Furlan and Adameyko DevBio 2018 PDF
Document Details
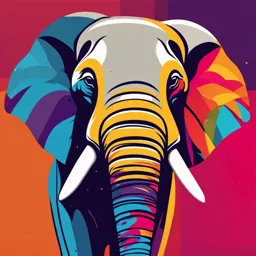
Uploaded by FormidableJadeite611
null
2018
Alessandro Furlan, Igor Adameyko
Tags
Summary
This article, published in Developmental Biology in 2018, explores the multifaceted roles of Schwann cell precursors (SCPs). It investigates their multipotency, functions, and potential therapeutic value, examining their similarities to neural crest cells. The research also analyzes the signals governing SCPs and their embryonic origin and evolutionary connections.
Full Transcript
Developmental Biology 444 (2018) S25–S35 Contents lists available at ScienceDirect Developmental Biology journal homep...
Developmental Biology 444 (2018) S25–S35 Contents lists available at ScienceDirect Developmental Biology journal homepage: www.elsevier.com/locate/developmentalbiology Schwann cell precursor: a neural crest cell in disguise? MARK a b,c,⁎ Alessandro Furlan , Igor Adameyko a Cold Spring Harbor Laboratory, Cold Spring Harbor, New York 11724 USA b Department of Physiology and Pharmacology, Karolinska Institutet, 17177 Stockholm, Sweden c Center for Brain Research, Medical University Vienna, 1090 Vienna, Austria A BS T RAC T Schwann cell precursors (SCPs) are multipotent embryonic progenitors covering all developing peripheral nerves. These nerves grow and navigate with unprecedented precision, delivering SCP progenitors to almost all locations in the embryonic body. Within specific developing tissues, SCPs detach from nerves and generate neuroendocrine cells, autonomic neurons, mature Schwann cells, melanocytes and other cell types. These properties of SCPs evoke resemblances between them and their parental population, namely, neural crest cells. Neural crest cells are incredibly multipotent migratory cells that revolutionized the course of evolution in the lineage of early chordate animals. Given this similarity and recent data, it is possible to hypothesize that proto- neural crest cells are similar to SCPs spreading along the nerves. Here, we review the multipotency of SCPs, the signals that govern them, their potential therapeutic value, SCP's embryonic origin and their evolutionary connections. We dedicate this article to the memory of Wilhelm His, the father of the microtome and “Zwischenstrang”, currently known as the neural crest. 1. SCPs mock the crest: multipotency and functions of nerve- today, we do not clearly understand the function of these cells in the associated cells context of the nerve fibers they associate with in a growing embryo. According to published experimental results, peripheral nerves do not Schwann cell precursors (SCPs), embryonic glial progenitors asso- require SCPs for their navigation or outgrowth as evident in Htpa-Cre/ ciated with almost omnipresent nerve fibers, are neural crest-derived R26RDTA mouse embryos with ablated neural crest cells (Coppola et al., cells that have recently attracted considerable attention from different 2010). SCPs migrate along the nerve fibers and proliferate on their sides of developmental and regenerative biology (Kastriti and surfaces using the nerve as a niche, and, via this mechanism, SCPs Adameyko, 2017). In recent years, numerous reports highlighted the spread throughout the body together with developing innervation existence of novel, non-canonical roles of the peripheral nervous (Heermann et al., 2012). There are some exceptions to this rule. For system, including the delivery of multipotent cells to various locations instance, in the facial region, post-migratory SOX10+ cells (which could (Furlan et al., 2017), the induction of body part regeneration (Kumar be considered as pre-SCPs) demarcate the future pathways that nerves et al., 2007), stem cell niche control (Brownell et al., 2011) and fine would take and later cling onto the passing peripheral nerve fibers positional information relay (Li et al., 2013). Many of these previously (Freter et al., 2013). The genetic ablation of SCPs leads to neuronal unnoticed roles are mediated by SCPs in the developing embryo and phenotypes only after E12.5, when nerve-associated progenitors com- often result from SCP's multipotency, which is similar to their parental mit to glial fate and start to acquire features of immature Schwann cells neural crest population (Ivashkin et al., 2014; Kaucka and Adameyko, (Davies, 1998). Thus, the early functions of SCPs should not be reduced 2014). only to obligations toward their carriers, i.e., neuronal processes projecting towards the periphery, and instead are often related to 1.1. Non-canonical functions of peripheral nerves and SCPs mediating nerve-to-tissue interactions. One of such example includes neurovascular alignment, a recently Historically, SCPs were merely considered to be direct progenitors discovered phenomena (despite being noted previously by Andreas of immature Schwann cells with generally unclear functions. Even Vesalius (Vesalius, 2003)) that outlines the alignment of vessels and ⁎ Corresponding author at: Department of Physiology and Pharmacology, Karolinska Institutet, 17177 Stockholm, Sweden. E-mail address: [email protected] (I. Adameyko). https://doi.org/10.1016/j.ydbio.2018.02.008 Received 18 December 2017; Received in revised form 14 February 2018; Accepted 14 February 2018 Available online 16 February 2018 0012-1606/ © 2018 The Authors. Published by Elsevier Inc. This is an open access article under the CC BY license (http://creativecommons.org/licenses/BY/4.0/). A. Furlan, I. Adameyko Developmental Biology 444 (2018) S25–S35 peripheral nerves into organized units within the embryonic skin. In oligogangliosis (Uesaka et al., 2015). Similar to parasympathetic and this case, nerve-associated SCPs generate signals that instruct geo- enteric neurons, neuroendocrine cells of the adrenal medulla (chro- metric remodeling of the vascular network and guide differentiation of maffin cells) are generated from SCPs starting from E11.5 in the mouse primitive vessels into arterioles and venules. To be more precise, embryo (Furlan et al., 2017). The experiments clearly demonstrate that CXCL12 and VEGF are secreted by the SCPs of the nerves innervating numerous SCPs are required to generate a local parasympathetic skin. These signaling molecules induce geometric re-organization of the ganglion or a cluster of chromaffin cells inside of the adrenal medulla capillary network (VEGF-A) and differentiation of arterioles (CXCL12) via a method that does not involve transiently amplifying progenitors that ultimately run in parallel to the nerve trajectory (Li et al., 2013; (Dyachuk et al., 2014; Furlan et al., 2017). Thus, SCPs do not behave Mukouyama et al., 2002). similarly to adult stem cells or anything related to the classical Additional interesting phenomena are based on the capacity of SCP organization of the stem cell niche. According to this logic, the local cells to detach from the nerve fibers and convert to the other fates of density of innervation defines the pool of SCPs available for recruit- the original neural crest spectrum. ment, which subsequently becomes a key factor influencing the size of the derived tissue or organ. 1.2. SCP-dependent origin of neural crest-derived cell types Melanocytes, Schwann cells, autonomic neurons and chromaffin cells belong to a somewhat “nervous system-related” spectrum of fates. One of the earliest discoveries along this line demonstrated the As noted below, the real spectrum of SCP-derived fates is much wider existence of SCP-derived melanocytes, which are pigment cells and includes cells of the mesenchymal nature. To the best of our (Adameyko et al., 2009, 2012). In fact, melanocytes inhabit not only knowledge, some dental mesenchymal progenitors and dental me- skin but also many other locations in the body, such as heart (Levin senchymal stem cells (MSCs) in mouse originate from the local facial et al., 2009; Yajima and Larue, 2008), inner ear (Steel and Barkway, innervation (Kaukua et al., 2014). Similarly, specific neural crest- 1989) and brain meninges (Goldgeier et al., 1984). Melanocytes derived mesenchymal populations of murine bone marrow arrive at derived from the local innervation can explain how these internal their final destination in the body via an intermediate stage of locations become populated by pigment cells. In addition, the most peripheral nerve-associated progenitors (Isern et al., 2014). Even deadly extra-cutaneous melanoma tumors originate from an unknown murine endoneurial fibroblasts split from their immediate progenitors, source and do not even manifests themselves at the skin levels which are located inside of the peripheral nerves (Joseph et al., 2004). (Hussein, 2008). SCP-derived melanocytes might be the origin for Taken together, this information highlights the remarkable plasticity of such melanoma subtypes. SCPs in the developing body (Fig. 1B). To