GPB 3.2 Theory (Fundamentals of Genetics) PDF 2018
Document Details
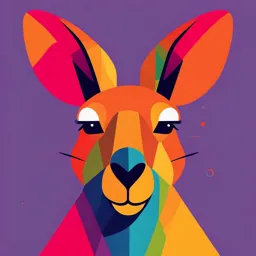
Uploaded by ChivalrousPersonification
Sheth M.C. Polytechnic in Agriculture, Anand Agricultural University, Anand
2018
null
Dr. J. I. Nanavati
Tags
Summary
This document is a set of lecture notes on Fundamentals of Genetics, specifically aimed at agricultural diploma students in their third semester. Included are sections on the history of genetics, different branches of genetics, cell cycle, mitosis, and meiosis. It appears to be a theory course from 2018.
Full Transcript
1 GPB 3.2 (2+1) Fundamentals of Genetics Third Semester (2018 Theory Course) (Course for Agricultural Diploma Students) Prepared by...
1 GPB 3.2 (2+1) Fundamentals of Genetics Third Semester (2018 Theory Course) (Course for Agricultural Diploma Students) Prepared by Dr. J. I. Nanavati Assistant Professor Sheth M.C. Polytechnic in Agriculture Anand Agricultural University Anand Dr. J. I. Nanavati 2 GENETICS Introduction and history of Genetics: Genetics is a biological science which deals with the principles of heredity and variation. In other words, it deals with the inheritance of various characters from parents to their offspring. Since characters are governed by genes, genetics is the study of structure, composition and function of genes. Genetics was born in 1900 when Mendel's result were rediscovered independently by three scientist, viz., Hugo de Vries (Holland), Correns (Germany) and Tschermak (Austria). The term genetics was coined by Bateson in 1905, five year after the birth of this new branch of biology. There are several branches of genetics, viz. plant genetics, microbial genetics, animal genetics, molecular genetics, population genetics, radiation genetics, eugenics, euphonies, mendelian genetics, cytogenetics, quantitative genetics, etc. Various views were prevailing about heredity before rediscovery of Mendel's laws of inheritance. the important pre-memdelian concepts about of inheritance : 1. Preformation theory 2. Theory of epigenesis 3. Theory of acquired characters 4. Theory of pan genes 5. Theory of germplasm After discovery of Mendel's principles of heredity in 1900 significant advancement have been made in genetics and various new concepts have been developed. Notable contribution has been made by various scientists in the advancement of genetics. Various disciplines such as cytology, biochemistry, biophysics and statistics have played important role in the advancement of genetics. genetical concepts have been developed on a variety of organism ranging from viruses to higher organism like flowering used for genetic investigations include bacteria, bacteriophages, Drosophila, Neurospora, corn, garden pea etc. Genetics has important practical application in the field of taxonomy, agriculture, medicine and evolution. In taxonomy, it helps in classification, based on chromosome number. In agriculture, it helps kin the improvement of crop plants and domestic animal, in medicine, it helps in the detection of heredity disease and production of antibiotics. It also helps in evolution by creating new species such as Triticale. Dr. J. I. Nanavati 3 Glossary Genetics:- A biological science which deals with the principles of heredity and variation. It is the study of structure, composition and function of genes. There are various branches of genetics. Plant genetics:- A branch of genetics which deals with inheritance and variation of characters in plant species. Mendelian genetics:- Genetics which deals with the inheritance of oligogenic characters. Cytogenetic:- Combined study of cytology and genetics. Bacteria:- Unicellular free living organism without well defined nucleus. Heredity:- Transmission of characters from parents to their offspring. Variation:- Differences for various characters among the individuals of the same species. Dr. J. I. Nanavati 4 CELL CYCLE Cell cycle can be defined as the entire sequence of events happening from the end of one nuclear division to the beginning of the next. A cell cycle consists of two phases, viz., 1) interphase and 2) the cell division proper. The time required for the completion of cell cycle differs from species to species. Interphase Interphase is generally known as DNA systhesis phase. Interphase consists of G1, S and G2 sub phases. G1 is the resting phase, S is the period of DNA replication and G2 again is a resting stage after DNA replication. G1 Phase: It is a pre-DNA replication phase. Thus, this is a phase between telophase and S phase. This is the longest phase which takes 12 hours in Vicia faba. It is the most variable period of cell cycle. Synthesis of proteins and RNA take place during this phase. S (Synthetic) Phase: This phase comes after G1and takes lesser time than G1phase. In Vicia faba, it takes six hours. The chromosome and DNA replications take place during this phase. G2 Phase: This is the post-DNA replication phase and last sub stage of interphase. This phase also takes 12 hours in Vicia faba. Synthesis of protein and RNA occur during this stage. G1 (12 hours) DNA synthesis DNA synthesis M S 1 (6 hours) ho RNA & ur Protein synthesis G2 (12 hours) RNA & Protein synthesis Periods in a mitotic cell cycle in the root tip of Vicia faba. Interphase includes G1, S and G2 Mitosis consists of prophase, metaphase, anaphase and telophase. Dr. J. I. Nanavati 5 CELL DIVISION All the cells are produced by division of pre-existing cells. Continuity of life depends on cell division. In the cell division, the division of nucleus is calledkaryokinesis and division of cytoplasm is called cytokinesis. The cell division is of two types. 1) Mitosis and 2) Meiosis MITOSIS The term mitosis was coined by Flemming in 1882. Mitosis occurs in somatic organs like root tip, stem tip and leaf base etc. Hence it is also known as somatic cell division. The daughter cells are similar to the mother cell in shape, size and chromosome complement. Since the chromosome number is same in the daughter cells as compared to that of mother cell, this is also known as homotypic or equational division. Mitotic cell cycle includes the following stages: Interphase : This is the period between two successive divisions. Cells in interphase are characterized by deeply stained nucleus that shows a definite number of nucleoli. The chromosomes are not individually distinguishable but appear as extremely thin coiled threads forming a faintly staining network. The cell is quite active metabolically during interphase. Mitosis consist of four stage, viz., (a) Prophase, (b) Metaphase, (c) Anaphase and (d) Telophase a) Prophase: The nucleus takes a dark colour with nuclear specific stains and also with acetocarmine / orcein. The size of the nucleus is comparatively big and the chromosomes that are thin in the initial stages slowly thicken and shorten by a specific process of coiling. The two chromatids of a chromosome are distinct with matrix coating and relational coiling. The disinte gration of nuclear membrane denotes the end of prophase. b) Metaphase: After the disintegration of nuclear membrane, the shorter and thicker chromosomes will spread all over the cytoplasm. Later, the size of the chromosomes is further reduced and thickened. The distinct centromere of each chromosome is connected to the poles through spindle fibres. The chromosomes move towards equator and the centromere of each chromosome is arranged on the equator. This type of orientation of centromeres on the equator is known as autoorientation. The chromosomes at this stage are shortest and thickest. The chromatids of a chromosome are held together at the point of centromeres and the relational coils are at its minimum. c) Anaphase: The centromere of each chromosome separates first and moves to towards the poles. Depending on the position of the centromeres (metacentric, acrocentric and telocentric), the chromosomes show ` V `, ` L ` and ` I ` shapes respectively as the anaphase progresses. The sister chromatids move to the Dr. J. I. Nanavati 6 poles. The chromosome number is constant but the quantity of each chromosome is reduced to half. d) Telophase: Chromosomes loose their identity and become a mass of chromatin. The nucleus will be re-organized from the chromatin. At late telophase stage, the cell plate will divide the cell into two daughter cells. Cytokinesis : The division of cytoplasm usually occurs between late anaphase and end of telophase. In plants, cytokinesis takes place through the formation of cell plate, which begins in the centre of the cell and moves towards the periphery in both sides dividing the cytoplasm into two daughter cells. In animal cells, cytokinesis occurs by a process known as cleavage, forming a cleavage furrow. Significance of Mitosis Mitosis plays an important role in the life of living organisms in various ways as given below: 1. Mitosis is responsible for development of a zygote into adult organism After the fusion of male and female gametes. 2. Mitosis is essential for normal growth and development of living organisms. It gives a definite shape to a specific organism. 3. In plants, mitosis leads to formation of new organs like roots, leaves, stems and branches. It also helps in repairing of damaged parts. 4. It acts as a repair mechanism by replacing the old, decayed and dead cells and thus it helps to overcome ageing of the cells. 5. It helps in asexual propagation of vegetatively propagated crops like sugarcane, banana, sweet potato, potato, etc. mitosis leads to production of identical progeny in such crops. 6. Mitosis is useful in maintaining the purity of types because it leads to production of identical daughter cells and does not allow segregation and recombination to occur. 7. In animals, it helps in continuous replacement of old tissue with new ones, such as gut epithelium and blood cells. Dr. J. I. Nanavati 7 MITOSIS Dr. J. I. Nanavati 8 MEIOSIS The term meiosis was coined by J.B. Farmer in 1905. This type of division is found in organisms in which there is sexual reproduction. The term has been derived from Greek word; Meioum = diminish or reduce. The cells that undergo meiosis are called meiocytes. Three important processes that occur during meiosis are: 1. Pairing of homologous chromosomes (synapsis) 2. Formation of chiasmata and crossing over 3. Segregation of homologous chromosomes The first division of meiosis results in reduction of chromosome number to half and is called reduction division. The first meiotic division is also called heterotypic division. Two haploid cells are produced at the end of first meiotic division and in the second meiotic division, the haploid cells divide mitotically and results in the production of four daughter cells (tetrad), each with haploid number of chromosomes. In a tetrad, two daughter cells will be of parental types and the remaining two will be recombinant types. The second meiotic division is also known as homotypic division. Both the meiotic divisions occur continuously and each includes the usual stages viz., prophase, metaphase, anaphase and telophase. Meiotic cell cycle involves the following stages: Interphase : Meiosis starts after an interphase which is not very different from that of an intermitotic interphase. During the premeiotic interphase DNA duplication occurs during the S phase I. Meiosis-I: (1) Prophase-I: It is of a very long duration and is also very complex. It has been divided into the following sub-stages: a) Leptotene or Leptonema: Chromosomes at this stage appear as long thread like structures that are loosely interwoven. In some species, on these chromosomes, bead-like structures called chromomeres are found all along the length of the chromosomes. b) Zygotene or Zygonema: It is characterized by pairing of homologous chromosomes (synapsis ), which form bivalents. The paired homologous chromosomes are joined by a protein containing frame work known as synaptonemal complex. The bivalents have four strands c) Pachytene or Pachynema: The chromosomes appear as thickened thread- like structures. At this stage, exchange of segments between nonsister chromatids of homologous chromosomes known as crossing over occurs. During crossing over, only one chromatid from each of the two homologous chromosomes takes part. The nucleolus still persists. Dr. J. I. Nanavati 9 d) Diplotene or Diplonema: At this stage further thickening and shortening of chromosomes takes place. Homologous chromosomes start separating from one another. Separation starts at the centromere and travels towards the ends (terminalization). Homologous chromosomes are held together only at certain points along the length. Such points of contact are known as chiasmata and represent the places of crossing over. The process of terminalization is completed at this stage. e) Diakinesis: Chromosomes continue to undergo further contraction. The bivalents appear as round darkly stained bodies and they are evenly distributed throughout the cell. The nuclear membrane and nucleolus disappear. 