Gene to Protein PDF
Document Details
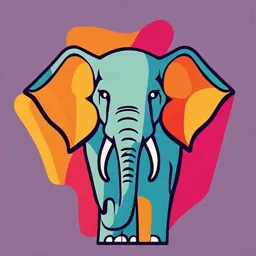
Uploaded by BestSellingAnemone
Tags
Related
- Molecular Biology and Cytogenetics - Translation PDF
- Molecular Biology and Cytogenetics - Translation PDF
- Molecular Biology and Cytogenetics: Translation from mRNA to Protein (PDF)
- Ch.8 Microbial Genetics Replication and Protein Synthesis PDF
- Biology Gene Expression Revision Notes PDF
- Biology Chapter 17 Lecture PDF
Summary
This document provides a detailed overview of gene to protein conversion, covering transcription initiation, elongation, termination, and processing. It explores RNA's role and the significance of protein structure for function.
Full Transcript
Gene to protein Lect 8 What are gene expressions? The process of going from DNA to a functional product (protein) DNA - RNA - Protein Genotype; an organism's hereditary information Phenotype; actual observable or physiological traits Our genotype and its interactions with the environment determines...
Gene to protein Lect 8 What are gene expressions? The process of going from DNA to a functional product (protein) DNA - RNA - Protein Genotype; an organism's hereditary information Phenotype; actual observable or physiological traits Our genotype and its interactions with the environment determines our phenotype. DNA (deoxyribonucleic acid) is the heritable material that is used to store and transmit information from generation to generation. RNA (ribonucleic acid) acts as a messenger to allow the information stored in the DNA to be used to make protein. Proteins carry out cellular functions; there are three main steps - transcription, processing and translation. Describe the sequence of events in protein synthesis: 1) the process of transcription, including its initiation Transcription 1. Initiation: ○ RNA polymerase, the main transcription enzyme, recognizes and binds to a promoter sequence near the beginning of a gene. ○ This binding marks the start of transcription for that specific gene. ○ The DNA double helix unwinds, creating a region known as the transcription bubble. Transcription Factors: ○ ○ ○ ○ Transcription factors are proteins that play a pivotal role in regulating gene expression. They help determine which genes are turned on or off by binding to nearby DNA. Activator transcription factors enhance gene transcription, while repressor factors decrease it. These factors allow cells to perform logical operations, combining different sources of information to decide whether to express a gene. Transcription Initiation Complex: ○ ○ ○ ○ The assembly of transcription factors, including the TATA box binding protein (TBP), forms the transcription initiation complex. The TATA box, located about 25 nucleotides upstream of the gene, serves as a crucial binding site for these factors. TBP is part of a larger protein complex that includes RNA Polymerase II. Once assembled, this complex marks the start of transcription. Initiation of Transcription: ○ ○ ○ ○ With the transcription initiation complex in place, RNA Polymerase II binds to it. Additional transcription factors also join at the promoter region of the gene. This binding signifies the beginning of transcription. RNA Polymerase II reads the DNA template strand, synthesising a complementary RNA strand. Coding and Non-Coding Strands: The coding strand of DNA has the same sequence as the RNA transcript, except with Thymine (T) instead of Uracil (U). ○ The non-coding strand, also known as the template strand, serves as the template for RNA synthesis. 2. Elongation: ○ Once initiated, RNA polymerase begins moving downstream along the gene. ○ It reads the DNA strand and adds complementary RNA nucleotides to the growing mRNA (messenger RNA) chain. ○ During this process, RNA uses Uracil (U) instead of Thymine (T), pairing Adenine (A) with Uracil. ○ Elongation of Transcription: During elongation, the DNA strand unwinds with around 10 to 20 nucleotides exposed at a time. RNA Polymerase II adds complementary RNA nucleotides to the 3' end of the growing RNA transcript. This addition occurs through the formation of a phosphodiester bond between the 3' hydroxyl group (3'OH) of the growing RNA transcript and the 5' phosphate group of the incoming RNA nucleotide. As the RNA polymerase moves along the DNA template, the double helix reforms behind it, ensuring the integrity of the DNA structure. 