date, nerve-derived melanocytes were identified in mammalian (Adameyko et al., 2009, 2012), bird (Nitzan et al., 2013) and fish 1.3. Plasticity of the Schwann cell lineage evokes evolutionary development (Kelsh and Barsh, 2011). For instance, the postmeta- discussions morphic melanophores in zebrafish originate from nerve-associated glial progenitor cells (Dooley et al., 2013; Kelsh and Barsh, 2011; Consistent with their name, SCPs generate all types of peripheral Parichy and Spiewak, 2015; Singh et al., 2016). Therefore, taking such glial cells found in the adult body, including myelinating, non- nerve-associated progenitors of pigment cells into account might help myelinating (Remak) and terminal Schwann cells, as well as other to resolve the enigma of pigmentation patterns. not well-studied subtypes, including specialized glial cells in various The local recruitment of SCPs to generate fates that were previously sensory skin end organs. Differentiation of SCPs into Schwann cells, considered as direct neural crest derivatives can be explained by the their largest derivative, includes a transitory state of an immature benefits of having a long-term supply of neural crest-like cells any- Schwann cell and partly resembles differentiation of oligodendrocytes where in the fast-growing body. Thus, the developmental constraints in the central nervous system (reviewed by Kastriti and Adameyko, related to the transiency and migration capacity of the neural crest can 2017). Such similarity stimulated discussions about the evolutionary be eliminated. A classic example of the implementation of such strategy connections between CNS and PNS glial cells types. For instance, it is is the discovery of SCP-derived parasympathetic neurons (Dyachuk possible that the gene expression module responsible for myelination et al., 2014; Espinosa-Medina et al., 2014). These autonomic neurons evolved primarily at the periphery of the body to provide faster develop proximal to the organ they typically innervate. Thus, their transmission of sensory and motor signals in animals, increasing their developmental timing is connected to the development of such organs. size. If so, the module could have been later co-opted into the CNS to Neural crest migration occurs at considerably earlier timepoints when increase the speed of complex computations. Adult Schwann cells do the embryos are small and the primordia of many such organs (for not exhibit any stem-like populations to the best of our knowledge and instance, glands) are not even in place yet (between E8.5 and E9.5 in instead rely on processes resembling de-differentiation in case of nerve mouse embryo). Indeed, mouse nerve-associated SCPs generate para- damage (Jessen and Mirsky, 2016). In such cases, mouse adult sympathetic ganglia after E12.5 (Dyachuk et al., 2014; Espinosa- Schwann cells located at the site of nerve damage can generate Medina et al., 2014). This finding also suggests another interesting pigmented spots and have been confirmed to be the origin (both notion of developing neuronal connectivity wherein the presynaptic myelinating and non-myelinating subtypes) of local melanocytes fibers bring progenitors of the postsynaptic neurons that will be (Adameyko et al., 2009; Rizvi et al., 2002). Interestingly, in continu- innervated by the fibers of the progenitor delivery system once formed. ously growing mouse incisors, adult peripheral glial cells are periodi- This is a self-growing neuronal network, where the placement of new cally recruited into the dental mesenchymal stem cell niche, giving rise postsynaptic neurons in the body is dictated by the navigation of to large amounts of odontoblasts and pulp cells during the mouse peripheral presynaptic nerves. The development of such a self-growing incisor tooth self-renewal process (Kaukua et al., 2014). Similarly, network can be predicted inside of the expanding murine enteric extrinsic nerves with associated SCPs are capable of re-supplying the nervous system, where Schwann lineage cells generate neurons in the postnatal growing gut with progenitors of enteric neurons (Uesaka gut during postnatal neurogenesis according to Uesaka and coauthors et al., 2015), whereas trauma to the mature enteric ganglia induces the (Uesaka et al., 2015). According to this study, a subset of SCPs invades differentiation of resident glial populations into neuronal components the growing gut via extrinsic innervation of the intestine and gives rise (Laranjeira et al., 2011). to approximately 20% of all neurons with a bias towards the neurons of Given that SCPs generate a wide variety of fates others than those some specific subtypes (for instance, calretinin-expressing cells). The related to adult Schwann cells (see Fig. 1 for an overview of SCP- population of SCPs that gives rise to these neurons is physiologically derived cell types), it seems logical to question whether the historical important given that genetic ablation of Ret in SCPs results in colonic term SCP clearly describes the nature of these multipotent embryonic S26 A. Furlan, I. Adameyko Developmental Biology 444 (2018) S25–S35 Fig. 1. Migratory neural crest and nerve-associated Schwann cell precursors represent a long-lasting source of multipotent progenitors available in any body location due to diversification of dissemination strategies. Outline of dissemination routes in the mouse embryo: E8.5-E10 NCCs (A) and E12.5-onwards nerve-dependent SCPs (B). NCCs and SCPs form most of the peripheral nervous system elements and other non-neuronal cell types (shown on the right). NCCs: neural crest cells; SCPs: Schwann cell precursors; MSC: mesenchymal stem cells. progenitors. Nerve-associated multipotent progenitors (NAMP) or Sommer, 2012). Therefore, SCPs can be considered as a relatively slow neural crest-like nerve-associated cells (NCLNAC) represent terms that and fluent continuation of a developing neural crest population. would better describe these cells. All of these cumbersome names have Alternatively, early SCPs can be perceived as nerve-associated neural a major disadvantage: they break the familiar connection between the crest cells that gradually become fate-restricted and adapt to life at the existing literature and the actual embryonic cell type. Therefore, we nerve surface in a more advanced embryo. support keeping the old name as long as nothing strikingly better is Indeed, there is no clear definition based on molecular markers that suggested. could define the entire neural crest cell population. These cells continuously transform following their developmental timeline from 2. Transiency of neural crest cells and SCPs: cells in the flow a pre-migratory state until differentiation and differ based on the anterior-posterior origin (Kuo and Erickson, 2010). Studies in murine Nothing is stable during the course of development. Cell lineages and avian models demonstrated that Sox10 and FoxD3 expression does actively progress, and their terminal branches undergo commitment not discriminate these cells from SCPs that are associated with nerves and final differentiation. In line with this concept, neural crest cells are (Adameyko et al., 2009; Britsch et al., 2001; Mundell and Labosky, not uniform when it comes to different timepoints or even locations 2011; Thomas and Erickson, 2009; Wahlbuhl et al., 2012). However, in given that vertebrate development is not synchronized along the boundary cap stem cells (if considered an SCP subtype) located at the anterior-posterior axis (Kuo and Erickson, 2010). Premigratory neural nerve root entry and exit points, we observe a number of distinct crest cells are generated inside of the dorsal neural tube and soon markers that are upregulated in mice, including the transcription factor delaminate via the epithelial-to-mesenchymal transition (Fig. 1A). This Krox20 (Egr2) (Coulpier et al., 2009; Maro et al., 2004). Similarly, no process involves a dramatic change in gene regulatory network clear molecular-based definition exists for SCPs because they also composition and effector module gene expression programs. change from the moment when the neural crest associates with the Delaminated cells actively migrate diversifying along different routes innervation until the moment when SCPs exhibit immature Schwann until they “settle” in specific anatomical structures, such as sensory or cell markers (Kastriti and Adameyko, 2017). However, there are autonomic ganglia, skin, anterior face, branchial arches or peripheral markers and milestones of the developmental progression from the nerves (Duband, 2006) (Fig. 1A). It is indeed difficult to draw a border neural crest to SCPs and from SCPs towards mature Schwann cells. defining what cells remain neural crest cells and those that are not. In Regarding the neural crest, these markers indicate the premigratory particular, this information is relevant to the