues, hyaline cartilage is susceptible to partial or isolated regions of calcification during aging, especially in the costal cartilage adjacent to the ribs. Calcification of the hyaline matrix, accompanied by degenerative changes in the chondrocytes, is a common part of the aging process and in many respects resembles endochondral ossification by which bone is formed. Chondrocyte synthesis of sulfated GAGs and secretion of proteoglycans are accelerated by many hormones and growth factors. A major regulator of hyaline cartilage growth is the pituitary-derived protein called growth hormone or somatotropin. This hormone acts indirectly, promoting the endocrine release from the liver of insulin-like growth factors, or somatomedins, which directly stimulate the cells of hyaline cartilage. MEDICAL APPLICATION Cells of cartilage can give rise to either benign (chondroma) or slow-growing, malignant (chondrosarcoma) tumors in which cells produce normal matrix components. Chondrosarcomas seldom metastasize and are generally removed surgically PERICHONDRIUM The perichondrium is a dense layer of connective tissue that surrounds most types of cartilage, providing mechanical support and protection. It plays a crucial role in cartilage growth and repair by supplying new chondrocytes. Except in the articular cartilage of The outer region of the perichondrium joints, all hyaline cartilage is covered consists largely of collagen type I by a layer of dense connective fibers and fibroblasts, but an inner tissue, the perichondrium, which is layer adjoining the cartilage matrix essential for the growth and also contains mesenchymal stem cells which provide a source for new maintenance of cartilage chondroblasts that divide and differentiate into chondrocytes. CARTILAGE TYPES OF ELASTIC CARTILAGE C CARTILAGE ELASTI Elastic cartilage is essentially similar to hyaline cartilage except that it contains an abundant network of elastic fibers in addition to a meshwork of collagen type II fibrils (Figures 7–4 and 7–1c), which give fresh elastic cartilage a yellowish color. With appropriate staining the elastic fibers usually appear as dark bundles distributed unevenly through the matrix. More flexible than hyaline cartilage, elastic cartilage is found in the auricle of the ear, the walls of the external auditory canals, the auditory (Eustachian) tubes, the epiglottis, and the upper respiratory tract. Elastic cartilage in these locations includes a perichondrium similar to that of most hyaline cartilage. Throughout elastic cartilage the cells resemble those of hyaline cartilage both physiologically and structurally. CARTILAGE ELASTIC The chondrocytes (C) and overall organization of elastic cartilage are similar to those of hyaline cartilage, but the matrix (M) also contains elastic fibers that can be seen as darker components with proper staining. The abundant elastic fibers provide greater flexibility to this type of cartilage. The section in part b includes perichondrium (P) that is also similar to that of hyaline cartilage. (a) X160; Hematoxylin and orcein. (b) X180; Weigert resorcin and van Gieson. CARTILAGE TYPES OF FIBROCARTILAGE FIBROCARTILAGE Fibrocartilage takes various forms in different structures but is essentially a mingling of hyaline cartilage and dense connective tissue (Figures 7– 5 and 7–1d). It is found in intervertebral discs, in attachments of certain ligaments, and in the pubic symphysis—all places where it serves as very tough, yet cushioning support tissue for bone. FIBROCARTILAGE Chondrocytes of fibrocartilage occur singly and often in aligned isogenous aggregates, producing type II collagen and other ECM components, although the matrix around these chondrocytes is typically sparse. Areas with chondrocytes and hyaline matrix are separated by other regions with fibroblasts and dense bundles of type I collagen which confer extra tensile strength to this tissue (Figure 7–5). The relative scarcity of proteoglycans overall makes fibrocartilage matrix more acidophilic than that of hyaline or elastic cartilage. There is no distinct surrounding perichondrium in fibrocartilage. Intervertebral discs of the spinal column are composed primarily of fibrocartilage and act as lubricated cushions and shock absorbers preventing damage to adjacent vertebrae from abrasive forces or impacts. Held in place by ligaments, intervertebral discs are discussed further with joints in Chapter 8 - Bone. FIBROCARTILAGE Fibrocartilage varies histologically in different structures, but is always essentially a mixture of hyaline cartilage and dense connective tissue. In a small region of intervertebral disc, the axially arranged aggregates of chondrocytes (C) are seen to be surrounded by small amounts of matrix and separated by larger regions with dense collagen and scattered fibroblasts with elongated nuclei (arrows). CARTILAGE FORMATION, GROWTH, & REPAIR CARTILAGE FORMATION, GROWTH, All cartilage forms from embryonic mesenchyme in the process of chondrogenesis (Figure 7–6). The first indication of cell differentiation is the rounding up of the mesenchymal cells, which retract their extensions, multiply rapidly, and become more densely packed together. In general the terms “chondroblasts” and “chondrocytes” respectively refer to the cartilage cells during and after the period of rapid proliferation. At both stages the cells have basophilic cytoplasm rich in RER for collagen synthesis (Figure 7–7). Production of the ECM encloses the cells in their lacunae and then gradually separates chondroblasts from one another. During embryonic development, the cartilage differentiation takes place primarilyfrom the center outward; therefore the more central cells have the characteristics of chondrocytes, whereas the peripheralcells are typical chondroblasts. The superficial mesenchyme develops as the perichondrium. Once formed, the cartilage tissue enlarges both by interstitial growth, involving mitotic division of preexisting chondrocytes, and by appositional growth, which involves chondroblast differentiation from progenitor cells in the perichondrium (Figure 7–2b). In both cases, the synthesis of matrix contributes greatly to the growth of the cartilage. Appositional growth of cartilage is more important during postnatal development, although as described in Chapter 8, interstitial growth in cartilaginous regions within long bones is important in increasing the length of these structures. In articular cartilage, cells and matrix near the articulating surface are gradually worn away and must be replaced from within, because there is no perichondrium to add cells by appositional growth. FORMATION, CARTILAGE GROWTH, Except in young children, damaged cartilage undergoes slow and often incomplete repair, primarily dependent on cells in the perichondrium which invade the injured area and produce new cartilage. In damaged areas the perichondrium produces a scar of dense connective tissue instead of forming new cartilage. The poor capacity of cartilage for repair or regeneration is due in part to its avascularity and low metabolic rate. BONE BONE Bone tissue, the main constituent of the adult skeleton, provides solid support for the body, protects vital organs such as those in the cranial and thoracic cavities, and encloses internal (medullary) cavities containing bone marrow where blood cells are formed. Bone (or osseous) tissue also serves as a reservoir of calcium, phosphate, and other ions that can be released or stored in a controlled fashion to maintain constant concentrations in body fluids. In addition, bones form a system of levers that multiply the forces generated during skeletal muscle contraction and transform them into bodily movements. This mineralized tissue therefore confers mechanical and metabolic functions to the skeleton. Bone is a specialized connective tissue composed of calcified extracellular material, the bone matrix, and following three major cell types : OSTEOCYTES- found in cavities (lacunae) between bone matrix layers (lamellae), with cytoplasmic processes in small canaliculi (L. canalis, canal) that extend into the matrix OSTEOBLASTS- growing cells which synthesize and secrete the organic components of the matrix OSTEOCLASTS- giant, multinucleated cells involved in removing calcified bone matrix and remodeling bone tissue BONE Because metabolites are unable to diffuse through the calcified matrix of bone, the exchanges between osteocytes and blood capillaries depend on communication through the very thin, cylindrical spaces of the canaliculi. All bones are lined on their internal and external surfaces by layers of connective tissue containing osteogenic cells—ENDOSTEUM on the internal surface surrounding the marrow cavity and PERIOSTEUM on the external surface. Because of its hardness, bone cannot be sectioned routinely. Bone matrix is usually softened by immersion in a decalcifying solution before paraffin embedding, or embedded in plastic after fixation and sectioned with a specialized microtome. OSTEOBLASTS OSTEOBLASTS OSTEOBLASTS, originating from mesenchymal stem cells, produce the organic components of bone matrix, including typeI collagen fibers, proteoglycans, and matricellular glycoproteins such as osteonectin. Deposition of the inorganic components of bone also depends on osteoblast activity. Active osteoblasts are located exclusively at the surfaces of bone matrix, to which they are bound by integrins, typically forming a single layer of cuboidal cells joined by adherent and gap junctions (Figure 8–3). When their synthetic activity is completed, some osteoblasts differentiate as osteocytes entrapped in matrixbound lacunae, some flatten and cover the matrix surface as