2) Metaphase-I: The chromosomes are most condensed and have smooth outlines. The centromeres of a bivalent are connected to the poles through the spindle fibres. The bivalents will migrate to the equator before they disperse to the poles. The centromeres of the bivalents are arranged on either side of the equator and this type of orientation is called co-orientation. 3) Anaphase-I: The chromosomes in a bivalent move to opposite poles (disjunction). Each chromosome possess two chromatids. The centromere is the first to move to the pole. Each pole has a haploid number of chromosomes 4) Telophase-I: Nuclear membranes are formed around the groups of chromosomes at the two poles. The nucleus and nucleolus are reorganized. II. Meiosis-II: The second meiotic division is similar to the mitotic division and it includes the following four stages: 1) Prophase-II: The chromosomes condense again. The nucleolus and nuclear membrane disappear. The chromosomes with two chromatids each become short and thick 2) Metaphase -II: Spindle fibres appear and the chromosomes get arranged on the equatorial plane(auto-orientation). This plane is at right angle to the equatorial plane of the first meiotic division. 3) Anaphase-II: Each centromere divides and separates the two chromtids, which move towards the opposite poles. 4) Telophase-II: The chromatids move to the opposite poles The nuclear envelope and the nucleolus reappears. Thus at each pole, there is re - organization of haploid nucleus. Cytokinesis: The division of cytoplasm takes place by cell plate method in plants and by furrow method in animals. The cytokinesis may take place after meiosis I and meiosis II separately or sometimes may take place at the end of meiosis II only. Dr. J. I. Nanavati 10 Significance of Meiosis Meiosis plays a very important role in the biological populations in various ways as given below: 1. It helps in maintaining a definite and constant number of chromosomes in a species. 2. Meiosis results in production of gametes with haploid (half) chromosome number. Union of male and female gametes leads to formation of zygote which receives half chromosome number from male gamete and half from the female gamete and thus the original somatic chromosome number is restored. 3.Meiosis facilitates segregation and independent assortment of chromosomes and genes. 4. It provides an opportunity for the exchange of genes through the process Of crossing over. Recombination of genes results in generation of variability in a biological population which is important from evolution points of view. 5. In sexually reproducing species, meiosis is essential for the continuity of generation. Because meiosis results in the formation of male and female gametes and union of such gametes leads to the development of zygotes and thereby new individual. Dr. J. I. Nanavati 11 MEIOSIS Dr. J. I. Nanavati 12 Differences between mitosis and meiosis Mitosis Meiosis 1 Consists of one nuclear division 1. Consists of two nuclear divisions 2. One cell cycle results in 2. One cell cycle results in production production of two daughter cells of four daughter cells 3. The chromosome number of 3. Daughter cells contain half the daughter cells is the same as chromosome number of mother cell that of mother cell (2n) (n) 4. Daughter cells are identical 4. Daughter cells are different from with mother cell in structure mother cell in chromosome number and chromosome composition and composition 5. It occurs in somatic cells 5. It occurs in reproductive cells 6. Total DNA of nucleus replicates 6. About 0.3% of the DNA is not during S phase replicated during S phase and it occurs during the zygotene stage. 7. The prophase is not divided 7. The prophase I is divided into five into sub stages sub stages 8. There is no pairing between 8. Homologous chromosomes pair homologous chromosomes during pachytene 9. Segregation and recombination 9. Crossing over takes place during do not occur pachytene 10. Chromosomes are in the form 10. Chromosomes are in the of dyad at metaphase form of tetrad at metaphase 11. The centromeres of all the 11. The centromeres of all the chromosomes lie on the chromosomes lie on either side of equatorial plate the equatorial plate (co-orientation) (auto-orientation) during metaphase I during metaphase 12. At metaphase, centromere of 12. The centromere does not each bivalent divides divide at metaphase I longitudinally 13. One member of sister 13. One member of homologous chromatids moves to chromosomes moves to opposite opposite pole during poles during the anaphase I anaphase 14. Maintains purity due to lack 14. Generates variability due to of segregation and segregation and recombination recombination Dr. J. I. Nanavati 13 MENDEL’S LAW OF INHERITANCE INTRODUCTION The first systematic approach for the investigation of inheritance was made by Gregor Johann Mendel, an Austrian monk, in the middle of nineteenth century. His brilliant and systematic ork with garden pea (Pisum sativum) resulted in the invention of the principles of inheritance and laid the foundation of new branch of biology known as genetics. For his pioneer work on the principles of heredity, Mendel is known as the father of genetics. this chapter deals with Mendel's laws of inheritance and related aspects. THRMINOLOGY A brif description of various terms used by Mendel and others related to principles of inheritance is presented below: Hereditary Determinants The entities which are responsible for inheritance of characters from one generation to another are called hereditary determinants of factors. Such factors are now called as genes. Mendel observed that (1) each plant had two factors, one from each parent for each character, and (2) each sex cell has only one determinant or factor. Alleles The various forms of gene are called alleles. Each gene has usually two alleles. The alleles for one character are located on corresponding locus on homologous chromosomes. Thus alleles are the alternative forms of a gene. Gamete Gamete refers to a sexual unit. In other words, sex cells are usually called as gametes. Each sex cell has one member of a gene, i.e., allele. Contrasting characters The individual plant features with marked phenotypic (observable) difference are known as contrasting characters or traits, such as red and white flower colour, yellow and green seed coat colour, tall and dwarf plant stature etc. Dominant and Recessive When a cross is made between plants having contrasting characters, only one character is expressed in the hybrid and the other is suppressed. The character which is expressed is called dominant character and the character which is suppressed is known as recessive character, For example, when we cross between red flowered and white flowered plants, the hybrid has red flower. Here red colour is dominant and white colour is recessive. the dominant character is represented by capital letter and recessive by small letter. For example, red colour is represented by R and white by r. Mendel studies seven Dr. J. I. Nanavati 14 characters in garden pea and recorded dominant and recessive behavior of all seven characters (Table 7.1). The dominant and recessive genes differ from one another in three main aspects as given below: 1. Dominant gene expresses in F1, whereas recessive gene is masked or suppressed in F1. 2. Dominant gene is represented by capital letter, whereas recessive gene is represented by small letter. 3. Dominant character is usually wild type, whereas recessive character is usually mutant type. Table 7.1. Dominant and recessive behavior of seven characters studied by mendel in garden pea character Dominant Recessive Plant stature Tall Dwarf Position of flower Exial Terminal Shape of Pod Inflanted Constricted Colour of Pod Green Yellow Seed shape Round Wrinkled Seed Colour Yellow Green Seed-coat Colour Grey White Gene Symbol In chemistry symbols are used to designate various elements. In genetics, symbols are used to represent various characters. English alphabets or letters are used for assigning symbol to various genetic characters or traits. Mendel also used symbols in his studies with garden pea. Two types of systems for gene symbols are used in genetics. These are (1) capital and small letter system, and (2) plus (+) and small letter system. A brief description of these systems of gene symbol is presented below: Capital and Small Letter System Generally there are two alternative forms of each gene, viz, dominant and recessive. The dominant from of a character is designated with capital letter and recessive from with small letter. The following rules are usually followed in this system. 1. The first letter from the name of dominant character is used to designate that character. For example, T for tall, R for red and Y for yellow. The corresponding small letters are used for the recessive allele viz., t for dwarf, r for white and y for green. 2. Sometimes, one or two other letters from the name of dominant trait are used to designate the dominant gene. For example, Pr for purple. When two or more traits begin with the same letter adoption of this type of system becomes essential. In case of several recessive alleles of the same trait use of one or two Dr. J. I. Nanavati 15 other letters along with first letter of a trait is common to differentiate among different alleles of a gene. 3. In case of multiple alleles a slightly different type of gene symbol is used (see under multiple alleles). Plus and Small Letter System This system of gene symbol is adopted when characters are classified as wild and mutant. The character which is commonly found is wild state in a population is called wild character. The wild type allele is designated with plus (+) symbol. The variant from the wild type is known as mutant character. a mutant gene is usually recessive to the wild type. Suppose tall is the wild character and dwarf is the mutant character, the tall will be designated with +and dwarf with d. Sometimes, the mutant is dominant to the wild type such as bar eye and notch wings in Drosophila. In this case the symbol B and N are assigned to bar eye and notch wings and b and n to their respective wild types. In such situations, the symbols of mutant alleles are used to assign the symbols to wild or normal types. Both these systems of gene symbol are widely used in genetics. However, some persons prefer to use the first system for its simplicity. The use of symbols in genetics is useful in four main ways as given below: Homozygous and Heterozygous Individuals having similar alleles on the corresponding locus of homo- locus chromosomes are known as homozygous. There are four main features of such individuals as given below: 1. They have similar alleles or genes, viz., either AA or aa. 2. Produce only one type of gamete, viz., either A or a. 3. Such individuals are pure breeding and produce same type of individuals on selfing. 