transition between neural state, delamination, migration and commitment to different fates crest cells and SCPs. Formally, we can assume that neural crest cells (Bronner, 2012; Kalcheim and Burstyn-Cohen, 2005). become SCPs when they associate with peripheral nerves (Fig. 1B). Regarding SCPs, these markers eventually include more Schwann However, this is very shaky reasoning given that we are not aware of cell-specific genes. For instance, BFABP becomes apparent in the any gene expression or functional change clearly demarcating this nerves of the cranial region as early as E10.5 and is minimally transition. In contrast to neural crest cells that enter neurogenesis, expressed in the trunk (Adameyko et al., 2012). For instance, in mouse undergo melanocyte differentiation, or generate the ectomesenchyme embryos, the first SCP cells form at E9.5-E10. At E12.5-E13.5, SCPs of a head, the transition of neural crest into SCPs does not involve a start to exhibit the expression of several myelination program-asso- dramatic phenotypical change to the best of our knowledge (Dupin and ciated genes despite the fact that it is too early for these cells to become S27 A. Furlan, I. Adameyko Developmental Biology 444 (2018) S25–S35 immature Schwann cells (Furlan et al., 2017). These data suggest the mouse, deletion of Phox2b completely aborts sympathetic (and para- eventual commitment and “solidification” of glial fate. Consistently, sympathetic) development programs, whereas the absence of Ascl1 in SCPs produce less of the non-glial progenies with progressing devel- autonomic progenitors reduces proliferation and induces apoptosis. opmental timing. Lineage tracing reveals that mouse SCPs almost stop Similar to the development of sympathetic neurons, namely immediate generating parasympathetic neurons or neuroendocrine cells at E15.5 neural crest derivatives, Phox2b and Ascl1 expression is initiated in (Dyachuk et al., 2014; Furlan et al., 2017). Although this finding may SOX10+ SCPs associated with cranial nerves (Dyachuk et al., 2014; not be associated with the intrinsic capacity of developing nerve- Espinosa-Medina et al., 2014). Consistent with this finding, the associated cells to differentiate into other fates, this information is deletion of Ascl1 prevents SCPs from becoming autonomic parasympa- rather supportive based on the above reasoning. thetic neurons (Dyachuk et al., 2014). The cues triggering Ascl1 and What makes a neural crest cell (NCC) a Schwann cell precursor Phox2b expression exclusively in some discreet locations along the (SCP)? What mechanisms drive an SCP into a mature Schwann cell or nerves are unclear, but we can assume that local sources of BMPs and another cell type? Although we still do not fully understand this CXCL12 might be involved (Saito et al., 2012). process, we do know about some major signaling pathways that govern Cell-lineage tracing and functional experiments performed in mice these progenitor's fate decisions. have demonstrated that cross-regulatory talk between SOX2 and MITF Schwann cell development, survival and lineage progression relies transcription factors is involved in the transition of SCPs towards glial on Nrg1 and Notch signaling pathways. Neuregulins are supplied by or melanocyte fates. SOX2 prevents Schwann cells from undergoing the the axons and bind to receptors of the ErbB family of receptor tyrosine melanocyte path by directly binding and repressing Mitf regulatory kinases, which are located on Schwann cell membranes. Mammals regions (Adameyko et al., 2012). SOX2 interacts and cross-represses have four Neuregulin genes, Ngr1–4, which all signal via an epidermal with KROX20 (Le et al., 2005), which guides the transition from growth factor (EGF)-like extracellular domain. In PNS nerves, ERBB2 immature to myelinated Schwann cells. SOX2 down-regulation is and ERBB3 dimers form a complex on the surface of Schwann cells. crucial for SCPs to mature either into Schwann cells or melanocytes. The extracellular signaling domain of NRG1 type III binds and Upon detaching from cranial nerves, newly formed melanocytes activates this complex, allowing for axon-glia communication. The migrate laterally towards the ectoderm and proliferate. Endothelin more NRG1 an axon secretes, the more myelin sheaths its Schwann cell and Kit pathways have been proposed to play a role in further will deposit. Consistent with this notion, experiments using mutant instructing these cells. Ablation of the EDNRB ligand EDN3 in vivo mice lacking one allele of Nrg1 or overexpressing Nrg1 have less and disrupts melanocyte migration pathways and reduces their numbers in more myelin, respectively, associated with their axons (Michailov et al., mice (Adameyko et al., 2012). Similar to neural crest-derived melano- 2004). cytes, mutations affecting the Kit pathway cause reduced numbers of Another main pathway driving Schwann cell lineage progression is nerve-associated melanocytes (Dooley et al., 2013). Notch. The key elements of the Notch pathway, the ligand JAGGED1 and its receptor NOTCH1, are expressed by murine neurons and nerve- 3. SCPs, neural crest and boundary cap stem cells–diversity associated Schwann cells, respectively (Woodhoo et al., 2009). within limited borders Although SCP differentiation from neural crest cells does not involve Notch signaling (Taylor et al., 2007; Woodhoo et al., 2009), in vivo SCPs are heterogeneous and might include several subpopulations deletion of Notch1 or of Notch transcriptional mediator RBPJ in SCPs with different properties and markers. For instance, boundary cap (BC) causes a delay in the emergence of Schwann cells and decreased stem cells (Radomska and Topilko, 2017) and embryonic satellite glial proliferation. In contrast, its activation accelerates this program by cells (Dublin and Hanani, 2007; Hanani, 2005; Huang et al., 2013; maintaining high levels of ERBB2 receptor and consequently boosting Pannese, 2010) might be considered as subtypes of SCPs given that NRG1 signaling. Notch inhibits myelination and is involved in de- they share many common markers (SOX10, FOXD3, and others) and myelination of adult nerves, whereas KROX20 inhibits Notch and largely perform supportive functions in the peripheral nervous system. thereby the timing of the myelination process (Woodhoo et al., 2009). BC cells are neural crest-derived. Soon after neural crest migration, During nerve injury, Ephrin and TgfB pathways play a fundamental they settle at the entry and exit points of peripheral nerves crossing the role in de-differentiating Schwann cells and facilitating axonal regen- central/peripheral nervous system border (Fig. 2A). BC cells function eration. In mouse, following nerve injury, Schwann cells and fibroblasts as repulsive plugs by expressing navigation molecules preventing CNS are located at the borders of the injury site, and their interactions direct cells from leaking outside of the developing neural tube (Coulpier et al., the migration of de-differentiated Schwann cells into the stump. 2010). As mentioned in a previous paragraph, mouse BC cells express Ephrin-B2 (EFNB2) secreted by fibroblasts binds the EPHB2 receptor Egr2 (Krox20), Prss56 and other specific markers (Coulpier et al., on Schwann cells, causing progenitor-like cells to form cords of cells 2009). BC cells exhibit exceptional multipotency (which might be a migrating into the stump. Mechanistically, this signaling pathway general property of the neural crest and SCP populations), which regulates SOX2-dependent N-cadherin re-distribution (Parrinello results in BC-derived sensory neurons, satellite cells and specific et al., 2010) that guides axonal regrowth. More recently, it was shown sensory nerve fiber-associated glia in skin (Aquino et al., 2006; that Schwann cell reprogramming into progenitors and their migration Gresset et al., 2015; Maro et al., 2004; Zujovic et al., 2011). The in the wound is dependent on TGF-beta-EPHB2 crosstalk (Clements immediate progeny of BC cells blend with other nerve-associated SCPs, et al., 2017). embryonic satellite cells, and neurons and actively migrate along the We have very little insight into the similarity of the fate-specifying sensory nerves towards the skin, where they give rise to highly mechanisms between migratory neural crest cells and nerve-associated specialized glial cells supporting the fine ends of nerve fibers in the SCPs. Migrating neural crest cells are exposed to multiple cues epidermal layer (Gresset et al., 2015). released, for instance, from the forming spinal cord. These signals Satellite glial cells (SG cells) are located inside of the peripheral are believed to trigger a transcriptional cascade that leads to the nervous system ganglia and may perform various unknown functions formation of the heterogeneously composed sensory ganglion (for during early post-neural crest development (Hanani, 