3. Termination: ○ Polymerase continues transcribing until it encounters a specific sequence called the terminator. ○ At the terminator, polymerase detaches from the DNA strand. ○ Transcription of that particular gene segment is now complete. Termination of Transcription: Transcription termination occurs after the RNA polymerase transcribes a specific sequence called the polyadenylation signal (AAUAAA) in the DNA. Nuclear enzymes recognize this signal and cleave the pre-mRNA transcript downstream of the signal. After cleavage, RNA Polymerase II dissociates from the DNA, releasing the pre-mRNA transcript. The pre-mRNA transcript is now ready for further processing, such as splicing and addition of a poly-A tail. Fidelity and Proofreading: During transcription, the fidelity or accuracy of nucleotide incorporation by RNA Polymerase II is lower compared to DNA replication. This lower fidelity is due to the lack of proofreading mechanisms during transcription, unlike DNA replication where DNA polymerases have proofreading capabilities to correct errors. 2) the processing of pre-mRNA to mature mRNA 1. Capping (5’ Capping): ○ During capping, a modified guanine nucleotide is added to the 5’ end of the pre-mRNA transcript. ○ This modified guanine, known as a “cap,” serves several critical functions: Protection: It shields the nascent mRNA from degradation by ribonucleases. Ribosome Binding: Initiation factors involved in protein synthesis recognize the cap, aiding in ribosome binding during translation. Export: The cap facilitates the export of mRNA from the nucleus to the cytoplasm. ○ Think of it as a protective hat for the mRNA! 2. Tailing (Polyadenylation) (3’ Poly-A Tail): ○ Tailing involves adding a sequence of 50 to 250 adenine nucleotides (poly-A tail) to the 3’ end of the pre-mRNA transcript. ○ Functions of the poly-A tail: Stability: It protects the mRNA from degradation. Transport: It assists in transporting the mature mRNA from the nucleus to the cytoplasm. Translation: The poly-A tail is involved in binding proteins that initiate translation. ○ Imagine it as the mRNA’s stylish tail, ensuring stability and efficient travel! 🎩 3. Splicing: Splicing is the process of removing introns (non-coding regions) from the pre-mRNA transcript. What remains after splicing are the exons—the segments that encode proteins. Why is splicing crucial? ○ It ensures that only the functional coding regions are retained in the mature mRNA. ○ Exons are like puzzle pieces that fit together to create a functional mRNA molecule. ○ This optimised mRNA can then be translated into a protein. Splicing and Alternative Splicing in mRNA Processing Splicing Basics: In splicing, introns (non-coding regions) are removed from the pre-mRNA transcript. Exons (coding regions) are then joined together to form mature mRNA. Spliceosome Complex: The spliceosome is a large complex of proteins and small RNAs. It carries out the splicing process by recognizing splice sites and cutting out introns. Alternative Splicing: Alternative splicing allows for the production of multiple gene products from the same gene. Different combinations of exons are joined together during splicing. This results in the generation of various forms of mRNA from the same pre-mRNA population. Impact of Alternative Splicing: With around 20,000 genes in humans, alternative splicing greatly expands protein diversity. It enables the creation of numerous protein isoforms with distinct functions and regulatory roles. Summary: Splicing removes introns and joins exons to form mature mRNA. The spliceosome complex facilitates this process. Alternative splicing leads to diverse mRNA and protein products, contributing to the complexity of the human proteome. Genes and their product an vary greatly in size 1. Gene Structure and mRNA Processing: ○ Genes are like intricate blueprints encoded in our DNA. Let’s dissect the details: Your gene spans 18,000 base pairs (bp) on chromosome 6. The initial transcript, called pri-RNA, stretches approximately 18,000 nucleotides (nt). After some magical molecular editing, the mature mRNA emerges, condensed to around 1900 nt. This mRNA harbors 8 exons, which are the coding regions that hold the instructions for building proteins. Ultimately, this gene encodes a protein composed of 339 amino acids—the building blocks of life. 2. The Human Genome in Numbers: ○ Our genome is a vast library of genetic information: It spans approximately 3,000 million base pairs (Mbp). Within this vast expanse, we find an astonishing 20,000 genes. Each gene is a unique chapter, contributing to our biological symphony. 3. Gene Size Diversity: ○ Now, let’s explore two captivating examples: TATA-box binding protein (TBP): 1. This gene sprawls across 180,000 bp on chromosome 4. 2. It boasts an impressive 67 exons, intricately woven together. 