4. Have one visible class or individuals on salfing. Individuals which have dissimilar alleles at the corresponding locus of homologous chromosome are called heterozygous. Such individuals have following main feature: 1. Have dissimilar alleles or genes, viz., Aa 2. Produce two types of gametes, viz., A and a for single gene difference. 3. Produce three types of offsprings, viz., AA, Aa and aa on selfing or intermeeting. 4. Have two visible classes, viz., AA/Aa and aa in F2. Pure Line A pure line is a plant which breeds true on selfing, means produces only one type of offspring on self pollination. Mendel developed pure lines for purple and white colour, yellow and green seed colour, round and wrinkled seed shape, etc. Dr. J. I. Nanavati 16 Crossing Artificial pollination between plants having contrasting genes (alleles) for a character is known as crossing and product of such a cross in known is hybrid or F1 (first filial generation). Depending on the gene pairs involved crosses are of three types viz., (1) monohybrid cross, (2) dihybrid cross and (3) polyhydric cross. There are defined below: Monohybrid Cross It refers to a cross involving one gene pair affecting one character. For example, cross between individuals having purple and white coloured flowers or yellow and green seed colour. Such crosses give phenotypic ratio of 3:1 and genotypic ration of 1 : 2 : 1. Dihybrid Cross In such corss two pairs of genes are involved each affecting a different character. Thus when inheritance of two characters is studies simultaneously, the cross involving such characters are called dihybrid cross. For example, cross between plants having yellow and round seed and green and wrinkled seed is called dihybrid cross. Such cross gives a phenotypic ration of 9 : 3 : 3 : 1 and genotypic ratio of 1 : 4 : 6 : 4 : 1. Phenotype and Genotype Phenotype refers to the actual characteristic appearance of an Individual. In other words, it refers to the visual characters such as colour, height, sex, etc. For example, purple and white flower colours are different phenotypes. The main features of phenotype are given below: 1. It refers to visual characters like colour, shape, stature, plant surface etc. 2. Individuals with similar phenotype may be homozygous (AA) as well as heterozygous (Aa), hence they may or may not breed true. 3. For single gene with complete dominance, the observed phenotypic ratio in F2 is 3 : 1. 4. Phenotype classes are always lesser than genotypic classes. Dr. J. I. Nanavati 17 MENDEL'S LAWS OF INHERITANCE Mendel laid the foundation of the science of genetics through the discovery of basic principles of heredity. He conducted his experiments with garden pea (Pisum sativum) in a small monastery garden for over seven years (1856-1864) and discovered two important laws of heredity, viz., (1) law of segregation, and (2) law independent assortment. These are briefly presented below. 1. Law of Segregation This law states that alleles segregate of separate from each other during gamete formation and pass on different gametes in equal number. In other words, when alleles for two contrasting characters come together in a hybrid, they do not blend, contaminate of affect each other while together. The different genes separate from each other in a pure from, pass on to different gametes formed by a hybrid and then go to different individuals in the offspring of the hybrid. Thus main features of this law are as follows: When a dominant and a recessive allele of gene come together in a hybrid after crossing between two plants having contrasting characters, they do not mix or blend together and they remain together in pure from without affecting each other. For this reason, low of segregation is also known as low of purity of gametes. 1. They separate into different gametes in equal number. Each gamete has only one type of allele (say either A or a). 2. Separation of two alleles of a gene during gamete formation takes place usually due to the separation of homologous chromosomes during meiosis (anaphase 1.), because alleles are located in the chromosomes. 3. With complete dominance, segregation leads to phenotypic ratio of 3 : 1 in F2 for characters governed by single gene, and 9 : 3 : 3 : 1 ratio for characters controlled by two genes. 4. If crossing over does not take place, segregation of fgenes takes place during anaphase. I. If crossing over occurs, segregation of genes will take place during anaphase II. Example When we make a cross between red (RR) and white (rr) flowered plants. we get red colour of flower in F1 In the F1 both alleles R and r remain together without blending or mixing with each other, through only the effect of dominat allele is visible. In F2, allele fo red flower colour and white flower colour segregate during gamete formation and pass on to the gametes in equal number. Thus two types of gametes, viz., R and r are formed. Each gamete has eigher R or r allele. When the F1 is self-pollinated, individuals with three genotypes, viz., RR, Dr. J. I. Nanavati 18 Rr and rr are obtained in F2. Here RR and Rr are all red and only rr individuals are white (Fig. 7.1). thus a phenotype ratio of 3 red only red : 1 white is obtained. the overall mechanism is represented below. Parents Red flower White Flower RR X rr I F1 Rr Red gametes F2 R r RR Rr Red Red R R Rr rr r r Red White Fig. 7.1 Segregation of gene for red and white colour. When selfed seeds of RR were grown in F3, they all produced all size true breeding individuals for red flower colour. The Rr individuals showed segregation in F3 similar to segregation in F2 generation, individuals with rr genotypes where found true breeding for white flower colour when their selfed seeds were raised in F3 generation. 2. Law of Independent Assortment This is the second law of inheritance discovered by Mendel. this law states that when two pairs of gene segregating together their assortment occurs randomly and quite freely. Thus main features of this low are given below: 1. This law explains simultaneous inheritance of two plant characters. 2. In F1 when two genes controlling two different characters, come together, each gene exhibits independent dominant behavior without affecting or modifying the effect of other gene. 3. These gene pairs Segregate during gamete formation independently. 4. The alleles of one gene can freely combine with the alleles of another gene. Thus each allele of one gene has an equel chance to combine with each allele of another gene. 5. Each of the two gene pairs when considered separately, exhibits typical 3 :1 segregation ratio in F2 generation. this is a typical dihybrid segregation ratio. Dr. J. I. Nanavati 19 6. Random or free assortment of alleles of two genes leads to formation of new gene combinations. Example When plants of garden pea with yellow round seeds are crossed with plants having green wrinkled seeds, we get yellow round seeds in F1. thus yellow colour of seed exhibits dominance over green and round seeds shape over wrinkled independently. The F1 produces four types of gametes, viz., yellow round (YR), yellow wrinkled (Yr), green round (yR), and green wrinkled (yr). Selfing of F1 gives rise to all above four types of individuals in 9 : 3 : 3 : 1 ratio (fig. 7.2). The behavior of all these genotypes was studies in F3 generation. Out of nine yellow round individuals only one (YYRR) was found true breeding in F3 generation. The other eight individuals showed segregation of various types. Similarly, out of 3 yellow wrinkled individuals only one (YYrr) bred true and others segregated in 3 : 1 ratio. Same thing happened with green round individuals. The green wrinkled individual was also true breeding (Table 7.2) Parents Yellow Round Green Wrinkled YYRR X yyrr I F1 YyRr Yellow round gameters YR Yr yR Yr F2 YR YYRR YYRr YyRR YyRr Yr YYRr YYrr YyRr Yyrr yR YuRR YyRr YYRR YYRr yr YyRr Yyrr yyRr yyrr Dr. J. I. Nanavati 20 Fig. 7.2 independent assortment of two pairs of genes in garden pea. TABLE 7.2 Behavour of different F2 individuals in F3 generation Genotypes No. Phenotypes Segregation in F3 YYRR 1 Yellow round All yellow round YYRr 2 Yellow round 3 yellow round 1 yellow wrinkled YyRR 2 Yellow round 3 yellow round 1 green round YyRr 4 Yellow round 9 : 3 : 3 : 1 like F1 in F2 YYrr 1 Yellow wrinkled All yellow wrinkled Yyrr 2 Yellow wrinkled 3 yellow wrinkled 1 green wrinkled yyRR 1 Green round All green round yyRr 2 Green round 3 green round 1 green wrinkled yyrr 1 Green wrinkled All green wrinkled REASONS FOR MENDEL'S SUCCESS 1. Proper Maintenance of Records Mendel has a systematic record of all the observations which he recorded on various characters in different generations. This helped him in proper understanding of laws responsible for the transmission of character from one generation to other. 2. Study of Individual character Mendel laid emphasis on the study of individual character, which helped him in systematic analysis of various characters. He studied seven characters of garden pea. Other workers studied the individual as a whole which confused the whole issue and they could not come up with concrete results. 3. Maintenance of purity Mendel always used pure breeding parents of lines for hybridization, which helped him in studying the inheritance of individual character in a systematic way. He used to maintain purity by growing different lines in separate plots to avoid mechanical admixture or contamination. Use of heterozygous material poses several difficulties in drawing general conclusions. 4. Knowledge of shortfalls of earlier workers Mendel systematically recorded the reasons for the failure of earlier workers in investigating the mechanism of inheritance. This helped Mendel to think in new direction and better planning of his work. 5. Mathematical Background Mendel had very good background of physics and mathematics. He studies mathematics at the University of Vienna where he was sent for higher studies by the monastery. his mathematical knowledge proved boon for him, which helped Dr. J. I. Nanavati 21 Mendel in explaining the segregation of characters in F2 and F3 generations in terms of segregation ratios. He was able to understand that in natural population, it is very difficult to get segregation perfectly in a particular ratio. A slight deviation from the exact ratio will generally be observed. 