2005). Data review, please see Marmigere and Ernfors, 2007). Others NCCs migrate suggest that SG cells are involved in the development of pain (Costa ventrally and coalescence by the dorsal aorta to generate the sympa- and Moreira Neto, 2015; Dublin and Hanani, 2007). In rats, excited thetic part of the autonomic nervous system. Members of the Bone sensory neurons (somas) communicate with SG cells via the release of Morphogenic Protein (BMP) family direct the expression of key ATP that activates P2×7 receptors in SG cells, which subsequently transcription factors involved in autonomic nervous system develop- release TNF-alpha and potentiate DRG neuron excitability (Zhang ment (Muller and Rohrer, 2002), including PHOX2B and ASCL1. In et al., 2007). Additionally, these cells can undergo local neurogenesis in S28 A. Furlan, I. Adameyko Developmental Biology 444 (2018) S25–S35 Fig. 2. Hypothetical evolutionary scenario explaining the potential mechanism of the exit of proto-neural crest cells from the central nervous system along nerve entry and exit points as an alternative strategy to delamination. (A) Boundary cap cells (BC) are neural crest-derived cells located at the CNS entry and exit points (dorsal root ganglion (DRG) afferents in the upper spinal cord and motor neurons in the ventral spinal cord, respectively). BCs prevent CNS elements from migrating outside the developing spinal cord (“STOP” sign) as demonstrated by the genetic ablation of the BC-key transcription factor Krox20 leading to migration of CNS cells outside of the neural tube (“GO” sign). (B) Before the elaboration of the BCs, in a hypothetical cephalochordate, this migration route could have been used for transporting progenitors from the CNS to the periphery of the body. response to peripheral nerve trauma (Muratori et al., 2015). Molecular representative model system) (Flood, 1966; Peters, 1963). Indeed, determinants clearly discriminating SG cells from other peripheral glia Amphioxus is one of the most basal chordates that does not have neural subtypes are currently unknown. These cells express classical markers crest cells (at least the traditional crest originating inside of the dorsal of SCPs, including Sox10, FoxD3, S100b and others. Interestingly, neural tube) (Holland and Holland, 2001; Yu et al., 2008). However, murine satellite glial cells do not rely on the NRG-ERBB signaling axis the function and origin of the rare nerve-associated cells, as well as as much as other SCPs do. In Erbb3 knockout models, satellite cells their homology to neural crest-derived glia from vertebrates, are not survive inside of the dorsal root ganglia, whereas most of the nerve- known. Whether these glia-like cells are of mesodermal origin or associated SOX10+ SCP cells disappear (Adameyko et al., 2009). emerge from the neural tube at the nerve entry and exit points is In mouse, nerve-associated PHOX2B+ SCP glial cells are located unclear. along specific branches of the cranial nerves and inside of the enteric Unlike cephalochordates and tunicates, cyclostomes possess well- nervous system (Dyachuk et al., 2014; Espinosa-Medina et al., 2014). developed neural crest cells that migrate out of the dorsal neural tube The expression of Phox2b in SOX10+ SCPs might be required to (Green and Bronner, 2014). As expected in the case of a creature facilitate their conversion into PHOX2B+ autonomic neurons. possessing a neural crest, these animals (currently living lamprey and SCPs associated with nerves express different amounts of Sox2 hagfish) have nerves fully covered with glia (Peters, 1960) although depending on their position along the nerve. SOX2 levels are gradually they do not exhibit any myelination (Bullock et al., 1984). Therefore, reduced in cells located further away from PNS ganglia or from CNS lamprey and hagfish are the most ancient and accessible models for the exit/entry points towards the periphery. Functional experiments analysis of neural crest evolution in connection with a Schwann cell revealed that SOX2 is likely biasing against melanocyte fate given that lineage. Strikingly, lampreys possess peripheral glial cells that retain Sox2 and melanocyte master regulator MITF demonstrate efficient crest-like multipotency. For instance, their enteric neurons are gener- mutual cross-repression. Overexpression of Sox2 in melanoblasts or ated from Schwann cell precursors associated with the nerves that their SCP-based progenitors blocks the development of the melanocyte innervate the developing gut (Green et al., 2017). This mechanism is fate (Adameyko et al., 2012). conceptually similar to that observed in mammals, where the enteric Thus, SCPs (or, in other words, neuron-associated glial cells in the neurons can be generated by the SCPs invading the developing mouse PNS) are quite diverse despite sharing many common features. Further gut with ingrowing extrinsic nerves (Uesaka et al., 2015). Thus, already investigation involving the unbiased analysis based on single-cell in agnathans we observe neural crest-like cells navigating the nerves transcriptomics might reveal more subpopulations of SCPs with and spreading throughout the body in a manner similar to gnathos- different properties and functions. tome SCPs. The comparative analysis of a neural crest progeny in lampreys and other animals suggested that the evolution of the crest progressed incrementally by adding additional fates to the spectrum 4. Intertwined evolution of neural crest cells, CNS glia and that was initially represented by a few fates (Baker, 2008; Hall and SCPs: the chicken or the egg? Gillis, 2013). If this is indeed the case, the Schwann cell lineage is among the most ancient fates if not the primary fate generated by the The evolution of peripheral glial cells is not widely discussed in the proto-crest. Given that SCPs and the neural crest share so many scientific literature (Kastriti and Adameyko, 2017) despite being common properties and might be equally ancient, we ask whether we intimately linked to the evolution of the neural crest (Ivashkin and clearly understand that SCPs evolved from the neural crest and not vice Adameyko, 2013). Rare scattered nerve-associated cells were pre- versa. Currently, we need to perform further work to unambiguously viously described in cephalochordates (with Amphioxus serving as a S29 A. Furlan, I. Adameyko Developmental Biology 444 (2018) S25–S35 answer this question. to ensure cellular sources during late embryonic development or metamorphosis. In any case, if we consider other vertebrate crown 4.1. Evolution of dissemination strategies in the multipotent proto- groups, including birds and mammals, both methods of spreading the neural crest lineage neural crest and multipotent neural crest-like cells in an embryo are observed: free migration and via the peripheral nerves (Furlan et al., The developmental logic of SCPs allows prolonging the existence of 2017; Nitzan et al., 2013). The existence of both strategies of multi- the multipotent neural crest pool by inhabiting a long-lasting niche in potent progenitor cell dissemination can provide better flexibility and the nerves over a long course of development and growth, whereas some degree of redundancy of the cellular sources for the sake of robust migratory neural crest cells are transient and are limited by the short and plastic development. Thus, during prehistorical times, it is possible distance of the free migration complicated by the complex navigation that early proto-neural crest cells could undergo initial short-range free required for every major direction of differentiation. As we will see migration that was followed by long-distance dispersal via the innerva- below, the benefits of the nerve-associated dispersion could be tion. During further evolutionary transformations, these “phases” of theoretically achieved without the capacity of CNS cells to delaminate neural crest dissemination could change quite dramatically to adapt to from the dorsal aspect of the neural tube. the size of the embryo, developmental heterochrony and the speed of For instance, in case of the impaired boundary cap (BC) stem cells growth. From this perspective, multipotent SCPs would reflect the later (via knockouts of Krox20 or Semaphorin6a), the immature neurons developmental phase of such dispersal and might be at least as and other CNS progenitors leak out of the neural tube towards the evolutionary ancient as a freely migrating neural crest. periphery from the entry and exit nerve points (Mauti et al., 2007; Vermeren et al., 2003) (Fig. 2A). More importantly, progenitors that 4.2. Evolutionary insights from comparing the CNS and PNS leak from the CNS in the absence of proper BC cells are capable of populations in various animal groups myelinating motor and sensory nerves extending outside of the CNS (Kucenas et al., 2008; Zujovic et al., 2010). This phenomenon