3. The resulting protein stretches across 3144 amino acids—a molecular masterpiece. Huntingtin (HTT): 1. While the details remain elusive, HTT exemplifies the diversity in gene and protein size. 2. It’s a testament to the intricate dance of life encoded within our DNA. protein sequence determines its final structure structure determines function DNA mutations can affect ability of the protein to function 1. Codons and tRNA: ○ Codons are like genetic sentences—triplets of nucleotides (A, C, G, U) in mRNA. ○ tRNA (transfer RNA) molecules, found in the cytosol, carry specific anticodons. ○ Each tRNA anticodon pairs with a complementary mRNA codon. ○ Think of tRNA as molecular couriers, delivering the right amino acids to the ribosome. 2. Hydrogen Bonds and Translation: ○ Hydrogen bonds form between the mRNA codon and the tRNA anticodon. ○ This precise pairing ensures that the correct amino acid is added to the growing protein chain. 3) The link between DNA sequence and protein sequence 1) mRNA Binding Sites in the Ribosome: ○ The ribosome, our cellular assembly line, has three key sites: i. A site (Aminoacyl site): Here, the “next-in-line” tRNA carries the correct amino acid docks. ii. P site (Peptidyl site): This holds the tRNA that carries the growing polypeptide chain. iii. E site (Exit site): tRNAs exit from here after their job is done. 2) Arrival of New Amino Acids: ○ Picture a conveyor belt: The ribosome moves along the mRNA, reading codons. ○ When the ribosome reaches the A site, the next-in-line tRNA (with the matching anticodon) arrives. ○ This tRNA carries the correct amino acid specified by the mRNA codon. ○ The ribosome catalyses the formation of a peptide bond between the new amino acid and the growing polypeptide. 4) the process of translation at the ribosome 1. tRNA: The Molecular Translator: ○ Imagine tRNA as a tiny interpreter, bridging the gap between the genetic code (mRNA) and the amino acid sequence of proteins. ○ Here’s how it works: tRNA molecules, found within the cytosol, carry specific anticodons. Each anticodon pairs with a complementary mRNA codon during translation. Like a multilingual guide, tRNA ensures that the right amino acids are delivered to the ribosome. Translation initiation within the ribosome. 🧬🌟 1. Initiation Complex Assembly: ○ Imagine a grand ballroom: The ribosome awaits its partners. ○ The large ribosomal subunit joins the small ribosomal subunit, completing the initiation complex. ○ The small ribosomal subunit, already bound to the initiator tRNA (carrying methionine, Met), approaches the 5’ cap of the mRNA. ○ It’s like dancers taking their positions on the dance floor! 2. Scanning for the Start Site: ○ Picture the small ribosomal subunit as a curious explorer. ○ It scans downstream along the mRNA, searching for the translation start site (usually the AUG codon). ○ When it finds the AUG, the initiation process begins. ○ Think of it as a quest for the perfect dance partner! 3. Energy and Hydrogen Bonds: ○ Energy in the form of GTP (Guanosine triphosphate) fuels this assembly. ○ Hydrogen bonds form between the initiator tRNA’s anticodon and the mRNA’s start codon (AUG). ○ The ribosome is now ready to waltz through translation. 💃🕺 Translation elongation Codon Recognition and tRNA Binding: ○ Picture the ribosome as a precision instrument. ○ The anticodon of the incoming tRNA base pairs with the mRNA codon in the A site. ○ GTP (Guanosine triphosphate) is invested to ensure accuracy and efficiency. ○ It’s like aligning puzzle pieces—the right tRNA fits the mRNA codon perfectly! 2. Peptide Bond Formation: ○ Imagine the ribosome as a master chef. ○ The large ribosomal subunit, with its rRNA, catalyzes the formation of a peptide bond. ○ This bond links the incoming amino acid to the growing polypeptide chain. ○ The tRNA in the P site donates its amino acid. ○ It’s like adding ingredients to a gourmet dish! 3. Translocation and Energy: ○ Now, the ribosome shifts the tRNA from the A site to the P site. ○ The tRNA in the P site moves to the E site and is released. ○ Energy (GTP) is required for this precise movement. Translation: termination. 🧬🌟 1. Stop Codon Recognition: ○ Picture the ribosome as a conductor, bringing the symphony to its conclusion. ○ When a stop codon (UAA, UAG, or UGA) enters the A site, it’s recognized by a release factor. ○ This release factor fits neatly into the P site (though it isn’t a tRNA). ○ It’s like the final note of a musical piece—the signal that the protein is complete. 