BONE LINING CELLS, and the majority undergo apoptosis. During the processes of matrix synthesis and calcification, osteoblasts are polarized cells with ultrastructural features denoting active protein synthesis and secretion. Matrix components are secreted at the cell surface in contact with existing bone matrix, producing a layer of unique collagen-rich material called OSTEOID between the osteoblast layer and the preexisting bone surface (Figure 8–3). This process of bone appositional growth is completed by subsequent deposition of calcium salts into the newly formed matrix. (a) Diagram showing the relationship of osteoblasts to the newly formed matrix called “osteoid,” bone matrix, and osteocytes. Osteoblasts and most of the larger osteoclasts are part of the endosteum covering the bony trabeculae. (b) The photomicrograph of developing bone shows the location and morphologic differences between active osteoblasts (Ob) and osteocytes (Oc). Rounded osteoblasts, derived from progenitor cells in the adjacent mesenchyme (M), cover a thin layer of lightly stained osteoid (Os) on the surface of the more heavily stained bony matrix (B). Most osteoblasts that are no longer actively secreting osteoid will undergo apoptosis; others differentiate either as flattened bone lining cells on the trabeculae of bony matrix or as osteocytes located within lacunae surrounded by bony matrix. (X300; H&E) OSTEOBLASTS The process of matrix mineralization is not completely understood, but basic aspects of the process are shown in Figure 8–4. Prominent among the noncollagen proteins secreted by osteoblasts is the vitamin K- MEDICAL APPLICATION dependent polypeptide OSTEOCALCIN which 2+ together Cancer originating directly from bone cells with various glycoproteins binds Ca ions and (a primary bone tumor) is fairly uncommon concentrates this mineral locally. Osteoblasts also (0.5% of all cancer deaths), although a release membrane-enclosed MATRIX VESICLES rich in cancer called OSTEOSARCOMA can arise alkaline phosphatase and other enzymes 3- whose activity in osteoprogenitor cells. The skeleton is raises the local concentration of PO4 ions. In the often the site of secondary, METASTATIC microenvironment with high concentrations of both TUMORS , however, arising when cancer these ions, matrix vesicles serve as foci for the cells move into bones via small blood or formation of hydroxyapatite [Ca10 (PO4 ) 6(OH) 2] crystals, lymphatic vessels from malignancies in the first visible step in calcification. These crystals other organs, most commonly the breast, grow rapidly by accretion of more mineral and lung, prostate gland, kidney, or thyroid eventually produce a confluent mass of calcified gland. material embedding the collagen fibers and proteoglycans (Figure 8–4). From their ends adjacent to the bone matrix, osteoblasts secrete type I collagen, several glycoproteins, and proteoglycans. Some of these factors, notably osteocalcin and certain glycoproteins, bind Ca2+ with high affinity, raising the local concentration of these ions. Osteoblasts also release very small membrane-enclosed matrix vesicles containing alkaline phosphatase and other enzymes. These enzymes remove PO4- ions from various matrix macromolecules, creating a high concentration of these ions locally. The high Ca2+ and PO4- ion concentrations cause calcified nanocrystals to form in and around the matrix vesicles. The crystals grow and mineralize further with formation of small growing masses of calcium hydroxyapatite [Ca10(PO4)6(OH)2], which surround the collagen fibers and all other macromolecules. Eventually the masses of hydroxyapatite merge as a confluent solid bony matrix as calcification of the matrix is completed. OSTEOCYTES OSTEOCYTES A cell found within the material of fully formed bone. It lives in a small chamber known as a lacuna, which is found in the calcified matrix of bone. During the transition from osteoblasts to osteocytes, the cells extend many long dendritic processes, which also become surrounded by calcifying matrix. The processes thus come to occupy the many canaliculi, 250-300 nm, radiating from each lacuna. Diffusion of metabolites between osteocytes and blood vessels occurs through the small amount of interstitial fluid in the canaliculi between the bone matrix and the osteocytes and their processes. Osteocytes also communicate with one another and ultimately with nearby osteoblasts and bone lining cells via gap junctions at the ends of their processes. These connections between osteocyte processes and nearly all other bone cells in the extensive lacunar-canalicular network allow osteocytes to serve as mechanosensors detecting the mechanical load on the bone as well as stress- or fatigue-induced microdamage and to trigger remedial activity in osteoblasts and osteoclasts. OSTEOCLASTS OSTEOCLASTS OSTEOCLASTS are very