Carbohydrate Metabolism PDF
Document Details
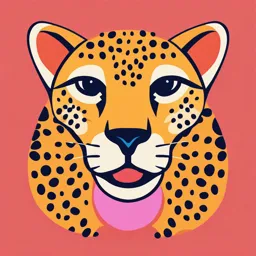
Uploaded by VivaciousCliché
Sphinx University
Sara Abdel-Reheem Atta
Tags
Summary
This document provides a comprehensive overview of carbohydrate metabolism, focusing on the intricacies of glycolysis. It details the various steps, the enzymes involved, the energy yield, and the regulation of the process under both aerobic and anaerobic conditions.
Full Transcript
Carbohydrate Metabolism By Sara Abdel-Reheem Atta Lecturer at medical biochemistry department NADH oxidation synthesizes 3 ATP, whereas, FADH2 oxidation synthesizes 2 ATP. ATP Production - Chemical bonds are classified int...
Carbohydrate Metabolism By Sara Abdel-Reheem Atta Lecturer at medical biochemistry department NADH oxidation synthesizes 3 ATP, whereas, FADH2 oxidation synthesizes 2 ATP. ATP Production - Chemical bonds are classified into high and low-energy bonds according to the free energy liberated on hydrolysis of these bonds. - High-energy bond (or ): On hydrolysis it gives more than 7000 cal per bond, e.g., - and -phosphate bonds of ATP, - Low-energy bond (or -): On hydrolysis, it gives less than 4000 calories per bond, e.g., -phosphate bond of ATP, - - The driving energy for phosphorylation reaction (ADP + Pi ATP) comes from two sources: 1. Oxidative Phosphorylation: - It is a process by which ATP is formed from ADP + Pi, an endergonic reaction, utilizing energy liberated during the transfer of electrons from NADH.H+ or FADH2 to oxygen by components of mitochondrial electron transport chain, an exergonic reaction. 2. Substrate level phosphorylation: - It is the formation of ATP utilizing direct energy liberated from a high energy bond in a compound, i.e., at the level of a substrate level without intermediary oxidation. Fates of the body sugars - In the liver fructose, galactose and mannose are changed to glucose. Glucose may undergo one of many fates: Oxidative fate: 1. Major pathways: A. Glycolysis. B. Krebs' cycle. 2. Minor pathways: A. Pentose shunt. Anabolic fates: 1. Glycogenesis/glycogenolysis. 2. Gluconeogenesis. Glycolysis Definition: Intracellular site and tissue distribution: Steps Bioenergetics Differences between aerobic and anaerobic glycolysis: Regulation Biological importance Special features of glycolysis in RBCs Glycolysis Embden-Myerhof Pathway Definition: -It is a cascade of reactions that converts glucose into two pyruvate molecules (when O2 is available) or into lactate (when O2 is not available; i.e. no mitochondria) aiming at production of ATP and other intermediates. It is also utilized in its opposite direction in gluconeogenesis. Intracellular site and tissue distribution: - It occurs in the cell cytoplasm of all tissues of the body. It is especially important in: 1. RBCs are devoid of mitochondria and depend on glycolysis as the main source of energy. 2. Cornea, lens and some parts of retina which have a limited blood supply and lack mitochondria. 3. Kidney (medulla), testicles, leukocytes and white muscle fibers, where there are relatively few mitochondria. 4. Contracting muscles due to occlusion of blood vessels by the muscular contraction that decreases oxygen. 5. Cancer cells due to dissociation of the high rate of glycolysis from Krebs'; i.e. aerobic production of lactate. 6. Brain and gastrointestinal tract also normally derive most of their energy from glycolysis. Steps of glycolysis: 1. Activation of glucose: - Glucose is activated to glucose-6-phosphate by hexokinase or glucokinase, in presence of ATP as a coenzyme and magnesium ions as a cofactor, where ATP reacts in the form of Mg+2-ATP complex. - Glucokinase is in liver parenchymal and pancreatic islet cells, whereas, hexokinase is in all extrahepatic cells. - It is irreversible because glucose-6-phosphate is at a higher energy level than glucose and products as a whole are at lower energy level than reactants as a whole. CHO CHO H OH Hexokinas, or H OH HO H HO H Glucokinase H OH H OH