6. Proper choice of characters In garden pea, Mendel could easily select contrasting (opposite) form for various characters, which helped him in getting clear-cut groups in F2 and F3 generations. Moreover, all the seven characters which Mendel studied in garden pea, were qualitative in nature. Such characters always display discontinuous variation, which permits classification of individuals into different clear-cut groups for these characters. This also helped Mendel in generalizing his results. Dr. J. I. Nanavati 22 STUDY OF CHROMOSOME STRUCTURE A chromosome is a structure that occurs within cells and that contains the cell's genetic material. That genetic material, which determines how an organism develops, is a molecule of deoxyribonucleic acid (DNA). A molecule of DNA is a very long, coiled structure that contains many identifiable subunits known as genes. In prokaryotes, or cells without a nucleus, the chromosome is merely a circle of DNA. In eukaryotes, or cells with a distinct nucleus, chromosomes are much more complex in structure. Historical background The terms chromosome and gene were used long before biologists really understood what these structures were. When the Austrian monk and biologist Gregor Mendel (1822–1884) developed the basic ideas of heredity, he assumed that genetic traits were somehow transmitted from parents to offspring in some kind of tiny "package." That package was later given the name "gene." When the term was first suggested, no one had any idea as to what a gene might look like. The term was used simply to convey the idea that traits are transmitted from one generation to the next in certain discrete units. The term "chromosome" was first suggested in 1888 by the German anatomist Heinrich Wilhelm Gottfried von Waldeyer-Hartz (1836–1921). Waldeyer-Hartz used the term to describe certain structures that form during the process of cell division (reproduction). One of the greatest breakthroughs in the history of biology occurred in 1953 when American biologist James Watson and English chemist Francis Crick discovered the chemical structure of a class of compounds known as deoxyribonucleic acids (DNA). The Watson and Crick discovery made it possible to express biological concepts (such as the gene) and structures (such as the chromosome) in concrete chemical terms. Dr. J. I. Nanavati 23 According to the classical cytological studies, each chromosome structurally consists of a limiting membrane called pellicle, an amorphous matrix and two very thin, highly coiled filaments called chromonema or chromonemata. Each chromonemata is 800A 0 thick and contains 8- microfibriis, each of which in its turn contains two double helics of DNA. Both chromonematae remain intimately coiled in spiral manner with each other and have a series of microscopically visible bead-like swelling along its length called chromomeres. The early geneticists have attached great significance to the chromomeres and errorneously considered them as hereditary unit, hereditary or Mendelian factors or genes; but modern cytological investigations have confirmed that the chromomeres are not genes but the regions of super-imposed coils. The recent cytological findings have also condemned the view that chromosomes have pellicle, matrix and chromonemata. A. Nucleolus organizer B. Chromosome C. Nucleolus The structure of chromosomes and genes A chromosome is an organized structure of DNA and protein that is found in cells. A chromosome is a single piece of coiled DNA containing many genes, regulatory elements and other nucleotide sequences. Chromosomes also contain DNA-bound proteins, which serve to package the DNA and control its functions. The word chromosome comes from the Greek chroma - color and soma - body due to their property of being very strongly stained by particular dyes. Dr. J. I. Nanavati 24 Chromosomes vary widely between different organisms. The DNA molecule may be circular or linear, and can be composed of 10,000 to 1,000,000,000 nucleotides in a long chain. Typically eukaryotic cells (cells with nuclei) have large linear chromosomes and prokaryotic cells (cells without defined nuclei) have smaller circular chromosomes, although there are many exceptions to this rule. Today we know that a chromosome contains a single molecule of DNA along with several kinds of proteins. A molecule of DNA, in turn, consists of thousands and thousands of subunits, known as nucleotides, joined to each other in very long chains. A single molecule of DNA within a chromosome may be as long as 8.5 centimeters (3.3 inches). To fit within a chromosome, the DNA molecule has to be twisted and folded into a very complex shape. Each chromosome has a constriction point called the centromere, which divides the chromosome into two sections, or “arms.” The short arm of the chromosome is labeled the “p arm.” The long arm of the chromosome is labeled Dr. J. I. Nanavati 25 the “q arm.” The location of the centromere on each chromosome gives the chromosome its characteristic shape, and can be used to help describe the location of specific genes. Furthermore, cells may contain more than one type of chromosome; for example, mitochondria in most eukaryotes and chloroplasts in plants have their own small chromosomes. Dr. J. I. Nanavati 26 MULTIPLE ALLELES Allele is a shorter term than allelomorph (another form) is the alternate form of gene. Many genes have two alternate forms but several other have more than two alternate forms. More than two alleles at the same locus give rise to a multiple allelic series. Multiple alleles can be defined as a series of forms of a gene situated at the same locus of homologous chromosomes. According to Mendel, each gene had two alternate forms or allele morphs are being dominant and the other being recessive. Dominant being the wild type from which recessive mutant was evolved through mutation. Likewise, a wild type can mutate in many ways and produce many mutant forms and a mutant can again undergo another mutation and give rise to a new mutant. Hence, a gene can exist in more than two allelomorphs. Usually wild type allele is dominant over its recessive allele. wild allele is represented as +. Multiple alleles can be defined as a series of forms of a gene situated at the same locus of homologous chromosomes affecting same character. Multiple alleles are different forms of the same gene that is the sequence of the bases is slightly different in the genes located on the same place of the chromosome. Multiple alleles are alternative states at the same locus. Remember: each individual will only have two alleles for a trait but there are several alleles to choose from.) The classical example for multiple alleles is human blood group self incompatibility in tobacco, coat colour in rabbit, self incompatability genes Dr. J. I. Nanavati 27 in brassica. Important features of multiple alleles 1) Multiple alleles always belong to the same locus and one allele is present at a locus at a time in a chromosome 2) Multiple alleles always control the same character of an individual 3) Wild type allele is dominant over other alleles 4) There is no crossing over in the multiple alleles 5) In a series of mutiple alleles wild type is always dominant 6) When two mutant types are crossed wild form cannot be recovered 7) The cross between two mutant alleles will always produce mutant phenotype. Examples of multiple alleles are 1) fur colour in a rabbit, 2) ABO blood group in man 3) Wing type in drosophila 4) Eye colour in drosophila etc. Fur colour in Rabbit. In rabbit, three alternate forms of genes, which controls coat colour. C causes wild type and its alleles. ABO Blood group in man. Antibody Antibody is a type of protein, which is commonly referred to as immunoglobin. It is usually found in the serum or plasma. The presence of antibody can be demonstrated by its specific reaction with an antigen. Antigen An antigen refers to an substance or agent, which when introduced into the system of vertebrate animal like cow, goat, man etc induces the production of specific antibody, which binds specifically to this (Antigen) substance Antigen are located in the red blood corpuscles (RBC). If a person has a particular antigen in his RBCs, his serum has usually antibodies against the other antigen. Dr. J. I. Nanavati 28 In human RBC two types of antigens viz A and B are present. Depending upon the presence or absence of antigen A and B the blood group in man is of four types viz A, B, AB and O. A person with blood group A has antigen A on the surface of RBCs: protein with blood group B will have antigen B those with blood group AB have antigens A and B; and those with blood group O have no antigen on the surface of their RBCs. Bloo Genotype Antige Antibo Compatib d n dy le blood Grou found presen group p t A IAIA, IAIA A B A and O B IBIB, IbIb B A B and O AB IAIB AB None A,B, AB,O O ii None AB O Recent studies shows that antigen is galactosamine and B is galactose Antibodies A, B, AB and None and are naturally present in the serum of individuals having A,B,AB, and O blood group respectively. The agglutination or coagulation of RBCs leads to clotting of blood due to interaction between antigen antibody. The blood group B cannot be transferred to an individual having blood group A because the recipient has antibody against antigen B which is present on the RBCs of blood group B. Similarly the reverse transfusion is not possible. The blood group AB does not have antibody A and B. Hence individuals with AB blood group can accept all types of blood, viz., A, B, AB and O. Such individuals are known as universal acceptors or recipients. The O blood group does not have any antigen and has antibody against antigen A and B, It cannot accept blood group other than O. Individuals with blood group O are known as universal donors, because transfusion of blood group O is possible with all the four blood types. The consideration of Rh (rhesus) type is important in blood transfusion. Each blood Dr. J. I. Nanavati 29 group has generally two types of Rh group, viz positive and negative. The same type of Rh is compatible for blood transfusion Opposite type lead to reaction resulting in death of the recipient. These are few examples of multiple alleles Now it is believed that multiple alleles are present almost for all genes. Pleiotropism In general one gene affects a single character. But many genes are known to affect more than one character such genes are known as pleiotropic genes and the condition is termed as pleiotrophy. An example of a pleiotropic gene in human beings is the recessive gene s which produces sickle cell anemia in the ss homozygotes. These gene causes changes in two or more parts of characters, which are not related, then the gene is said to be pleiotropic gene. Pseudoalleles The genes that are so closely linked can be separatable only by rare crossing over. Such genes are called pseudoalleles. Dr. J. I. Nanavati 30 LINKAGE Sutton and Boveri proposed the chromosome theory of inheritance. According to chromosome theory of inheritance, the tendency of genes to remain together in their original combination during inheritance is called linkage. Types of Linkage: Linkage is generally classified on the basis of three criteria viz., (i) Crossing over, (ii) Genes involved and (iii) Chromosomes involved (i) Based on crossing over: Linkage may be classified into (a) complete and (b) incomplete / partial depending up on absence or presence of recombinant phenotypes in test cross progeny. (a) Complete linkage: It is known in case of males of Drosophila and females of silkworms, where there is complete absence of recombinant types due to absence of crossing over. (b) Incomplete / partial linkage: If some frequency of crossing over also occurs between the linked genes, it is known as incomplete / partial linkage. Recombinant types are also observed besides parental combinations in the test cross progeny. Incomplete linkage has been observed in maize, pea, Drosophila female and several other organisms. (ii) Based on genes involved : Depending on whether all dominant or some dominant and some recessive alleles are linked together, linkage can be categorized into (a) Coupling phase and (b) Repulsion phase (a) Coupling phase: All dominant alleles are present on the same chromosome or all recessive alleles are present on same chromosome. TR tr --- --- Coupling phase TR tr (b) Repulsion phase: Dominant alleles of some genes are linked with recessive alleles of other genes on same chromosome. Tr tR ---- --- Repulsion phase Tr tR (iii) Based on chromosomes involved: Based on the location of genes on the chromosomes, linkage can be categorized into (a) autosomal linkage and (b) X-chromosomal linkage / allosomal linkage / sex linkage (a) Autosomal linkage: It refers to linkage of those genes which are located in autosomes (other than sex chromosomes). (b) X-chromosomal linkage / allosomal linkage / sex linkage: It refers To linkage of genes which are located in sex chromosomes i.e. either ‘X’ or ‘Y’ (generally ‘X’) Dr. J. I. Nanavati 31 Characteristic features of Linkage: 1. Linkage involves two or more genes which are located in same Chromosome in a linear fashion. 