suggests The embryonic CNS zoo has a limited diversity of cell types when a plausible evolutionary scenario where ancient CNS progenitors could compared with modern neural crest progenies. Nevertheless, there are emigrate from the CNS in the absence of delamination capacity via the cell types that demonstrate striking similarities: CNS and PNS neurons incoming and outgoing nerve tracks via a mechanism similar to that in (despite differences in their particular subtypes) or oligodendrocytes embryos lacking BC cells (Fig. 2B). This notion imposes new interesting and myelinating Schwann cells. The gene expression programs of questions about when the boundary cap population evolved for the first oligodendrocytes and Schwann cells, their myelinating capacity and time and what it meant for the evolution of the neural crest (including some other features related to the dynamics of their development point their capacity to delaminate and migrate freely). Given that the dorsal towards their evolutionarily related nature either at the basis of co- root entry points are located proximally to the neural crest domain, it is option of specific programs or based on a deep homology given that possible that the cells that were previously leaking through these points both types initially originate in the CNS (in case of Schwann cells via could in principle “invent” delamination and free migration during neural crest and SCP intermediates), as recently reviewed by (Kastriti further evolution. and Adameyko, 2017). Consistent with this logic, myelination of the Taken together, the earliest proto-neural crest cells in a common central and peripheral nervous system components evolved around the ancestral state could spread following the peripheral nerves that same time, namely, sometime after the cyclostomes split from the provided a niche and a navigation route after the progenitors were common ancestor with other vertebrates (Bullock et al., 1984). Indeed, leaked from the nerve root entry points (see Fig. 2 outlining these neural crest-derived peripheral glial cells could be the pioneering (or concepts). In that sense, such proto-neural crest cells could be more even proto-crest) fate during neural crest evolution. similar to SCPs rather than free migrating modern crest cells. Not only Schwann cells and oligodendrocytes can be considered as Following the same logic, the multipotency of SCPs might be con- related cell types (at least at the level of gene expression programs). In sidered as an archetypical (if not atavistic) feature, whereas the free addition, as pigment cells, melanocytes exhibit evolutionarily relevant migration of a traditional crest could be an easily achievable innova- counterparts dwelling inside of the CNS, namely, the retinal pigmented tion. epithelium of vertebrate eyes (Martinez-Morales et al., 2004). For instance, such innovative free migratory behavior of CNS Similarly, we can compare CNS-residing pigmented Hesse ocelli from progenitor cells can be artificially triggered by the ectopic expression Amphioxus (Castro et al., 2006) and pigmented cells from the tunicate of Twist, as shown in experiments performed in a tunicate embryo CNS (Abitua et al., 2012) to the modern vertebrate melanocytes (Abitua et al., 2012). Even in the absence of artificial reprogramming, especially because melanocytes were recently shown to be photosensi- neuronal progenitors can delaminate from the ascidian larval neural tive in the same manner as classical photoreceptors. Indeed, melano- plate border, migrate short distances and generate specialized afferent cytes and melanophores from different vertebrate groups express neurons at the periphery (Stolfi et al., 2015). melanopsins and members of the phototransduction cascade that are Importantly, the evolution of the nerve-associated migration or the functionally active and trigger physiological responses to the visible free migration should not be considered equivalent to the evolution of light and UV (Isoldi et al., 2005; Ivashkin and Adameyko, 2013; the neural crest as a cell type. The dissemination strategy is only a Provencio et al., 1998; Wicks et al., 2011). The similarity of neural property of the neural crest or SCP cells. Such a property may or may crest-derived melanocytes to these CNS-residing pigmented photosen- not be connected to the evolution of other key properties, such as sory cells suggests the possibility of some intertwined or common neural crest fate induction, specification and multipotency, that origin related to the emergence of the multipotent crest. collectively comprise the nature of a so-called neural crest cell. We previously suggested a new evolutionary scenario where the However, the capacity of CNS-born progenitors to migrate into the formation of the proto-neural crest occurred as a consequence of intra- body periphery via the nerve routes could potentially catalyze further CNS pigmented photosensory structures being repositioned to the evolutionary transformations, leading to the assembly of gene expres- periphery of the body (outside of the CNS) for better light detection. sion programs necessary for modern neural crest formation via a series This arrangement could occur via formation of migratory nerve- of incremental steps. associated multipotent progenitors (Ivashkin and Adameyko, 2013). Of course, the proposed scenario is highly speculative given that it This transformation requires the formation of an intermediate multi- is possible that free migratory neural crest-like cells evolved first. Then, potent progenitor cell that could exit the CNS to form the external during the evolutionary body size increase, the option to maintain photosensory organ in sub-epithelial locations. Such a multipotent cell neural crest-like progeny along the nerves could subsequently be added potentially possessed the ability to generate sensory neurons and glial S30 A. Furlan, I. Adameyko Developmental Biology 444 (2018) S25–S35 cells as well given that the embryonic/larval neural tube of Amphioxus the vestibulocochlear nerve (the 8th cranial nerve) and causing hearing consists of very few cells compared with other animals. Thus, the low problems (Lanser et al., 1992). Schwannomas are very common in cell number in the Amphioxus neural tube could be compensated by patients with neurofibromatosis, particularly types I and II, given that their late proliferative activity, multipotency and shared lineage. these tumors are generally composed of Schwann cell derivatives. At Emigration of one or two cells at the early stage could relocate the the same time, type I neurofibromas (NF) are heterogeneous in entire domain of the progeny beyond the borders of the CNS. With composition and may contain fibroblasts, mast cells, perineural cells time, the visual function of these external ocelli would diminish, and melanocytes. uncovering additional roles for the pigmentation, namely camouflage, Neurofibromatosis type 1 is a multisystem genetic disorder caused pigmentation pattern-based signalization and UV protection. This by a mutation in the Neurofibromatosis-Related Protein NF-1 (Nf1) scenario favors peripheral glial (SCP-like progenitors of melanocytes) gene. Mutations in the Nf1 gene can inflict a wide range of defects in and photosensory pigmented fates (melanocyte-like) as primary evolu- neural crest-derived tissues (Cichowski and Jacks, 2001) and predis- tionary developments during the early steps of neural crest elaboration. pose patients to a variety of cancer types. Neurofibromas, which are Later, the fate repertoire could be enriched by adding more neuronal typically benign tumors, are classified as dermal or plexiform. The subtypes and other cell type variations. It is possible that such proto- dermal variant typically manifests around adolescence as hyper- neural crest cells left the CNS via the sensory or motor nerve exit pigmented patches on the skin (café-au-lait macules), which increase points, spread along the corresponding nerves and were subsequently in number with age. Plexiform NFs occur in 20–40% of patients. Unlike recruited to specific innervated destinations. Thus, this hypothesis does dermal NF, which is superficial and benign, plexiform NFs form along not contradict the notion of primary nerve-associated dissemination of peripheral nerves and preferentially affect the para-spinal area asso- the proto-neural crest. ciated with the dorsal root ganglia (DRG). These plexiform masses can grow to large tumors and become malignant. The initiation of such tumors has remained elusive; however, the origin cell clearly belongs to 5. Therapeutic perspectives and importance of peripheral the peripheral glial lineage. Recently, an elegant and detailed mouse glia plasticity for human health study addressed the known embryonic Schwann cell subgroups and demonstrated that PLP1+/GAP43+ cells are the main source of NF