H OH Mg+2 H OH O CH2OH ATP ADP CH2-O P-OH Glucose OH Glucose-6-Phosphate Differences between glucokinase and hexokinase: Hexokinase Glucokinase Specific gene Different gene Site: most of tissues. Liver parenchymal and pancreatic islet cells. Substrate: It acts mainly on glucose but similar to other hexokinase isoenzymes, it can act at a slower rate on fructose and is therefore called hexokinase D or type galactose. M. Induction: It is a non-inducible enzyme; It is an inducible enzyme; i.e. affected by i.e. constitutive (present at a constant glucose and insulin concentration. level). Affinity for glucose: It has a high It has a low affinity (a high Km). It acts affinity as indicated by its low Km 0.05 optimally at high blood glucose mM in RBCs for glucose (acts at very concentration above 5 mmol/L. low glucose concentration). Allosteric inhibitor (glucose-6- No. phosphate): Yes. Effect of feeding and insulin: No change Increased rate of activity (induction). in activity Effect of fasting or diabetes: No change Decreased rate of activity (repressed). in activity. Function: Activates glucose for energy Activates glucose to remove it from the production irrespective of its blood blood following a meal for storage. concentration. 2. Glucose-6-phosphate is isomerized into fructose-6-phosphate by phosphohexose isomerase that is an aldose-ketose isomerase. CHO CH2OH H OH O HO H Phosphohexose HO H H OH Isomerase H OH H OH H OH O O CH2-O P-OH CH2-O P-OH OH OH Glucose-6-Phosphate Fructose-6-Phosphate 3. Activation of fructose-6-phosphate by phosphofructokinase 1 into fructose-1, 6-diphosphate requiring ATP and Mg+2. This the most important rate limiting irreversible reactions of glycolysis since fructose-1,6-diphosphate is at a higher energy level than fructose-6-phosphate and products as a whole are at a lower energy level than reactants as a whole. Phosphofructokinase 2 is an enzyme different from phosphofructokinase 1 which converts a tiny amount of fructose-6- p to fructose 2,6 dihosphate. O CH2OH CH2-O P-OH O O OH HO H Phosphofructokinase HO H H OH H OH H OH Mg+2 H OH O ATP ADP O CH2-O P-OH CH2-O P-OH OH OH Fructose-6-Phosphate Fructose-1,6-diphosphate 4. Cleavage of fructose-1,6-diphosphate into 2 triose phosphates by aldolases: There are several tissue specific aldolase isoenzymes. Aldolase A occurs in most tissues and acts on fructose-1,6- diphosphate. Aldolase B occurs in liver and kidney and acts on fructose-1-phosphate and fructose-1,6-diphosphate. 5. Interconversion of the two triose phosphates by phosphotriose isomerase enzyme: Withdrawal of glyceraldehyde-3-phosphate make the equilibrium is in favor of its formation. O CH2-O P-OH OH O O Dihydroxyacetone CH2-O P-OH CH2OH phosphate O OH Phosphotriose HO H Aldolase Isomerase H OH A or B H OH O CHO CH2-O P-OH H OH O Glyceraldehyde- OH (2) CH2-O P-OH 3-phosphate Fructose-1,6-diphosphate OH 6. Glyceraldehyde-3-phosphate (two molecules) is oxidized into 1,3-diphosphoglycerate by glyceraldehyde-3-phosphate dehydrogenase, an SH-containing enzyme, in presence of NAD as a coenzyme and inorganic phosphate (Pi). 7. Phosphoglycerate kinase unlike most other kinases , is physiologically reversible converts 1,3-diphosphoglycerate into 3- phosphglycerate and the high-energy phosphate at C1 is utilized to produce an ATP from an ADP. - It is an important reaction and one example of the substrate level phosphorylation; i.e. production of ATP from ADP + Pi utilizing high-energy bond in a substrate directly without intermediates. O O O 2 ADP 2 ATP C ~ P-OH C OH OH +2 Mg H OH O H OH O (2) CH2-O Phosphoglycerate (2) CH2-O P-OH P-OH OH Kinase OH 1,3-DiPhospho-Glycerate 3-Phospho-Glycerate 8. The phosphate group is moved from C3 to C2 in 3- phophoglycerate by phosphoglycerate mutase. 