2. Linkage reduces variability. 3. Linkage may involve either dominant or recessive alleles (coupling phase) or some dominant and some recessive alleles (repulsion phase). 5. It may involve either all desirable traits or all undesirable traits or some desirable and some undesirable traits. 6. It is observed for oligo-genic traits as well as polygenic traits. 7. Linkage usually involves those genes which are located close to each other. 8. The strength of linkage depends on the distance between the linked genes. Lesser the distance, higher the strength and vice versa. 9. Presence of linkage leads to higher frequency of parental types than recombinants in test cross. When two genes are linked the segregation ratio of dihybrid test cross progeny deviates significantly from 1:1:1:1 ratio. 10. Linkage can be determined from test cross progeny data. 11. If crossing over does not occur, all genes located on one chromosome Are expected to be inherited together. Thus the maximum number of Linkage groups possible in an organism is equal to the haploid chromosome number. Eg. Onion 2n = 16 n = 8 maximum linkage groups possible = 8 Maize 2n = 20 n = 10 maximum linkage groups possible = 10 12. Linkage can be broken by repeated intermating of randomly selected plants in segregating population for several generations or by mutagenic treatment. 13. Besides pleiotropy, linkage is an important cause of genetic correlation between various plant characters. Dr. J. I. Nanavati 32 RECOMBINATION The term crossing over was first used by Morgan and Cattell in 1912. The hange of precisely homologous segments between non-sister chromatids of homologous chromosomes is called crossing over. Mechanism of crossing over: It is responsible for recombination between linked genes and takes place during pachytene stage of meiosis i.e. after the homologous chromosomes have undergone pairing and before they begin to separate. It occurs through the process of breakage and reunion of chromatids. During pachytene, each chromosome of a bivalent (chromosome pair) has two chromatids so that each bivalent has four chromatids or strands (four-strand stage). Generally one chromatid from each of the two homologues of a bivalent is involved in crossing over. In this process, a segment of one of the chromatids becomes attached in place of the homologous segment of the nonsister chromatid and vice-versa. It is assumed that breaks occur at precisely homologous points in the two nonsister chromatids involved in crossing over; this is followed by reunion of the acentric segments. This produces a cross (x) like figure at the point of exchange of the chromatid segments. This figure is called chiasma (which is seen in diplotene stage of meiosis) (plural-chiasmata). The crossover chromatids will have new combinations of the linked genes, i.e. will be recombinant; Similarly, the noncrossover chromatids will contain the parental gene combinations and the gametes carrying them will give rise to the parental phenotypes or noncrossover types. Therefore the frequency of crossing over between two genes can be estimated as the frequency of recombinant progeny from a test-cross for these genes. Types of crossing over: Depending upon the number of chiasmata involved, crossing over is of three types. 1. Single crossing over: It refers to the formation of single chiasma between non-sister chromatids of homologous chromosomes. It involves two linked genes (Two point test cross). 2. Double crossing over: It refers to the formation of two chiasmata between non-sister chromatids of homologous chromosomes. It involves three linked genes (Three point test cross). 3. Multiple crossing over: Occurrence of more than two crossing overs between non-sister chromatids of homologous chromosomes is known as multiple crossing over. However, the frequency of such type of crossing over is extremely low. Dr. J. I. Nanavati 33 MUTATIONS Mutation in a broad sense include all those heritable changes which alter the phenotype of an individual. Thus mutation can be defined as a sudden heritable change in the character of an organism which is not due to either segregation or recombination. Ø The term mutation was first used by Hugo de Vries to describe the Sudden phenotypic changes which were heritable, while working with Oenothera lamarckiana. Ø But the earliest record of mutations dates back to 1791 when Seth Wright noticed a male lamb with unusually short legs in his flock of sheep. This lamb served as a source of short leg trait for the development of Ancon breed of sheep. Ø However the systematic studies on mutations were started in 1910 by T.H. Morgan who used Drosophila melanogaster for his studies. Ø In 1927, H.J. Muller demonstrated for the first time the artificial induction Of mutations by using x-rays in Drosophila. Ø Similarly in 1928, L.J. Stadler demonstrated an increase in the rate of mutations due to x-rays in barley and maize. Ø Induction of mutations by chemicals in fungus Aspergillus was demonstrated by R.A. Steinberg in 1939. Ø C. Auerbach and J.N. Robson in 1946 used chemicals to induce mutations In Drosophila. Ø The first plant breeding programme using mutations (mutation breeding) was initiated in 1929 in Sweden, Germany and Russia. Ø In India it was initiated in early 1930s. Terminology Muton: The smallest unit of gene capable of undergoing mutation and it is represented by a nucleotide. Mutant: An organism or cell showing a mutant phenotype due to mutant allele of a gene. Mutagen: A physical or chemical agent which induces mutation. Gene mutations or point mutations: The changes which alter the chemical structure of a gene at molecular level. Classification of mutations: Mutations can be classified in several ways. 1. Based on direction of mutations : a) Forward mutation : Any change from wild type allele to mutant allele b) Backward mutation or reverse mutation: A change from mutant allele to wild type 2. Based on source / cause of mutations : a) Spontaneous mutation: Mutation that occur naturally Dr. J. I. Nanavati 34 b) Induced mutation: Mutation that originates in response to mutagenic treatment 3. Based on tissue of origin : a) Somatic mutation: A mutation in somatic tissue b) Germinal mutation: A mutation in germline cells or in reproductive tissues 4. Based on effect on survival : a) Lethal mutation: Mutation which kills the individual that carries it. (survival 0%) b) Sub-lethal mutation: When mortality is more than 50% of individuals that carry mutation c) Sub-vital mutation: When mortality is less than 50% of individual that carry mutation. d) Vital mutation: When all the mutant individuals survive (survival-100%) 5. Based on trait or character effected : a) Morphological mutation: A mutation that alters the morphological features of an individual b) Biochemical mutation: A mutation that alters the biochemical function of an individual. 6. Based on visibility or quantum of morphological effect produced : a) Macro-mutations: Produce a distinct morphological change in phenotype (which can be detected easily with out any confusion due to environmental effects) Generally found in qualitative characters. Eg : colour of flowers, height of plant etc. b) Micro-mutations: Mutations with invisible phenotypic changes, (which can be easily confused with effects produced due to environment). Generally observed in quantitative characters. 7. Based on the site of mutation or on cytological basis : a) Chromosomal mutations: Mutations associated with detectable changes in either chromosome number or structure. b) Gene or point mutations: Mutations produced by alterations in base sequences of concerned genes. c) Cytoplasmic mutations: Mutations associated with the changes in chloroplast DNA (cpDNA) and mitochondrial DNA (mtDNA). Dr. J. I. Nanavati 35 Characteristic features of mutations: 1. Mutations are mostly recessive and very rarely dominant. 2. Most mutations have harmful effects and very few (less than 0.1 %) are beneficial. 3. Mutations may be due to a change in a gene, a group of genes or in entire chromosome. 4. If gene mutations are not lethal, the mutant individuals may survive. However, chromosomal mutations are generally lethal and such mutants do not survive. 6. If mutation occur at both loci simultaneously, the mutants can be Identified M1 generation. However, if it is restricted to one locus only, (dominant to recessive) the effect can be seen only in M2 generation. 6. Macro-mutations are visible and can be easily identified, while micro - mutations can not be seen with naked eye and need special statistical tests (or statistical analysis). 7. Many of the mutants show sterility. 8. Most mutants are of negative selection value. 9. Mutation for altogether new character generally does not occur. 10. Mutations are random i.e. they can occur in any tissue or cell of an organism. However some genes show higher mutation rate than others. 11. Mutations can be sectorial. The branches arising from mutated sector show mutant characters. 12. Mutations are recurrent i.e. the same mutation may occur again and again. 13. Induced mutations commonly show pleiotropy often due mutation in closely linked genes. Dr. J. I. Nanavati 36 QUALITATIVE AND QUANTITATIVE INHERITANCE The inheritance pattern of various characters of living creatures can be described in two ways Qualitative inheritance The inheritance pattern which is governed by few major genes (one or two gene pair) has clear cut phenotypic expression can be termed as qualitative inheritance and the characters show such inheritance are termed as qualitative characters.Following characters can be considered as qualitative characters. 