large, motile cells with multiple nuclei (Figure 8–6) that are essential for matrix resorption during bone growth and remodeling. The large size and multinucleated condition of osteoclasts are due to their origin from the fusion of bone marrow-derived monocytes. Osteoclast development requires two polypeptides produced by osteoblasts: macrophage colony stimulating factor (M-CSF) and the receptor activator of nuclear factor-κB ligand (RANKL). In areas of bone undergoing resorption, osteoclasts on the bone surface lie within enzymatically etched depressions or cavities in the matrix known as RESORPTION LACUNAE ( or HOWSHIP LACUNAE). In an active osteoclast, the membrane domain that contacts the bone forms a circular SEALING ZONE that binds the cell tightly to the bone matrix and surrounds an area with many surface projections, called the RUFFLED BORDER This circumferential sealing zone allows the formation of a specialized microenvironment between the osteoclast and the matrix in which bone resorption occurs (Figure 8–6b). Into this subcellular pocket the osteoclast pumps protons to acidify and promote dissolution of the adjacent hydroxyapatite, and releases matrix metalloproteinases and other hydrolytic enzymes from lysosome-related secretory vesicles for the localized digestion of matrix proteins. Osteoclast activity is controlled by local signaling factors from other bone cells. Osteoblasts activated by parathyroid hormone produce M-CSF, RANKL, and other factors that regulate the formation and activity of osteoclasts. MEDICAL APPLICATION In the genetic disease OSTEOPETROSIS, which is characterized by dense, heavy bones (“marble bones”), the osteoclasts lack ruffled borders and bone resorption is defective. This disorder results in overgrowth and thickening of bones, often with obliteration of the marrow cavities, depressing blood cell formation and causing anemia and the loss of white blood cells. The defective osteoclasts in most patients with osteopetrosis have mutations in genes for the cells’ proton-ATPase pumps or chloride channels. BONE MATRIX About 50% of the dry weight of bone matrix is inorganic materials. Calcium hydroxyapatite is most abundant, but bicarbonate, citrate, magnesium, potassium, and sodium ions are also found. Significant quantities of noncrystalline calcium phosphate are also present. The surface of hydroxyapatite crystals are hydrated, facilitating the exchange of ions between the mineral and body fluids. The organic matter embedded in the calcified matrix is 90% type I collagen, but also includes mostly small proteoglycans and multiadhesive glycoproteins such as osteonectin. Calcium-binding proteins, notably osteocalcin, and the phosphatases released from cells in matrix vesicles promote calcification of the matrix. Other tissues rich in type I collagen lack osteocalcin and matrix vesicles and therefore do not normally become calcified. The association of minerals with collagen fibers during calcification provides the hardness and resistance required for bone function. If a bone is decalcified by a histologist, its shape is preserved but it becomes soft and pliable like other connective tissues. Because of its high collagen content, decalcified bone matrix is usually acidophilic. PERIOSTEUM AND ENDOSTEUM External and internal surfaces of all bones are covered by connective tissue of the periosteum and endosteum, respectively. The periosteum is organized much like the perichondrium of cartilage, with an outer fibrous layer of dense connective tissue, containing mostly bundled type I collagen, but also fibroblasts and blood vessels. Bundles of periosteal collagen, called perforating (or Sharpey) fibers, penetrate the bone matrix and bind the periosteum to the bone. Periosteal blood vessels branch and penetrate the bone, carrying metabolites to and from bone cells. The periosteum’s inner layer is more cellular and includes osteoblasts, bone lining cells, and mesenchymal stem cells referred to as osteoprogenitor cells. With the potential to proliferate extensively and produce many new osteoblasts, osteoprogenitor cells play a prominent role in bone growth and repair. Internally the very thin endosteum covers small trabeculae of bony matrix that project into the marrow cavities. It also contains osteoprogenitor cells, osteoblasts, and bone lining cells, OSTEOPOROSIS This is frequently found in immobilized patients and in postmenopausal women, is an imbalance in skeletal turnover so that bone resorption exceeds bone formation. This leads to calcium loss from bones and reduced bone mineral density (BMD). Individuals at risk for osteoporosis are routinely tested for BMD by dual-energy x- ray absorptiometry (DEXA scans). TYPES OF BONE LAMELLAR BONE Gross observation of a bone in cross section (Figure 8–7) shows a dense area near the surface corresponding to compact (cortical) bone, which represents 80% of the total bone mass, and deeper areas with numerous interconnecting Short bones such as those of the wrist cavities, called cancellous (trabe and ankle usually have cores of In long bones, the bulbous ends, called epiphyses, cancellous bone surrounded completely are composed of cancellous bone covered by a thin by compact bone. layer of compact cortical bone. The cylindrical part or diaphysis ( a growing between) is almost totally The flat bones that form the calvaria dense compact bone, with a thin region of (skullcap) have two layers of compact cancellous bone on the inner surface around the bone called plates, separated by a central marrow cavity. thicker layer of cancellous bone called the diploë.cular) bone, constituting about 20% of total bone mass. At the microscopic level both compact and cancellous bones typically show two types of organization: mature lamellar bone, with matrix existing as discrete sheets, and woven bone, newly formed with randomly arranged components. Macroscopic photo of a thick section of bone showing the cortical compact bone and the lattice of trabeculae in cancellous bone at the bone’s interior. The small trabeculae that make up highly porous cancellous bone serve as supportive struts, collectively providing considerable strength, without greatly increasing the bone’s weight. The compact bone is normally covered externally with periosteum and all trabecular surfaces of the cancellous bone are covered with endosteum. LAMELLAR BONE LAMELLAR BONE Most bone in adults, compact or cancellous, is organized as lamellar bone, characterized by multiple layers or lamellae of calcified matrix, each 3-7 nm thick. The lamellae are organized as parallel sheets or concentrically around a central canal. In each lamella, type I collagen fibers are aligned, with the pitch of the fibers’ orientation shifted orthogonally (by about 90 degrees) in successive lamellae ). This highly ordered organization of collagen within lamellar bone causes birefringence with polarizing light microscopy; the alternating bright and dark layers are due to the changing orientation of collagen fibers in the lamellae. Like the orientation of wood fibers in plywood the highly ordered, alternating organization of collagen fibers in lamellae adds greatly to the strength of lamellar bone. WOVEN BONE Woven bone is nonlamellar and characterized by random disposition of type I collagen fibers and is the first bone tissue to appear in embryonic development and in fracture repair. Woven bone is usually temporary and is replaced in adults by lamellar bone, except in a very few places in the body, for example, near the sutures of the calvaria and in the insertions of some tendons.In addition to the irregular, interwoven array of collagen fibers, woven bone typically has a lower mineral content (it is more easily penetrated by x rays) and a higher proportion of osteocytes than mature lamellar bone. These features reflect the facts that immature woven bone forms more quickly but has less strength than lamellar bone. OSTEOGENESIS OSTEOGENESIS Bone Development or Osteogenesis The names refer to the mechanisms by occurs by one of the two processes: which the bone forms initially; in both processes woven bone is produced Intramembranous ossification, in first and is soon replaced by stronger which osteoblasts differentiate lamellar bone. During growth of all directly from mesenchyme and bones, areas of woven bone, areas of begin secreting osteoid bone resorption, and areas of lamellar Endochondral ossification, in bone all exist contiguous to one which a preexisting matrix of another. hyaline cartilage is eroded and invaded by osteoblasts, which then begin osteoid production US OSSIFICATION INTRAMEMBRANO Intramembranous ossification, by Within the condensed mesenchyme which most flat bones begin to form, bone formation begins in ossification takes place within condensed sheets centers, areas in which (“membranes”) of embryonic osteoprogenitor cells arise, mesenchymal tissue. Most bones of proliferate, and form incomplete the skull and jaws, as well as the layers of osteoblasts around a network scapula and clavicle, are formed of developing capillaries. Osteoid embryonically by intramembranous secreted by the osteoblasts calcifies ossification. as described earlier, forming small irregular areas of woven bone with osteocytes in lacunae and canaliculi. US OSSIFICATION INTRAMEMBRANO Continued matrix secretion and calcification enlarges these areas and leads to the fusion of neighboring ossification centers. The anatomical bone forms gradually as woven bone matrix is replaced by compact bone that encloses a region of cancellous bone with marrow and larger blood vessels. Mesenchymal regions that do not undergo ossification give rise to the endosteum and the periosteum of