A number of neurocutaneous diseases likely involve Schwann cells tumors (Chen et al., 2014). Consistently, the deletion of murine and their progenitors, exploiting their plasticity in a manner similar to transcription factor Runx1 from SCPs delays NF formation in vivo the neural crest, including neurofibromatosis type I, leprosy, melano- (Li et al., 2016). mas, and pigmentation abnormalities, such as nevus of Ota and the so- Approximately all NF patients display pigmented patches on the called “Mongolian spot” (Fig. 3). Moreover, recent findings suggested skin called café-au-lait macules together with the presence of abundant that cancers, such as neuroblastoma and pheochromocytoma, may melanocytes within actual tumors. These features are indicative of a originate from nerve-associated progenitors during development or in strong association among Schwann cell progenitors, melanocytes and adulthood. cancers derived from these cell types. Consistent with this notion, Cre- Schwannomas are mostly benign tumors growing on the surface of mediated deletion of the Nf1 gene specifically in peripheral glial cranial and other peripheral nerves. Although Schwannomas can progenitors (after neural crest cells completed their migration and appear on virtually any nerve, approximately half of cases occur in differentiation) leads to the formation of NF tumors associated with the head and neck region (Biswas et al., 2007). In humans, the most pigmentation (Wu et al., 2008), as extensively reviewed in (Adameyko common variant is represented by the vestibular Schwannoma affecting Fig. 3. Human pathologies associated or likely associated with abnormalities of peripheral glial lineage involving melanocyte-related (i.e., nevi, melanomas, and nevus of Ota) or sympathoadrenal-related (i.e., paraganglioma, pheochromocytoma, and neuroblastoma) cases or stemming directly from the glial cells compartment (i.e., neurofibromatosis, Schwannomas, peripheral neuropathies, and leprosy). S31 A. Furlan, I. Adameyko Developmental Biology 444 (2018) S25–S35 and Lallemend, 2010). Further proof of the association between SCPs hierarchy of these new chromaffin progenitor cells may underlie some and melanocytes includes the fact that nevi and melanoma cancer cells specific properties of particular subtypes of neuroblastoma and pedia- express Schwann cell markers in mice and humans (Aso et al., 1988; tric pheochromocytoma. Future work should address the potential of Iwamoto et al., 2001; Warner et al., 1981). Conversely, melanotic these glial and bridge nerve-associated progenitors in the generation of schwannomas, a group of tumors originating presumably from various tumors. Schwann cells, express melanocyte markers (Er et al., 2007). The plasticity of adult and embryonic peripheral glial cells is Nevi, or pigmented moles, are localized benign skin lesions beneficial to our body during the nerve damage by transforming appearing after birth and affecting almost everyone. However, some Schwann cells into reparative cell types (Boerboom et al., 2017; Han nevi can become a melanoma, a malignant cancer of the skin with an et al., 2017). Consistent with this notion, growing evidence suggests increased incidence worldwide (Erdmann et al., 2013). Melanocytes that Schwann cells give rise to specialized cells that inhabit skin were considered to be immediate derivatives of migratory neural crest wounds and promote the wound closure and healing via a paracrine cells (Kawakami and Fisher, 2011; Sommer, 2011) until it was modulation (Johnston et al., 2013; Parfejevs et al., 2018). demonstrated that they can originate from SCPs associated with the Such regenerative plasticity of the Schwann cell lineage can be growing nerves (Adameyko et al., 2009). In mouse, abnormalities in harnessed as an advantage by pathogens. this mechanism might be responsible for conditions, such as extra- In humans, an example of this phenomenon involves cutaneous melanomas (and some other melanoma subtypes), the nevus Mycobacterium leprae infection of Schwann cells, resulting in a of Ota and the so-called “Mongolian disorder”. The nevus of Ota is a condition known as leprosy or Hansen's disease. Recently, extensive disorder where melanocytes form massive blue/gray patches that are research performed in mouse models revealed that M. leprae highjacks deposited along the first and second branches of the cranial trigeminal the molecular program of mature Schwann cells and reverts them to nerve, demonstrating a clear connection with neuroanatomy. Although the stem-like mesenchymal state by turning off adulthood Schwann primarily affecting the skin, extracutaneous spreading to the eye sclera genes and turning on the mesodermal-like developmental program is frequent (Radhadevi et al., 2013) (Fig. 3). A similar condition is the (Masaki et al., 2013). Such de-differentiated Schwann cells can so-called “Mongolian spot”, which is characterized by the appearance proliferate, form granulomas (skin bumps) and even migrate along of blue/gray spots predominantly in the lumbosacral area at birth. the nerves to other body locations. Indeed, the fact that mature Although these spots disappear relatively soon after birth in most Schwann cells can be reprogrammed and reverted to a specific cases, metabolic abnormalities have been reported in affected people progenitor state by a bacterium is surely striking. However, in the case (Gupta and Thappa, 2013). In light of this notion, a better under- of Hansen's disease, the re-derived progenitors form only highly standing of the developmental dynamics is necessary for the clear contagious aberrant masses of tissue (Fig. 3). What would occur if we identification of the origin cell for such pathologies, thereby providing could de-differentiate adult Schwann cells and then re-direct them into new potential targets. the formation of new desirable cell types for regenerative purposes? Recently, new data demonstrated that chromaffin cells of the Schwann cells are found everywhere in the body along the nerves, adrenal medulla are derived from SCPs during murine embryo devel- functioning as a generous and almost omnipresent cell source that can opment via a newly identified progenitor cell expressing markers of be instructed and reprogrammed in vivo. On the other hand, devising both glial and chromaffin (sympathoadrenal) lineages and thus named strategies to culture and expand these cells in vitro for the purpose of “bridge” cell (Furlan et al., 2017). The sympathoadrenal lineage is the transplantation is of crucial importance. Schwann cells and their source of neuroblastoma, the most common extracranial solid tumor of progenitors can be obtained in large numbers from induced pluripotent early childhood (Maris et al., 2007), and pheochromocytoma, which stem cells (IPSCs) (Kim et al., 2017; Ma et al., 2015). Unlike embryonic originates from rather mature chromaffin cells mostly during adult life stem cells (ESCs), ISPCs can be derived from a patient's own somatic (Turchini et al., 2018). Consistent with this notion, neuroblastoma is cells (autologous), differentiated into SCPs and transplanted back into thought to form from proliferating sympathetic nervous system pre- patients. In vitro, newly derived SCPs become mature functional cursors during development, whereas pheochromocytomas are consid- Schwann cells, secreting neutrophins and myelinating rat axons. ered to stem from mature chromaffin cells after embryonic develop- When transplanted in vivo, human pluripotent stem cells (hPSCs) ment is completed (due to their late onset despite the existence of rare facilitate axonal regeneration (Kim et al., 2017). Additionally, avian pediatric subtypes). In humans, neuroblastomas and pheochromocy- (quail) Schwann cells and their progenitors expanded in vitro can tomas are clinically heterogeneous (Burnichon et al., 2016; Chicard generate other cell types, including myofibroblasts (Real et al., 2005) or et al., 2017). The factors that define such a spectrum of tumor subtypes melanocytes (Dupin et al., 2003), in a dish. and their variable behaviors are unclear; however, some important Taken together, many recent studies described remarkable steps differences can result from the properties of a cell of origin. forward in understanding SCPs and Schwann cells in health and Chromaffin cells have long been considered to be a product of disease (summarized in Fig. 3). In particular, SCPs are now considered common sympathoadrenal precursor cells subsequently derived from to be a potential source of other cell types in addition to mature neural crest stem cells migrating dorso-ventrally towards the dorsal Schwann cells. The advent of the single cell transcriptomics era aorta. According to this notion, this common precursor pool later splits considerably improved our ability to gather data about molecular and generates the sympathetic lineage