1. Seed colour : white/brown 2. Flower colour: red/white 3. Disease reaction: resistance/susceptible 4. Seed shape: round/wrinkled Quantitative inheritance The inheritance pattern which is governed by many genes (polygenic) having continuous additive and discriminate phenotypic expression can be termed quantitative inheritance and the characters show such inheritance are termed as quantitative characters. Following characters can be considered as quantitative characters and often referred as metric traits as the effects can be measured in cm, kg,gms. 1.Grain yield, 2. Fodder yield, 3. Oil content, 4. Milk production The major differences between the two are following: Qualitative Inheritance Quantitative Inheritance It deals with the inheritance of traits of It deals with the inheritance of traits of kind, viz., form, structure, colour, etc. degree, viz., yield, length, weight, number, etc. Discrete phenotypic classes occur which A spectrum of phenotypic classes occur display discontinuous variations which contain continuous variations. Each qualitative trait is governed by two Each quantitative trait is governed by or many alleles of a single gene. many non-allelic genes or polygenes. The phenotypic expression of a gene is not Environmental conditions effect the influenced by environment. phenotypic expression of polygenes variously. It concerns with individual matings and It concerns with a population of their progeny. organisms consisting of all possible kinds of matings. In it analysis is made by counts and ratios. In it analysis is made by statistical method Dr. J. I. Nanavati 37 MULTIPLE FACTOR HYPOTHESIS Multiple factor It is quite natural that small differences exist among individuals of similar genotype due to the effect of environment on genotype. On the other hand, there are some heritable differences also exist with continuous variation. Most of the economical traits show continuous variation and they are measurable or quantifiable. Quantitative characters Quantitative characters are traits which show continuous variation and governed by a large number of genes called multiple genes or multiple factors or polymeric genes or polygenes. Their inheritance follows same mendelian principles. Quantitative characters Qualitative characters show ▪ discontinuous variation and ▪ are governed by one or two major genes or oligognes. Multiple factor Hypothesis (Nilson – Ehle) Nilson-Ehle studied Kernel colour in wheat concluded that is a quantitative character He crossed true breeding red kernel whet (RR) with true breeding white (rr) and the F1 was red (Rr) and the F2 segregated for red and white in 3:1 ratio indicating the dominance of red over white. However, careful examination indicated the variation in red color among the red color progenies. Dr. J. I. Nanavati 38 F1 red was not as intense as one of the parents. In F2 he could observe two grades of red ie. one was red as that of one of its parent, two were higher red as that of F1 individuals. In some crosses, a ratio of 15 red : 1 white was found in F2 indicating that there are two pairs of genes for red colour that either or both of these can produce red kernels. Finally he observed different shades of red in F2 for red kernel types. The F2 showed red shades and white as follows; Dark red : 1 Medium dark red : 4 Medium red : 6 15 Light red : 4 White : 1 Total : 16 ▪ It was concluded two duplicate dominant alleles R1 and R2 cumulatively decide the intensity of red colour ▪ and both R1 and R2 are in completely dominant over white. ▪ The high intensity of red colour depends on the number. The F2 ratio in wheat Genotype Genotypic ratio Phenotype R1R1 R2R2 1 Dark red R1R1 R2r2 2 Medium dark red R1r1 R2R2 2 Medium dark red R1r1 R2r2 4 Medium red R1R1 r2r2 1 Medium red r1r1 R2R2 1 Medium red R1r1 r2r2 2 light red r1r1 R2r2 2 light red r1r2 r2r2 1 white Hence, if two parents differ for the two genes the segregation was 1:4:6:4:1 provided both R1 and R2 contribute equally to the colour. If three genes are involved in F2 segregation showed 1:6:15:20:15:6:1 for red shades and 1 for white. Dr. J. I. Nanavati 39 Thus, Nilson-Ehle’s multiple factor states that i) For a given quantitative trait there could be several genes, which were independent in their segregation, but had cumulative effect on phenotype ii) Dominance is usually incomplete iii) Each gene contributes something to the strength of expression of character whereas its recessive allele does not of genes present dominance gene. Dr. J. I. Nanavati 40 CYTOPLASMIC INHERITANCE Inheritance of most of the characters in eukaryotic organisms shows the following characteristic features. 1. The contributions by both male and female parents are equal so that the results from reciprocal crosses are identical. 2. Segregation produces the characteristic 3:1 ratio in the F 2 generation of a monohybrid cross and a typical 9:3:3:1in dihybrid crosses. These features of inheritance were first demonstrated by Mendel: consequently, such an inheritance pattern is referred to as Mendelian inheritance. It is universally accepted that genes showing Mendelian inheritance are located in the chromosomes of eukaryotic nuclei. Therefore Mendelian inheritance pattern is regarded as a sufficient evidence for a gene to be located in the chromosomes, such genes are termed as nuclear genes or more commonly simply as genes. Non Mendelian Inheritance But some characters in several organisms do not show Mendelian inheritance or they show a non Mendelian inheritance pattern. In such cases, the following characteristic features are observed. 1. There is consistent difference between the results from reciprocal crosses; generally only the trait from female parent is transmitted. 2. In most cases, there is no segregation in the F2 and subsequent generations. Characters showing non Mendelian inheritance may be grouped under three broad categories: (1) those related to cellular structures and patterns, (2) those produced by intracellular parasites, symbionts and viruses (3) those associated with DNA containing cell organelles viz., mitochondria and chloroplasts. Dr. J. I. Nanavati 41 In addition to these cases of non Mendelian inheritance, some characters in several organisms exhibit a Mendelian inheritance pattern but the development of these characters in an individual is markedly affected by the genotype of the maternal parent of the concerned individual; such cases are classified as maternal effects. The evidence for cytoplasmic inheritance was first presented by Correns in Mirabilis jalapa and by Baur in Pelargonium zonale in 1908. In case of cytoplasmic inheritance generally the character of only one of the two parents ( usually the female parent) is transmitted to the progeny. As a result, reciprocal crosses exhibit consistent differences for such characaters and there is a lack of segregation in the F2 and the subsequent generations. Such inheritance is also referred as extra nuclear inheritance, extrachromosomal inheritance and maternal inheritance. Genes governing the traits showing cytoplasmic inheritance are located outside the nucleus and in the cytoplasm; hence they are referred to as plasma genes, cytoplasmic genes, cytogenes, extranuclear genes or extra chromosomal genes. The sum total of all the genes present in the cytoplasm of a cell is known as Plasmon, while all the genes present in a plastid constitute a plastron. Characteristics of cytoplasmic inheritance: 1. Reciprocal differences: Reciprocal crosses show marked differences for the characters governed by plasmagenes. In most cases, plasmagenes from only one parent, generally the female parent are transmitted, this phenomenon is known as uniparental inheritance. 2. Lack of segregation: In general, F2 F3 and the subsequent generations do not show segregation for a cytoplasmically inherited trait. This is because the f1 individuals generally receive plasma genes from one parent only. Dr. J. I. Nanavati 42 3. Irregular segregation in biparental inheritance: In some cases, plasma genes from both the parents are transmitted to the progeny, this is known as biparental inheritance. 4. Somatic segregation: Plasma genes generally show somatic segregation during mitosis, a feature of rare occurrence in the case of nuclear genes. 5. Association with organelle DNA: Several plasma genes have been shown to be associated with cp-DNA or mt-DNA. 6. Nuclear transplantation: If nuclear transplantation revealas a trait to be governed by the genotype of cytoplasm and not by that of nucleus, cytoplasmic inheritance of the trait is strongly indicated. In nuclear transplantation, nucleus of a cell is removed and replaced by a nucleus of another genotype from a different cell. Generally nuclei of somatic cells are transplanted into zygotes before the first mitotic division is initiated. 7. Transfer of nuclear genome through back crosses: The nucleus of a variety or species may be transferred into the cytoplasm of another species or variety through repeated back crossing with the former, which is used as the recurrent male parent. Lines produced in this way are known as alloplasmic lines since they have nuclei and cytoplasms from two different species. A comparison of the various characters of alloplasmic lines with those of the corresponding euplasmic line (lines having nuclei and cytoplasms from the same species) demonstrates cytopalsmic effects, if any on these traits. This technique is time consuming, but extremely powerful; it has been extensively used to study the cytopalsmic differentiation during evolution. 8. Mutagenesis: Some mutagens eg: Ethidium bromide are highly specific mutagens for plasma genes while nuclear genes are not affected by them.Induction of mutation by such agenets in a gene indicates it to be a plasma gene. 9. Lack of chromosomal location: In many organism, extensive linkage maps of nuclear genes are available. If a gene is shown to be located in one of Dr. J. I. Nanavati 43 these linkage groups, it cannot be a plasma gene. Failure to demonstrate the location of a gene in one of the linkage groups of an organism is indicative of its cytoplasmic location, but this is highly tentative. 10. Lack of association with a parasite, symbiont or virus: In many cases, a cytoplasmically inherited character is associated with a parasite, symbiont or virus present in the cytoplasm of the organism. Such cases cannot be regarded as cases of cytoplamic inheritance. Only those cytoplasmically inherited characters which are not associated with parasites, symbionts or viruses can be regarded as governed by plasma genes. The known cases of true cytoplasmic inheritance are concerned with either choloroplast or mitochondrial traits and are usually associated with their DNA. Such cases are therefore often referred to as organellar inheritance, plastid inheritance and mitochondrial inheritance. Dr. J. I. Nanavati 44 DNA AND IT’S STRUCTURE The discovery that DNA is the prime genetic molecule, carrying all the hereditary information within chromosomes, immediately had its attention focused on its structure. It was hoped that knowledge of the structure would reveal how DNA carries the genetic messages that are replicated when chromosomes divide to produce two identical copies of themselves. During the late 1940s and early 1950s, several research groups in the United States and in Europe engaged in serious efforts— both cooperative and rival—to understand how the atoms of DNA are linked together by covalent bonds and how the resulting molecules are arranged in three-dimensional space. Not surprisingly, it was feared that DNA might have very complicated and perhaps bizarre structures that differed radically from one gene to another. Great relief, if not general elation, was thus expressed when the fundamental DNA structure was found to be the double helix. It told us that all genes have roughly the same three-dimensional form and that the differences between two genes reside in the order and number of their four nucleotide building blocks along the complementary strands. What is DNA? The work of many scientists paved the way for the exploration of DNA. Way back in 1868, almost a century before the Nobel Prize was awarded to Watson, Crick and Wilkins, a young Swiss physician named Friedrich Miescher, isolated something no one had ever seen before from the nuclei of cells. He called the compound "nuclein." This is today called nucleic acid, the "NA" in DNA (deoxyribo-nucleic-acid) and RNA (ribo- nucleic-acid). Two years earlier, the Czech monk Gregor