2,3-bi- phosphoglycerate is an intermediate and/or cofactor in this reaction. O O C OH 3-Phospho-Glycerate C OH O H OH O Mutase P-OH H O (2) CH2-O P-OH (2,3-DiPhospho-Glycerate) (2) CH2-OH OH OH 2-Phospho-Glycerate 3-Phospho-Glycerate 9. Enolase enzyme: In presence of Mg+2 or Mn+2 ,it dehydrates; i.e. removes a water molecule, from 2- phosphoglycerate and redistributes energy within the molecule to form phosphoenol pyruvate which contains a high phosphate energy bond at C2. This reaction is reversible despite the energy product. O O C OH C OH O O H O P-OH Enolase O ~ P-OH OH (2) CH2-OH OH Mg+2 (2) CH2 2 H 2O Phosphoeno 2-Phospho-Glycerate Pyruvate 10. A second substrate level phosphorylation then occurs at pyruvate kinase that utilizes the high-energy bond at C2 of phosphoenol pyruvate to convert an ADP into an ATP. This reaction is irreversible because phosphoenol pyruvate is at a higher energy level than pyruvate. O 2 ADP 2 ATP O O C OH C OH C OH O +2 Spontaneous Mg O ~ P-OH OH O OH Pyruvate Kinase (2)CH 2 (2) CH2 (2) CH3 Phosphoenol Enol-Pyruvate Pyruvate Pyruvate 11. Under aerobic conditions (presence of oxygen and mitochondria), the steps of glycolysis end at this point and pyruvate is oxidatively decarboxylated into acetyl-CoA that enters Krebs' cycle for further oxidation. 12. Under anaerobic conditions; i.e. absence of O2 or mitochondria, NADH.H+ is reoxidized to allow glycolysis to continue by lactate dehydrogenase (LDH) that reversibly reduces pyruvate into lactate. O O + C OH 2 NADH.H 2 NAD C OH O H OH Lactate (2) CH3 Dehydrogenase (2) CH3 Pyruvate Lactate CHO CHO CH2OH Hexokinase, or H OH H OH Phosphohexose O Glucokinase HO H Isomerase HO H HO H H OH Mg+2 H OH H OH H OH H OH H OH ATP ADP+Pi O O CH2OH CH2-O P-OH CH2-O P-OH Glucose OH OH Glucose-6-Phosphate Fructose-6-Phosphate ATP Phosphofructokinase Mg+2 O ADP CH2-O P-OH O O OH CH2-O P-OH CH2OH O OH Dihydroxyacetone- HO H phosphate H OH H OH Phosphotriose O Aldo las e CH2-O P-OH O O Isomerase A or B C ~ P-OH OH OH 2 NADH.H+ 2 NAD + Pi CHO Fructose-1,6- diphosphate H OH H OH O O CH2-O P-OH CH2-O P-OH Glyceraldehyde- OH OH 3-Phosphate (2) Glyceraldehyde- (2) 1,3-DiPhospho- Dehydrogenase Glycerate 3-phosphate 2 ADP Mg+2 Phosphoglycerate Kinase 2 ATP O O O 2 H2O 3-Phospho- C OH O C OH C OH O Glycerate Mg+2 O ~ P-OH H OH O H O P-OH Mutase Enolase OH CH2-O P-OH CH2-OH OH CH2 OH (2) 2-Phospho-Glycerate (2) Phosphoenol (2) 3-Phospho-Glycerate Pyruvate 2 ATP +2 O Pyruvate O O Kinase Mg C OH C OH 2 NAD 2 NADH.H+ C OH 2 ADP OH H OH O Spontaneous CH2 CH3 CH3 Lactate (2) Enol- (2) Lactate (2) Pyruvate Dehydrogenase Pyruvate Bioenergetics of (or Energy yield from) glycolysis: Under aerobic conditions: 1. Total ATP lost = 2 ATP, as under anaerobic conditions. 2. Total ATP gained = 8 or 10 ATP are generated as follows, 4 ATP (obtained by substrate level phosphorylation) + 2 NADH.H+ chain (produced from oxidation of glyceraldehyde-3-phosphate) 2 X 2 or 3 ATP = 4 or 6 ATP, after oxidation in the functioning respiratory chain, depending on the type of transporting shuttle used. Thus, 8 or 10 ATP are generated. 3. Net ATP gained = 6 or 8 ATP as follows, 8 ATP – 2 ATP = 6 ATP ,or 10 ATP – 2 ATP = 8 ATP for the aerobic oxidation of one mole of glucose. Under anaerobic conditions: 1- Total ATP lost = 2 ATP; One ATP in the activation of glucose to glucose-6-phosphate. One ATP in the activation of fructose-6-phosphate to fructose1,6- diphosphate. 2- Total ATP gained = 4 ATP; 2 ATP by substrate level phosphorylation from 1,3- diphosphoglycerate 2 ATP by substrate level phosphorylation from phosphoenol pyruvate. 3- Net ATP gained = 4 ATP gained - 2 ATP lost = 2 ATP for the anaerobic oxidation of one mole of glucose into lactate. Differences between aerobic and anaerobic glycolysis: Aerobic Anaerobic 1- End product pyruvate Lactate 2- Energy 6-8 ATP 2 ATP 3- Regeneration Through respiratory chain Through lactate of NAD+ in mitochondria formation 4- Availability to Available and 2 pyruvate Not available as TCA in can oxidize to give 30 lactate is cytosolic mitochondria ATP substrate Regulation (Control) of Glycolysis: A. Key regulatory enzymes: I. Phosphofructokinase-1: II. Hexokinase III. Pyruvate kinase: B. Hormonal