and the chromaffin precursors in changes during development or regeneration and enabled an unprece- a rather ventral position in mouse and chick (Huber et al., 2009; Saito dented degree of exploration. In particular, the identification of a new et al., 2012). Rather unexpectedly, a number of experiments, including nerve-associated cell, the so-called “bridge” progenitor between SCPs lineage tracing and single-cell sequencing of SCPs, bridge and chro- and mature chromaffin cells, forces the community to reconsider what maffin cells, revealed a progressive transition between cell types. In- we previously thought about the normal development of sympathoa- depth molecular and bioinformatics analysis revealed that after an drenal tissues and corresponding developmental abnormalities leading initial step where SCPs proliferate to expand their pool, they transiently to the onset of life-threatening conditions, such as neuroblastoma and halt cell division and become immature chromaffin cells via a “bridge” pheochromocytoma. If one of our main goals is to cure neural crest- step before resuming proliferation and expanding the pool of chro- derived cancers and other diseases stemming from Schwann cells or maffin cells (Furlan et al., 2017). This proliferation halt is similar to their progenitors, a multidisciplinary approach must be involved. that described for sympathetic neurons (Gonsalvez et al., 2013). Expression of Mycn, the amplification of which correlates with 6. Concluding remarks progression and prognosis of neuroblastoma, was detected in both SCP and bridge cells (Furlan et al., 2017). Thus, the diversity and The progress made during recent years revealed that neural crest S32 A. Furlan, I. Adameyko Developmental Biology 444 (2018) S25–S35 cells eventually transit into a population of nerve-associated and Cell 104, 593–604. Coppola, E., Rallu, M., Richard, J., Dufour, S., Riethmacher, D., Guillemot, F., Goridis, multipotent neural crest-like cells, namely, SCPs that become gradually C., Brunet, J.F., 2010. Epibranchial ganglia orchestrate the development of the fate-restricted on the path towards Schwann cell differentiation. SCPs cranial neurogenic crest. Proc. Natl. Acad. Sci. USA 107, 2066–2071. strongly resemble the crest based on their gene expression programs Costa, F.A., Moreira Neto, F.L., 2015. [Satellite glial cells in sensory ganglia: its role in pain]. Rev. Bras. Anestesiol. 65, 73–81. and multipotency and challenge old views on the evolution of neural Coulpier, F., Decker, L., Funalot, B., Vallat, J.M., Garcia-Bragado, F., Charnay, P., crest properties, suggesting a novel scenario where proto-crest cells Topilko, P., 2010. CNS/PNS boundary transgression by central glia in the absence of could spread throughout the body via nerve-dependent migration. Schwann cells or Krox20/Egr2 function. J. Neurosci. 30, 5958–5967. Coulpier, F., Le Crom, S., Maro, G.S., Manent, J., Giovannini, M., Maciorowski, Z., Finally, the plasticity of Schwann cells and their progenitors plays a key Fischer, A., Gessler, M., Charnay, P., Topilko, P., 2009. Novel features of boundary role in the development of numerous human diseases ranging from cap cells revealed by the analysis of newly identified molecular markers. Glia 57, neurofibromatosis to leprosy. Hopefully, such plasticity can be har- 1450–1457. Davies, A.M., 1998. Neuronal survival: early dependence on Schwann cells. Curr. Biol. 8, nessed for future therapeutic implications that would include better R15–R18. nerve regeneration, improved skin healing, in vivo induction of Dooley, C.M., Mongera, A., Walderich, B., Nusslein-Volhard, C., 2013. On the embryonic neurons inside of the aganglionic human gut or the development of origin of adult melanophores: the role of ErbB and Kit signalling in establishing the new strategies for repigmentation of patients suffering from melanophore stem cells in zebrafish. Development 140, 1003–1013. Duband, J.L., 2006. Neural crest delamination and migration: integrating regulations of cosmetic defects, vitiligo and skin burns. cell interactions, locomotion, survival and fate. Adv. Exp. Med Biol. 589, 45–77. Dublin, P., Hanani, M., 2007. Satellite glial cells in sensory ganglia: their possible Acknowledgements contribution to inflammatory pain. Brain Behav. Immun. 21, 592–598. Dupin, E., Real, C., Glavieux-Pardanaud, C., Vaigot, P., Le Douarin, N.M., 2003. Reversal of developmental restrictions in neural crest lineages: transition from Schwann cells We thank our illustrator Dr. Olga Kharchenko for helping with to glial-melanocytic precursors in vitro. Proc. Natl. Acad. Sci. USA 100, 5229–5233. figures. IA was supported by the ERC Consolidator Grant Dupin, E., Sommer, L., 2012. Neural crest progenitors and stem cells: from early development to adulthood. Dev. Biol. 366, 83–95. “STEMMING-FROM-NERVE”, Vetenskapsradet Project Grant, Bertil Dyachuk, V., Furlan, A., Shahidi, M.K., Giovenco, M., Kaukua, N., Konstantinidou, C., Hallsten Research Foundation, Ake Wiberg Foundation and EMBO Pachnis, V., Memic, F., Marklund, U., Muller, T., Birchmeier, C., Fried, K., Ernfors, Young Investigator Program. P., Adameyko, I., 2014. Neurodevelopment. Parasympathetic neurons originate from nerve-associated peripheral glial progenitors. Science 345, 82–87. Er, U., Kazanci, A., Eyriparmak, T., Yigitkanli, K., Senveli, E., 2007. Melanotic References schwannoma. J. Clin. Neurosci. 14, 676–678. Erdmann, F., Lortet-Tieulent, J., Schuz, J., Zeeb, H., Greinert, R., Breitbart, E.W., Bray, F., 2013. International trends in the incidence of malignant melanoma 1953–2008– Abitua, P.B., Wagner, E., Navarrete, I.A., Levine, M., 2012. Identification of a are recent generations at higher or lower risk? Int J. Cancer 132, 385–400. rudimentary neural crest in a non-vertebrate chordate. Nature 492, 104–107. Espinosa-Medina, I., Outin, E., Picard, C.A., Chettouh, Z., Dymecki, S., Consalez, G.G., Adameyko, I., Lallemend, F., 2010. Glial versus melanocyte cell fate choice: schwann cell Coppola, E., Brunet, J.F., 2014. Neurodevelopment. Parasympathetic ganglia derive precursors as a cellular origin of melanocytes. Cell Mol. Life Sci. 67, 3037–3055. from Schwann cell precursors. Science 345, 87–90. Adameyko, I., Lallemend, F., Aquino, J.B., Pereira, J.A., Topilko, P., Muller, T., Fritz, N., Flood, P.R., 1966. A peculiar mode of muscular innervation in Amphioxus. Light and Beljajeva, A., Mochii, M., Liste, I., Usoskin, D., Suter, U., Birchmeier, C., Ernfors, P., electron microscopic studies of the so-called ventral roots. J. Comp. Neurol. 126, 2009. Schwann cell precursors from nerve innervation are a cellular origin of 181–217. melanocytes in skin. Cell 139, 366–379. Freter, S., Fleenor, S.J., Freter, R., Liu, K.J., Begbie, J., 2013. Cranial neural crest cells Adameyko, I., Lallemend, F., Furlan, A., Zinin, N., Aranda, S., Kitambi, S.S., Blanchart, form corridors prefiguring sensory neuroblast migration. Development 140, A., Favaro, R., Nicolis, S., Lubke, M., Muller, T., Birchmeier, C., Suter, U., Zaitoun, I., 3595–3600. Takahashi, Y., Ernfors, P., 2012. Sox2 and Mitf cross-regulatory interactions Furlan, A., Dyachuk, V., Kastriti, M.E., Calvo-Enrique, L., Abdo, H., Hadjab, S., consolidate progenitor and melanocyte lineages in the cranial neural crest. Chontorotzea, T., Akkuratova, N., Usoskin, D., Kamenev, D., Petersen, J., Sunadome, Development 139, 397–410. K., Memic, F., Marklund, U., Fried, K., Topilko, P., Lallemend, F., Kharchenko, P.V., Aquino, J.B., Hjerling-Leffler, J., Koltzenburg, M., Edlund, T., Villar, M.J., Ernfors, P., Ernfors, P., Adameyko, I., 2017. Multipotent peripheral glial cells generate 2006. In vitro and in vivo differentiation of boundary cap neural crest stem cells into neuroendocrine cells of the adrenal medulla. Science 357. mature Schwann cells. Exp. Neurol. 198, 438–449. Goldgeier, M.H., Klein, L.E., Klein-Angerer, S., Moellmann, G., Nordlund, J.J., 1984. The Aso, M., Hashimoto, K., Eto, H., Fukaya, T., Ishihara, M., Shimao, S., Shimizu, Y., 1988. distribution of melanocytes in the leptomeninges of the human brain. J. Invest Expression of Schwann cell characteristics in pigmented nevus. Dermatol. 82, 235–238. Immunohistochemical study using monoclonal antibody to Schwann cell associated Gonsalvez, D.G., Cane, K.N., Landman, K.A., Enomoto, H., Young, H.M., Anderson, C.R., antigen. Cancer 62, 938–943. 2013. Proliferation and cell cycle dynamics in the developing stellate ganglion. J. Baker, C.V., 2008. The evolution and elaboration of vertebrate neural crest cells. Curr. Neurosci. 33, 5969–5979. Opin. Genet Dev. 18, 536–543. Green, S.A., Bronner, M.E., 2014. The lamprey: a jawless vertebrate model system for Biswas, D., Marnane, C.N., Mal, R., Baldwin, D., 2007. Extracranial head and neck examining origin of the neural crest and other vertebrate traits. Differentiation 87, schwannomas–a 10-year review. Auris Nasus Larynx 34, 353–359. 