Mendel, had finished a series of experiments with peas. His observations turned out to be closely connected to the finding of nuclein. Mendel was able to show that certain traits in the peas, such as their shape or colour, were inherited in different packages. Dr. J. I. Nanavati 45 These packages are what we now call genes. For a long time the connection between nucleic acid and genes was not known. But in 1944 the American scientist Oswald Avery managed to transfer the ability to cause disease from one strain of bacteria to another. But not only that: the previously harmless bacteria could also pass the trait along to the next generation. What Avery had moved was nucleic acid. This proved that genes were made up of nucleic acid. Solving the Puzzle In the late 1940's, the members of the scientific community were aware that DNA was most likely the molecule of life, even though many were skeptical since it was so "simple". They also knew that DNA included different amounts of the four bases adenine, thymine, guanine and cytosine (usually abbreviated A, T, G and C), but nobody had the slightest idea of what the molecule might look like. In order to solve the elusive structure of DNA, a couple of distinct pieces of information needed to be put together. One was that the phosphate backbone was on the outside with bases on the inside; another that the molecule was a double helix. It was also important to figure out that the two strands run in opposite directions and that the molecule had a specific base pairing. Watson and Crick In 1951, the then 23-year old biologist James Watson travelled from the United States to work with Francis Crick, an English physicist at the University of Cambridge. Crick was already using the process of X-ray crystallography to study the structure of protein molecules. Together, Watson and Crick used X-ray crystallography data, produced by Rosalind Franklin and Maurice Wilkins at King's College in London, to decipher DNA's structure. This is what they already knew from the work of many scientists, about the Dr. J. I. Nanavati 46 DNA molecule: 1. DNA is made up of subunits which scientists called nucleotides. 2. Each nucleotide is made up of a sugar, a phosphate and a base. 3. There are 4 different bases in a DNA molecule: adenine (a purine) cytosine (a pyrimidine) guanine (a purine) thymine (a pyrimidine) 4. The number of purine bases equals the number of pyrimidine bases 5. The number of adenine bases equals the number of thymine bases 6. The number of guanine bases equals the number of cytosine bases 7. The basic structure of the DNA molecule is helical, with the bases being stacked on top of each other Components of DNA DNA is a polymer. The monomer units of DNA are nucleotides, and the polymer is known as a "polynucleotide". Each nucleotide consists of a 5- carbon sugar (deoxyribose), a nitrogen containing base attached to the sugar, and a phosphate group. There are four different types of nucleotides found in DNA, differing only in the nitrogenous base. The four nucleotides are given one letter abbreviations as shorthand for the four bases. A is for adenine G is for guanine C is for cytosine T is for thymine Dr. J. I. Nanavati 47 Purine Bases Adenine and guanine are purines. Purines are the larger of the two types of bases found in DNA. Structures are shown below: The 9 atoms that make up the fused rings (5 carbon, 4 nitrogen) are numbered 1-9. All ring atoms lie in the same plane. Pyrimidine Bases Cytosine and thymine are pyrimidines. The 6 atoms (4 carbon, 2 nitrogen) are numbered 1-6. Like purines, all pyrimidine ring atoms lie in the same plane. Dr. J. I. Nanavati 48 Deoxyribose Sugar The deoxyribose sugar of the DNA backbone has 5 carbons and 3 oxygens. The carbon atoms are numbered 1', 2', 3', 4', and 5' to distinguish from the numbering of the atoms of the purine and pyrmidine rings. The hydroxyl groups on the 5'- and 3'- carbons link to the phosphate groups to form the DNA backbone. Deoxyribose lacks an hydroxyl group at the 2'-position when compared to ribose, the sugar component of RNA. Nucleosides A nucleoside is one of the four DNA bases covalently attached to the C1' position of a sugar. The sugar in deoxynucleosides is 2'- deoxyribose. The sugar in ribonucleosides is ribose. Nucleosides differ from nucleotides in that they lack phosphate groups. The four different nucleosides of DNA are deoxyadenosine (dA), deoxyguanosine (dG), deoxycytosine (dC), and (deoxy)thymidine (dT, or T). Dr. J. I. Nanavati 49 In dA and dG, there is an "N-glycoside" bond between the sugar C1' and N9 of the purine. Nucleotides A nucleotide is a nucleoside with one or more phosphate groups covalently attached to the 3'- and/or 5'-hydroxyl group(s). DNA Backbone The DNA backbone is a polymer with an alternating sugar- phosphate sequence. The deoxyribose sugars are joined at both the 3'- hydroxyl and 5'- hydroxyl groups to phosphate groups in ester links, also known as "phosphodiester" bonds. Example of DNA Backbone: 5'-d (CGAAT) Dr. J. I. Nanavati 50 Features of the 5'-d(CGAAT) structure: Alternating backbone of deoxyribose and phosphodiester groups Chain has a direction (known as polarity), 5'- to 3'- from top to bottom Oxygens (red atoms) of phosphates are polar and negatively charged A, G, C, and T bases can extend away from chain, and stack atop each other Bases are hydrophobic DNA Double Helix DNA is a normally double stranded macromolecule. Two polynucleotide chains, held together by weak thermodynamic forces, form a DNA molecule. Structure of DNA Double Helix Dr. J. I. Nanavati 51 Features of the DNA Double Helix Two DNA strands form a helical spiral, winding around a helix axis in a right-handed spiral The two polynucleotide chains run in opposite directions The sugar-phosphate backbones of the two DNA strands wind around the helix axis like the railing of a sprial staircase The bases of the individual nucleotides are on the inside of the helix, stacked on top of each other like the steps of a spiral staircase. Dr. J. I. Nanavati 52 The Double Helix The double helix of DNA has these features: It contains two polynucleotide strands wound around each other. The backbone of each consists of alternating deoxyribose and phosphate groups. The phosphate group bonded to the 5' carbon atom of one deoxyribose is covalently bonded to the 3' carbon of the next. The two strands are "antiparallel"; that is, one strand runs 5′ to 3′ while the other runs 3′ to 5′. The DNA strands are assembled in the 5′ to 3′ direction and, by convention, we "read" them the same way. The purine or pyrimidine attached to each deoxyribose projects in toward the axis of the helix. Each base forms hydrogen bonds with the one directly opposite it, forming base pairs (also called nucleotide pairs). Å separate the planes in which adjacent base pairs are located. The double helix makes a complete turn in just over 10 nucleotide pairs, so each turn takes a little more (35.7 Å to be exact) than the 34 Å shown in the diagram. There is an average of 25 hydrogen bonds within each complete turn of the double helix providing a stability of binding about as strong as what a covalent bond would provide. The diameter of the helix is 20 Å. The helix can be virtually any length; when fully stretched, some DNA molecules are as much as 5 cm (2 inches!) long. The path taken by the two backbones forms a major (wider) groove (from "34 A" to the top of the arrow) and a minor (narrower) groove (the one below). Dr. J. I. Nanavati 53 TRANSCRIPTION – TRANSLATION - GENETIC CODE AND OUTLINE OF PROTEIN SYNTHESIS Central Dogma of Protein Synthesis Proteins constitute the major part by dry weight of an actively growing cell. They are widely distributed in living matter. All enzymes are proteins. Proteins are built up from about 20 amino acids which constitute the basic building blocks. In proteins the amino acids are linked up by peptide bonds to from long chains called polypeptides. The sequence of amino acids has a bearing on the properties of a protein, and is characteristic for a particular protein. The basic mechanism of protein synthesis is that DNA makes RNA, which in turn makes protein. The central dogma of protein synthesis is expressed as follows: DNA ----------------> DNA --------------------> RNA -------------> PROTEIN Replication Transcription Translation Noble Prize Winners in Protein Synthesis Nobel prize winnes. In the last 15 years several Nobel prizes in physiology and medicine have been awarded for work done on nucleic acids and protein synthesis. In 1975 Alexander Todd of Great Britain was awarded the prize for his studies on nucleotides and nucleotidic coenzymes. The 1958 prize went to Beadle and Tatum for their work showing that one gene is responsible for one enzyme. Lederberg also shared the prize for his work on genetic recombination. The 1959 Nobel prize was shared by Ochoa and Kornberg for successful synthesis of RNA and DNA, repectively. Dr. J. I. Nanavati 54 Watson of U. S. A and Crick and Wilkins of Great Britain received the 1962 prize for elcucidating the structure of DNA. In 1965 the prize was awarded to Jacob, Monod and Lwoff of France for their discovery of regulator genes. The 1968 prize went to the Americans Nirenberg and Khorana for their work on the genetic code, and to Holley (also of U. S. A.) for his finding out the nucleotide sequence of tRNA. In 1969 Delbruck, Hershey and Luria received the prize for their work on the reproductive pattern of viruses, In 1975 Temin was awarded the Nobel prize for his work on RNA directed DNA synthesis. PROTEIN SYNTHESIS Proteins are widely used in cells to serve diverse functions. Some proteins provide the structural support for cells while others act as enzymes to catalyze certain reactions. We have already seen the roles that different enzymes play in building the cell's structure and in catalyzing metabolic reactions, but where do proteins come from? Since the beginning of evolution, cells have developed the ability to synthesize proteins. They can produce new proteins either for reproduction or to simply replace a degraded one. To manufacture proteins, cells follow a very systematic procedure that first transcribes DNA into mRNA and then translates the mRNA into chains of amino acids. The amino acid chain then folds into specific proteins. Protein synthesis requires two steps: transcription and translation. Ribonucleic acid (RNA) was discovered after DNA. DNA, with exceptions in chloroplasts and mitochondria, is restricted to the nucleus (in eukaryotes, the Dr. J. I. Nanavati 55 nucleoid region in prokaryotes). RNA occurs in the nucleus as well as in the cytoplasm (also remember that it occurs as part of the ribosomes that line the rough endoplasmic reticulum). Crick's central dogma. Information flow (with the exception of reverse transcription) is from DNA to RNA via the process of transcription, and thence to protein via translation. Transcription is the making of an RNA molecule off a DNA template. Translation is the construction of an amino acid sequence (polypeptide) from an RNA molecule. Although originally called dogma, this idea has been tested repeatedly with almost no exceptions to the rule being found. Step 1: DNA Transcription Protein synthesis begins in the cell's nucleus when the gene encoding a protein is copied into RNA. Genes, in the form of DNA, are embedded in in the cell's chromosomes. The process of transferring the gene's DNA into RNA is called transcription. Transcription helps to magnify the amount of DNA by creating many copies of RNA that can act as the template for protein