the new bone. In cranial flat bones, lamellar bone formation predominates over bone resorption at both the internal and external surfaces. Internal and external plates of compact bone arise, while the central portion (diploë) maintains its cancellous nature. The fontanelles or “soft spots” on the heads of newborn infants are areas of the skull in which the membranous tissue is not yet ossified. RAL OSSIFICATION ENDOCHOND Endochondral (Gr. endon, within + chondros, cartilage) ossification takes place within hyaline cartilage shaped as a small version, or model, of the bone to be formed. This type of ossification forms most bones of the body and is especially well studied in developing long bones, where it consists of the sequence of events shown in Figure 8–14. RAL OSSIFICATION ENDOCHOND Articular cartilage within the joints between long bones (Figure 8–14), which normally persists through adult life The specially organized epiphyseal cartilage (also called the epiphyseal plate or growth plate), which connects each epiphysis to the diaphysis and allows longitudinal bone growth (Figure 8–14) SUMMARRY In summary, longitudinal growth of a bone occurs by cell proliferation in the epiphyseal plate cartilage. At the same time, chondrocytes in the diaphysis side of the plate undergo hypertrophy, their matrix becomes calcified, and the cells die. Osteoblasts lay down a layer of new bone on the calcified cartilage matrix. Because the rates of these two opposing events (proliferation and destruction) are approximately equal, the epiphyseal plate does not change thickness, but is instead displaced away from the center of the diaphysis as the length of the bone increases. Growth in the circumference of long bones does not involve endochondral ossification but occurs through the activity of osteoblasts developing from osteoprogenitor cells in the periosteum by a process of appositional growth which begins with formation of the bone collar on the cartilaginous diaphysis BONE REMODELING AND REPAIR EMODELING BONE R Basic growth involves both the continuous resorption of bone tissue formed earlier and the simultaneous laying down of new bone at a rate exceeding that of bone removal. The sum of osteoblast and osteoclast activities in growing bone constitutes osteogenesis or the process of bone modeling, which maintains the bone’s general shape while increasing its mass. the rate of bone turnover is very active in young children where it can be 200 times faster than adults. in adults, the skeleton is also renewed continuously in a process of bone remodeling which involves the coordinated, localized, cellular activities for bone resorption and formation. EMODELING BONE R The constant remodeling of bone ensures that, despite its hardness, this tissue remains plastic and capable of adapting its internal structure in the face of changing stresses. A well k nown example of bone plasticity is the ability of the positions of teeth in the jawbone to be modified by the lateral pressures produced by orthodontic appliances. Bone forms on the side where traction is applied and is resorbed on the opposite side where pressure is exerted. In this way, teeth are moved within the jaw while the bone is being remodeled EMODELING BONE R Because it contains osteoprogenitor stem cells in the periosteum, endosteum, and marrow and is very well vascu l arized, bone normally has an excellent capacity for repair. Bone repair after a fracture or other damage uses cells, signaling molecules, and processes already active in bone remodeling. Surgically created gaps in bone can be filled with new bone, especially when periosteum is left in place. The major phases that occur typically during bone fracture repair include initial formation of fibrocartilage and its replacement with a tempo r ary callus of woven bone. METABOLIC ROLE OF BONES ROLE OF BONES METABOLIC Calcium ions are required for the activity of many enzymes and many proteins mediating cell adhesion, cytoskeletal movements, exocytosis, membrane permeability, and other cellular functions. The skeleton serves as the calcium reservoir, containing 99% of the body’s total calcium in hydroxyapatite crystals. The concentration of calcium in the blood (9-10 mg/dL) and tissues is generally quite stable because of a continuous interchange between blood calcium and bone calcium. The principal mechanism for raising blood calcium levels is the mobilization of ions from hydroxyapatite to interstitial fluid, primarily in cancellous bone. Ca2+ mobili z ation is regulated mainly by paracrine interactions among bone cells, many of which are not well understood, but two olypeptide hormones also target bone cells to influence cal c ium homeostasis: ID HORMONE PARATHYRO raises low blood calcium levels by stimulating osteoclasts and osteocytes to resorb bone matrix and release Ca2+. The PTH