2. Hydrolysis and Recycling: ○ The bond between the P-site tRNA and the last amino acid is hydrolyzed. ○ The polypeptide is released, ready to perform its cellular role. ○ Two GTP molecules are hydrolyzed to provide the energy for this final step. ○ Ribosome components can be recycled for future performances. Gene expression control is crucial for achieving the right thing at the right time and in the right place within a cell. This control can be both temporal (timing) and spatial (location). Let’s explore why it matters: 1. Temporal Control: ○ Housekeeping Proteins: Some proteins are continuously produced because they perform essential functions in the cell. These proteins have longer half-lives and are present in large quantities. For example, tubulin, a component of microtubules, falls into this category. ○ Stimulus-Responsive Proteins: Other proteins are produced in response to specific stimuli. For instance: Cell Signaling: Ligands binding to cell surface receptors or activating intracellular receptors trigger a cascade of events. Transcription Activation: Some signals enter the nucleus and activate transcription, resulting in the production of short-lived proteins that carry out specific functions. 2. Spatial Control: ○ Proteins are often localized to specific cellular compartments or structures. Their presence in the right place ensures proper function. ○ For example, microtubules are essential for cell division and are precisely positioned within the cell. Why is Control of Gene Expression Important? It allows cells to respond dynamically to changing conditions. It ensures efficient resource utilization by producing proteins only when needed. It contributes to cellular specialization and adaptation. Understand the concept of vesicular transport and secretion of a protein Protein processing and sorting within the cell. 🧬🌟 1. Proteins Destined for the Cytosol: ○ Some proteins are continuously produced and function in the cytosol. ○ These proteins complete translation on free ribosomes. ○ Translation begins on these ribosomes, which are not associated with the endomembrane system. ○ They are like independent artisans crafting proteins for the cell. 2. Proteins Going Through the Endomembrane System: ○ Other proteins are destined for the endomembrane system (ER, Golgi, etc.). ○ These proteins complete translation at fixed ribosomes on the rough ER (RER). ○ It’s like specialized assembly lines for specific protein destinations. 3. How Does a Ribosome “Know”?: ○ The ribosome’s location determines its function. ○ Fixed ribosomes on the RER are specialized for proteins destined for the endomembrane system. ○ Free ribosomes in the cytosol produce proteins for general cellular functions. Signal peptide - at N terminus of the protein (20aa) SRP - signal recognition particle At step 6 of protein synthesis, a fascinating decision awaits: secretory proteins and membrane proteins take divergent paths. 1. Secretory Proteins (e.g., Insulin): ○ These proteins are solubilized within the lumen of the endoplasmic reticulum (ER). ○ They are destined for secretion or export from the cell. ○ Imagine them as travellers preparing for a journey. 2. Membrane Proteins: ○ These proteins remain anchored to the ER membrane. ○ They will play essential roles in cell membranes. ○ Think of them as steadfast sentinels guarding the cell’s boundaries. Both groups then embark on vesicles, like cellular ships, heading toward the Golgi apparatus for further maturation and sorting. Summary of Common Post-Translational Modifications: Phosphorylation: Addition of a phosphate group; confers activity or targets proteins. Methylation: Addition of a methyl group; modifies activity or gene expression. Acetylation: Addition of an acetyl group; regulates function or interactions. Biotinylation: Addition of biotin; used in assays or for specific functions. Carboxylation: Addition of a carboxylic acid group; modulates activity or stability. Carbohydrate Addition: Affects membrane proteins, influencing folding and interactions. Cleavage: Proteolytic processing; activates proteins or regulates pathways. Ubiquitination: Addition of ubiquitin; targets proteins for degradation or regulates activity. Functional Implications: These modifications affect protein activity, interactions, localization, and stability. They play roles in enzymatic activity, signalling pathways, gene regulation, and cellular processes. Location: Some modifications occur in the Golgi (e.g., glycosylation), while others occur in the cytosol (e.g., phosphorylation). Objective: Post-translational modifications are crucial for protein functionality, regulation, and cellular processes after translation is complete. Understanding them helps decipher protein function and cellular mechanisms. Discuss primary, secondary, tertiary and quaternary levels of protein structure and the relationship between structure and function of proteins -refer to lecture 14