synthesis. The RNA copy of the gene is called the mRNA. Dr. J. I. Nanavati 56 DNA and RNA are both constructed by a chain of nucleotides. However, RNA differs from DNA by the substitution of uracil (U) for thymine (T). Also, because only one strand of mRNA is needed when synthesizing proteins, mRNA naturally exist in single-stranded forms. After transcription, the mRNA is transported out of the cell's nucleus through nuclear pores to go to the site of translation, the rough endoplasmic reticulum. Transcription is a process of making an RNA strand from a DNA template, and the RNA molecule that is made is called transcript. In the synthesis of proteins, there are actually three types of RNA that participate and play different roles: a. Messenger RNA(mRNA) which carries the genetic information from DNA and is used as a template for protein synthesis. b. Ribosomal RNA(rRNA) which is a major constituent of the cellular particles called ribosomes on which protein synthesis actually takes place. c. A set of transfer RNA(tRNA) molecules, each of which incorporates a particular amino acid subunit into the growing protein when it recognizes a specific group of three adjacent bases in the mRNA. DNA maintains genetic information in the nucleus. RNA takes that information into the cytoplasm, where the cell uses it to construct specific proteins, RNA synthesis is transcription; protein synthesis is translation. RNA differs from DNA in that it is single stranded, contains Uracil instead of Dr. J. I. Nanavati 57 Thymine and ribose instead of deoxyribose, and has different functions. The central dogma depicts RNA as a messenger between gene and protein, but does not adequately describe RNA's other function. Transcription is highly controlled and complex. In Prokaryotes, genes are expressed as required, and in multicellular organisms, specialized cell types express subsets of gene. Transcription factors recognize sequences near a gene and bind sequentially, creating a binding transcription. Transcription proceeds as RNAP inserts complementary RNA bases opposite the coding strand of DNA. Antisense RNA blocks gene expression. Messenger RNA transmits information in a gene to cellular structures that build proteins. Each three mRNA bases in a row forms a codon that specifies a particular amino acid. Ribosomal RNA and proteins form ribosomes, which physically support the other participants in protein synthesis and help catalyze formation of bonds betweens amino acids. In eukaryotes, RNA is often altered before it is active. Messenger RNA gains a cap of modified nucleotides and a poly A tail. Introns are transcribed and cut out, and exons are reattached by ribozymes. RNA editing introduced bases changes that alter the protein product in different cell types. Step 2: RNA Translation After the mRNA has been transported to the rough endoplasmic reticulum, it is fed into the ribosomal translation machineries. Ribosomes begins to read the mRNA sequence from the 5` end to the 3` end. To convert the mRNA into protein, tRNA is used to read the mRNA sequence, 3 nucleotides at a time. Amino acids are represented by codons, which are 3-nucleotide RNA sequences. The mRNA sequence is matched three nucleotides at a time to a complementary set of three nucleotides in the anticodon region of the corresponding tRNA molecule. Opposite the anticodon region of each tRNA, an amino acid is attached and as the mRNA is read off, the Dr. J. I. Nanavati 58 amino acids on each tRNA are joined together through peptide bonds. Translation is the mechanism by which the triplet base sequence of an mRNA guides the linking of a specific sequence of amino acids to form a polypeptide (protein) on ribosomes. All the proteins a cell needs are synthesized by the cell within itself. Dr. J. I. Nanavati 59 LAC OPERON CONCEPT History The term ‘‘operon’’ was first proposed in a short paper in the Proceedings of the French Academy of Sciences in 1960. From this paper, the so-called general theory of the operon was developed. This theory suggested that all genes are controlled by means of operons through a single feedback regulatory mechanism: repression. Later, it was discovered that the regulation of genes is a much more complicated process. Indeed, it is not possible to talk of a general regulatory mechanism, as there are many, and they vary from operon to operon. Despite modifications, the development of the operon concept is considered one of the landmark events in the history of molecular biology. Components of operon The structural genes The structural genes form a single long polycistronic m RNA molecule and the number of structural genes corresponds to the number of proteins. Each structural gene is controlled independently and transcribe mRNA molecule separately, this, depends on substrate to be utilized. Example: In lac operon three structural genes (Z, Y, A) are associated with lactose utilization. Beta- galactosidase is the product of lac Z that cleaves beta (1-4) linkage of lactose & releases the free monosaccharides. The enzyme permease (a product of lacy) facilitates the lactose the entry inside the bacterium. The enzyme transacylase is a product of lac A where no definite role has been assigned. The lac operon consists of a promoter (p) operator (o) together with structural genes. The lac operon cannot function in the presence of sugars other than lactose. Dr. J. I. Nanavati 60 The operator gene The operator gene is present adjacent to lac Z gene. The operator gene overlaps the promoter region. The lac repressor protein binds to the operator invitro & protect part of the promoter region from the digestion of DNase. The repressor protein binds to the operator & forms an operator –repressor complex which in turn physically blocks the transcription of Z, Y & A genes by preventing the release of RNA polymerase to begin transcription. The promoter gene The promoter gene is long nucleotide &continuous with the operator gene. The promoter gene lies between the operator ®ulator gene, like operators the promoter region consists of palindromic sequences of nucleotides (i.e show 2 fold geometry from a point). These palindromic sequence are recognized by such proteins that have symmetrically arranged subunits. This section of two fold symmetry is present on the CRP site(c-AMP receptor protein site that binds to a protein called CRP).the CRP is encoded by CRP gene, it has been shown experimentally that CRP gene binds to cAMP (c AMP found in e.coli & other organisms) molecule & form a cAMP CRP complex. This complex is required for transcription because it binds to promoter& enhances the attachment of RNA polymerase to the promoter therefore it increases the transcription &translation process. The repressor (regulator) gene Regulator gene determines the transcription of structural gene. It is of two types-active & inactive repressor. It codes for amino acids of a defined repressor protein. After synthesis, the repressor molecules are diffused from the ribosome & bind to the operator in the absence of an induces. Finally the path of RNA polymerase is blocked & m RNA is not transcribed consequently; Dr. J. I. Nanavati 61 no protein synthesis occurs.this type of mechanism occurs in inducible system of active repressor. Moreover when an inducer is present it binds to repressor proteins 7forms an inducer – repressor complex. Due to formation of complex the repressor undergoes changes in the confirmation of shape 7 becomes inactive consequently the structural genes can synthesize the polycistronic m RNA and later synthesize enzyme. In contrast in the reversible system the regulator gene synthesis repressor protein that is inactive & therefore fails to binds to operator, consequently ,proteins are synthesized by the structural genes. However the repressor protein can be activated in the presence of an co- repressor. the co-repressor together with repressor proteins forms the repressor-co repressor complex. This complex binds to operator gene & blocks the protein synthesis Types of operon 1. Lactose (Lac) operon The regulatory mechanism of operon is responsible for the utilization of lactose as a carbon source that is why it is called as lac operon. the lactose utilizing system consists of 2 types of components i.e the structural genes (lacZ, lacy, lacA) the products of which are required for transport and metabolism of lactose ®ulatory genes (lacI, lacP, lacO).these two components together comprises of lac operon.one of the most key features is that operon provides a mechanism for the co- ordinated expression of structural genes controlled by regulatory genes. Operon shows polarity i.e. the genes Z, Y, A synthesize equally qualities of 3 enzymes beta-galactosidase by lac Z, permease by lac Y & acetylase by lac A. These are synthesized in an order i.e. beta-galactosidase at first and acetylase in the last. Regulation of lac operon Regulation of the lac operon by repressor is called negative control. The lac Dr. J. I. Nanavati 62 operon is also under positive control by CRP (or cAMP Receptor Protein; also known as CAP or catabolite activator protein). CRP or CAP is now thought to be bound to its lac binding site at all times (even during repression). During induction, the inducer (either the natural inducer, allolactose, or the synthetic inducer, IPTG, binds to the lac repressor. Inducer-bound repressor does not bind to operator sites. This allows RNA polymerase to bind to the promoter and start transcribing the lac operon. Negative (lac repressor) -------Bound to DNA-----------Not bound to DNA (Type of Control) (Operon off) (Operon on) Positive(CRP protein) --------- Bound to DNA----------Not bound to DNA (Type of control) (Operon on) (Operon off) 2. Tryptophan (Trp) operon The tryptophan operon of E.coli is responsible for the synthesis of the amino acids tryptophan regulation of this operon occurs in such a way that when tryptophan is present in the growth medium, Trp operon is not active but, when adequate trp is present, the transcription of the operon is inhibited, however when its supply is insufficient transcription occurs, the Trp is quite different from the lac operon in that trp acts directly in the repression system rather than as an inducer. Moreover since the trp operon encodes a set of bio- synthetic caranabolic rather than a catabolic enzyme neither glu nor c AMP – CAP has a role in the operon activity. Regulation of Trp Operon Trp is synthesized in 5 steps each required a particular enzyme.in E.coli chromosome the genes encoding these enzymes are located adjacent to one another in the same order as they are used in the bio- synthetic pathway they Dr. J. I. Nanavati 63 are translated from a single polycistronic m RNA molecule. These genes are called TrpE, TrpP, TrpC, TrpB, TrpA, The TrpE gene is the first one translated. Adjacent to the Trp E gene are the promoter, the operator &2 region, called the leader and the attenuated which are designated as TrpL & TrpA respectively.the repressor gene TrpR is located quite for from the gene cluster. The regulatory protein of the repressor system o the TrpR operon is the product of the TrpR gene. mutations either in this gene or in the operator cause constitute initiation of transcription of Trp-m RNA on the lac operon. This regulatory protein is called Trp apo repressor &it does not bind to the operator, unless Trp is present. The apo repressor &the tryptophan molecule joins together to form an active trp repressor which binds to the operator. The reaction scheme is as follows: Apo repressor (no trp) ---------------active repressor (transcription occurs