regulation: I. Insulin: II.Adrenaline and glucagon C. In vitro inhibition of glycolysis: I. 2-Deoxyglucose II.Arsenate III.Iodoacetate IV.Fluoride B. Hormonal regulation: I. Insulin: stimulates synthesis of glucokinase, phosphofructokinase and pyruvate kinase, so it stimulates glycolysis. It also induces glucose transporters to provide cells with glucose for glycolysis. II.Adrenaline and glucagon are inhibitory by inhibiting pyruvate kinase. C. In vitro inhibition of glycolysis: I. 2-Deoxyglucose inhibits hexokinase and glucokinase. II.Arsenate closely resembles inorganic phosphate and replaces it in the reaction catalyzed by glyceraldehyde-3-phosphate dehydrogenase producing 1-arseno-3-phosphoglycerate, which is unstable. This compound rapidly decomposes to give 3- phosphoglycerate plus heat. This means that glycolysis continues in the presence of arsenate, but producing two ATPs less. III.Iodoacetate, an ―SH poison inhibits glyceraldehyde-3-phosphate dehydrogenase which contains this group at its active site. IV.Fluoride inhibits enolase. So, on accurately measuring blood glucose levels the samples should be fluorinated to stop glycolysis in RBCs. Biological importance (Functions) of glycolysis: 1. Glucose oxidation producing ATP. It is the major source of energy in certain tissues, e.g. RBCs and skeletal muscles. 2. It provides pyruvic acid needed for Krebs' cycle. 3. It is a link with fat metabolism, e.g. dihydroxyacetone phosphate into glycerol 3-phosphate in adipose tissue. 4. It a link with amino acid metabolism, e.g., 3- phopshoglycerate into serine and pyruvate into alanine and vice versa. 5. Production of 2,3-DPG that is important in tissue oxygenation. 6. It is the major source of lactic acid that is gluconeogenic. 7. Reversal of glycolysis is gluconeogenesis, an important source of glucose. 8. Main pathway of metabolism of fructose from the diet. Special features of glycolysis in RBCs (Rapoport- Lubering cycle): 1- Mature RBCs contain no mitochondria, thus: a) they depend only upon glycolysis for energy production (=2 ATP). b) Lactate is always the end product. 2- Glucose uptake by RBCs is independent on insulin hormone. 3- Reduction of met-hemoglobin: glycolysis produces NADH+H+ , which used for reduction of met-hemoglobin in red cells. This reaction is catalyzed by cytochrome b5-methemoglobin reductase system (cyt b5): Met – Hb (Fe+++) + NADH+H+ Hb (Fe++) + NAD+ Rapoport-Lubering cycle is a side-pathway or a shunt from glycolysis that aims at dissipating or waste the excess ATP. The cells lack many metabolic reactions that require energy. It is formed of two steps: A. 1,3-diphosphoglycerate is mutated into 2,3-diphosphoglycerate (2,3- DPG): The transfer of phosphate from C1 to C2 results in transformation of phosphate from high to low energy bond. B. By 2,3-diphosphoglycerate phosphatase, the phosphate group at C2 of 2,3-DPG is hydrolyzed producing 3-phosphoglycerate. This reaction is also activated by phosphoglycerate mutase. 3-phosphoglycerate rejoins the ordinary pathway of glycolysis. - Biochemical significance of 2,3-Diphosphoglycerate (2,3-DPG): A. 2,3-DPG acts as a cofactor in the conversion of 3-phosphoglycerate to 2- phosphoglycerate by mutase. B. It decreases the affinity of hemoglobin (Hb) to oxygen helping oxygen dissociation and unloads oxygen in tissue capillaries for oxygenation. C. Red blood cells have a high concentration of 2,3-DPG. During storage of blood in blood banks, 2,3-DPG concentration decreases gradually to reach traces at ten days. So, Hb of this blood has a high affinity to O2 and is not suitable for blood transfusion to hypoxic patients. RBCs membrane is impermeable to 2,3-DPG. So, instead of adding 2,3-DPG inosine is added which penetrates RBCs easily and its ribose is changed very slowly into 2,3-DPG by HMP shunt and glycolysis. D. Fetal hemoglobin (alpha2 gamma2) binds 2,3-DPG less strongly than adult hemoglobin and therefore has a higher O2 affinity to extract O2 from mother's blood.