effect on osteoclasts is indirect; PTH receptors occur on osteoblasts, which respond by secreting RANKL and other paracrine factors that stimulate osteoclast formation and activity CALCITOSIN produced within the thyroid gland, can reduce elevated blood calcium levels by opposing the effects of PTH in bone. This hormone directly targets osteoclasts to slow matrix resorption and bone turnover. JOINTS JOINTS Joints are regions where adjacent bones are capped and held together firmly by other connective tissues. The type of joint determines the degree of movement between the bones. Joints classified as synarthroses (Gr. syn, together + arthrosis, artic u lation) allow very limited or no movement and are subdi v ided into fibrous and cartilaginous joints, depending on the type of tissue joining the bones. Major subtypes of synarthro s es include the following: SYNOSTOSES SYNDESMOSES SYMPHYSES involve bones linked join bones by have a thick pad of to other bones and dense connective fibrocartilage allow essentially no tissue only. between the thin movement. In older articular cartilage Examples include adults synosto s es the interosseous covering the ends unite the skull bones, ligament of the of the bones. All which in children and inferior symphyses, such as young adults are held together by sutures, tibiofibular joint the intervertebral or thin layers of and the posterior discs and pubic dense connective symphysis, occur in region of the tissue with the midline of the sacroiliac joints. osteogenic cells. body. JOINTS Intervertebral discs are large symphyses between the articular surfaces of successive bony vertebral bodies. Held in place by ligaments these discoid components of the intervertebral joints cushion the bones and facilitate limited movements of the vertebral column. Each disc has an outer portion, the annulus fibrosus, consisting of concentric fibrocartilage laminae in which collagen bundles are arranged orthogonally in adjacent layers. The multiple lamellae of fibrocartilage produce a disc with unusual toughness able to withstand pressures and torsion generated within the vertebral column. JOINTS Situated in the center of the annulus fibrosus, a gel l ike body called the nucleus pulposus allows each disc to function as a shock absorber (Figure 8–20). The nucleus pulposus consists of a viscous fluid matrix rich in hyaluro n an and type II collagen fibers, but also contains scattered, vacuolated cells derived from the embryonic notochord, the only cells of that structure to persist postnatally. The nucleus pulposus is large in children, but these structures gradu a lly become smaller with age and are partially replaced by fibrocartilage JOINTS Joints classified as diarthroses permit free bone move m ent. Diarthroses (Figure 8–21) such as the elbow and knee generally unite long bones and allow great mobility. In a diar t hrosis ligaments and a capsule of dense connective tissue maintain proper alignment of the bones. The capsule encloses a sealed joint cavity containing a clear, viscous liquid called synovial fluid. The joint cavity is lined, not by epithelium, but by a specialized connective tissue called the synovial mem b rane that extends folds and villi into the joint cavity and pro d uces the lubricant synovial fluid. JOINTS In different diarthrotic joints the synovial membrane may have prominent regions with dense connective tissue or fat. The superficial regions of this tissue however are usually well vascularized, with many porous (fenestrated) capillaries. Besides having cells typical of connective tissue proper and a changing population of leukocytes, this area of a synovial membrane is characterized by two specialized cells with dis t inctly different functions and origins C RO P HA GE -L IK E MA SYN OV IA L C EL L S are derived from blood monocytes and remove wear-and-tear debris from the synovial fluid. These modified macrophages, which represent approximately 25% of the cells lining the synovium, are important in regulating inflammatory events within diarthrotic joints. FIBROBLASTIC SYNOVIAL CELLS produce abundant hyaluronan and smaller amounts of proteoglycans. Much of this material is transported by water from the capillaries into the joint cavity to form the synovial fluid, which lubricates the joint, reducing friction on all internal surfaces, and supplies nutrients and oxygen to the articular cartilage. JOINTS The collagen fibers of the hyaline articular cartilage are disposed as arches with their tops near the exposed surface which, unlike most hyaline cartilage, is not covered by perichondrium (Figure 8–23). This arrangement of collagen helps distribute more evenly the forces generated by pressure on joints. The resilient articular cartilage efficiently absorbs the intermittent mechanical pressures to which many joints are subjected. THAN K Y O U ! !