44–51. Boerboom, A., Dion, V., Chariot, A., Franzen, R., 2017. Molecular mechanisms involved Green, S.A., Uy, B.R., Bronner, M.E., 2017. Ancient evolutionary origin of vertebrate in schwann cell plasticity. Front Mol. Neurosci. 10, 38. enteric neurons from trunk-derived neural crest. Nature 544, 88–91. Britsch, S., Goerich, D.E., Riethmacher, D., Peirano, R.I., Rossner, M., Nave, K.A., Gresset, A., Coulpier, F., Gerschenfeld, G., Jourdon, A., Matesic, G., Richard, L., Vallat, Birchmeier, C., Wegner, M., 2001. The transcription factor Sox10 is a key regulator J.M., Charnay, P., Topilko, P., 2015. Boundary Caps Give Rise to Neurogenic Stem of peripheral glial development. Genes Dev. 15, 66–78. Cells and Terminal Glia in the Skin. Stem Cell Rep. 5, 278–290. Bronner, M.E., 2012. Formation and migration of neural crest cells in the vertebrate Gupta, D., Thappa, D.M., 2013. Mongolian spots: how important are they? World J. Clin. embryo. Histochem Cell Biol. 138, 179–186. Cases 1, 230–232. Brownell, I., Guevara, E., Bai, C.B., Loomis, C.A., Joyner, A.L., 2011. Nerve-derived sonic Hall, B.K., Gillis, J.A., 2013. Incremental evolution of the neural crest, neural crest cells hedgehog defines a niche for hair follicle stem cells capable of becoming epidermal and neural crest-derived skeletal tissues. J. Anat. 222, 19–31. stem cells. Cell Stem Cell 8, 552–565. Han, S.B., Kim, H., Lee, H., Grove, M., Smith, G.M., Son, Y.J., 2017. Postinjury Induction Bullock, T.H., Moore, J.K., Fields, R.D., 1984. Evolution of myelin sheaths: both lamprey of Activated ErbB2 Selectively Hyperactivates Denervated Schwann Cells and and hagfish lack myelin. Neurosci. Lett. 48, 145–148. Promotes Robust Dorsal Root Axon Regeneration. J. Neurosci. 37, 10955–10970. Burnichon, N., Buffet, A., Gimenez-Roqueplo, A.P., 2016. Pheochromocytoma and Hanani, M., 2005. Satellite glial cells in sensory ganglia: from form to function. Brain Res paraganglioma: molecular testing and personalized medicine. Curr. Opin. Oncol. 28, Brain Res Rev. 48, 457–476. 5–10. Heermann, S., Spittau, B., Zajzon, K., Schwab, M.H., Krieglstein, K., 2012. Schwann cells Castro, A., Becerra, M., Manso, M.J., Sherwood, N.M., Anadon, R., 2006. Anatomy of the migrate along axons in the absence of GDNF signaling. BMC Neurosci. 13, 92. Hesse photoreceptor cell axonal system in the central nervous system of amphioxus. Holland, L.Z., Holland, N.D., 2001. Evolution of neural crest and placodes: amphioxus as J. Comp. Neurol. 494, 54–62. a model for the ancestral vertebrate? J. Anat. 199, 85–98. Chen, Z., Liu, C., Patel, A.J., Liao, C.P., Wang, Y., Le, L.Q., 2014. Cells of origin in the Huang, L.Y., Gu, Y., Chen, Y., 2013. Communication between neuronal somata and embryonic nerve roots for NF1-associated plexiform neurofibroma. Cancer Cell 26, satellite glial cells in sensory ganglia. Glia 61, 1571–1581. 695–706. Huber, K., Kalcheim, C., Unsicker, K., 2009. The development of the chromaffin cell Chicard, M., Colmet Daage, L., Clement, N., Danzon, A., Bohec, M., Bernard, V., lineage from the neural crest. Auton. Neurosci. 151, 10–16. Baulande, S., Bellini, A., Deveau, P., Pierron, G., Lapouble, E., Janoueix-Lerosey, I., Hussein, M.R., 2008. Extracutaneous malignant melanomas. Cancer Invest 26, 516–534. Peuchmaur, M., Corradini, N., Desfachelles, A.S., Valteau-Couanet, D., Michon, J., Isern, J., Garcia-Garcia, A., Martin, A.M., Arranz, L., Martin-Perez, D., Torroja, C., Combaret, V., Delattre, O., Schleiermacher, G., 2017. Whole exome sequencing of Sanchez-Cabo, F., Mendez-Ferrer, S., 2014. The neural crest is a source of cell-free DNA reveals temporo-spatial heterogeneity and identifies treatment- mesenchymal stem cells with specialized hematopoietic stem cell niche function. resistant clones in neuroblastoma. Clin. Cancer Res.. Elife 3, e03696. Cichowski, K., Jacks, T., 2001. NF1 tumor suppressor gene function: narrowing the GAP. S33 A. Furlan, I. Adameyko Developmental Biology 444 (2018) S25–S35 Isoldi, M.C., Rollag, M.D., Castrucci, A.M., Provencio, I., 2005. Rhabdomeric the skin. Cell 109, 693–705. phototransduction initiated by the vertebrate photopigment melanopsin. Proc. Natl. Mundell, N.A., Labosky, P.A., 2011. Neural crest stem cell multipotency requires Foxd3 Acad. Sci. USA 102, 1217–1221. to maintain neural potential and repress mesenchymal fates. Development 138, Ivashkin, E., Adameyko, I., 2013. Progenitors of the protochordate ocellus as an 641–652. evolutionary origin of the neural crest. Evodevo 4, 12. Muratori, L., Ronchi, G., Raimondo, S., Geuna, S., Giacobini-Robecchi, M.G., Fornaro, Ivashkin, E., Voronezhskaya, E.E., Adameyko, I., 2014. A paradigm shift in neurobiology: M., 2015. Generation of new neurons in dorsal root Ganglia in adult rats after peripheral nerves deliver cellular material and control development. Zool. (Jena.) peripheral nerve crush injury. Neural Plast. 2015, 860546. 117, 293–294. Nitzan, E., Pfaltzgraff, E.R., Labosky, P.A., Kalcheim, C., 2013. Neural crest and Schwann Iwamoto, S., Burrows, R.C., Agoff, S.N., Piepkorn, M., Bothwell, M., Schmidt, R., 2001. cell progenitor-derived melanocytes are two spatially segregated populations The p75 neurotrophin receptor, relative to other Schwann cell and melanoma similarly regulated by Foxd3. Proc. Natl. Acad. Sci. USA 110, 12709–12714. markers, is abundantly Expressed in spindled melanomas. Am. J. Dermatopathol. Pannese, E., 2010. The structure of the perineuronal sheath of satellite glial cells (SGCs) 23, 288–294. in sensory ganglia. Neuron Glia Biol. 6, 3–10. Jessen, K.R., Mirsky, R., 2016. The repair Schwann cell and its function in regenerating Parfejevs, V., Debbache, J., Shakhova, O., Schaefer, S.M., Glausch, M., Wegner, M., Suter, nerves. J. Physiol. 594, 3521–3531. U., Riekstina, U., Werner, S., Sommer, L., 2018. Injury-activated glial cells promote Johnston, A.P., Naska, S., Jones, K., Jinno, H., Kaplan, D.R., Miller, F.D., 2013. Sox2- wound healing of the adult skin in mice. Nat. Commun. 9, 236. mediated regulation of adult neural crest precursors and skin repair. Stem Cell Rep. Parichy, D.M., Spiewak, J.E., 2015. Origins of adult pigmentation: diversity in pigment 1, 38–45. stem cell lineages and implications for pattern evolution. Pigment Cell Melanoma Joseph, N.M., Mukouyama, Y.S., Mosher, J.T., Jaegle, M., Crone, S.A., Dormand, E.L., Res 28, 31–50. Lee, K.F., Meijer, D., Anderson, D.J., Morrison, S.J., 2004. Neural crest stem cells Parrinello, S., Napoli, I., Ribeiro, S., Wingfield Digby, P., Fedorova, M., Parkinson, D.B., undergo multilineage differentiation in developing peripheral nerves to generate Doddrell, R.D., Nakayama, M., Adams, R.H., Lloyd, A.C., 2010. EphB signaling endoneurial fibroblasts in addition to Schwann cells. Development 131, 5599–5612. directs peripheral nerve regeneration through Sox2-dependent Schwann cell sorting. Kalcheim, C., Burstyn-Cohen, T., 2005. Early stages of neural crest ontogeny: formation Cell 143 (1), 145–155. http://dx.doi.org/10.1016/j.cell.2010.08.039, Oct 1 https:// and regulation of cell delamination. Int J. Dev. Biol. 49, 105–116. www.ncbi.nlm.nih.gov/pubmed/15044753. Kastriti, M.E., Adameyko, I., 2017. Specification, plasticity and evolutionary origin of Peters, A., 1960. The structure of the peripheral nerves of the lamprey (Lampetra peripheral glial cells. Curr. Opin. Neurobiol. 47, 196–202. fluviatilis). J. Ultrastruct. Res 4, 349–359. Kaucka, M., Adameyko, I., 2014. Non-canonical functions of the peripheral nerve. Exp. Peters, A., 1963. The Structure of the Dorsal Root Nerves of Amphioxus; an Electron Cell Res 321, 17–24. Microscope Study. J. Comp. Neurol. 121, 287–304. Kaukua, N., Shahidi, M.K., Konstantinidou, C., Dyachuk, V., Kaucka, M., Furlan, A., An, Provencio, I., Jiang, G., De Grip, W.J., Hayes, W.P., Rollag, M.D., 1998. Melanopsin: an Z., Wang, L., Hultman, I., Ahrlund-Richter, L., Blom, H., Brismar, H., Lopes, N.A., opsin in melanophores, brain, and eye. Proc. Natl. Acad. Sci. USA 95, 340–345. Pachnis, V., Suter, U., Clevers, H., Thesleff, I., Sharpe, P., Ernfors, P., Fried, K., Radhadevi, C.V., Charles, K.S., Lathika, V.K., 2013. Orbital malignant melanoma Adameyko, I., 2014. Glial origin of mesenchymal stem cells in a tooth model system. associated with nevus of Ota. Indian J. Ophthalmol. 61, 306–309. Nature 513, 551–554. Radomska, K.J., Topilko, P., 2017. Boundary cap cells in development and disease. Curr. Kawakami, A., Fisher, D.E., 2011. Key discoveries in melanocyte development. J. Invest Opin. Neurobiol. 47, 209–215. Dermatol. 131, E2–E4. Real, C., Glavieux-Pardanaud, C., Vaigot, P., Le-Douarin, N., Dupin, E., 2005. The Kelsh, R.N., Barsh, G.S., 2011. A nervous origin for fish stripes. PLoS Genet 7, e1002081. instability of the neural crest phenotypes: schwann cells can differentiate into Kim, H.S., Lee, J., Lee, D.Y., Kim, Y.D., Kim, J.Y., Lim, H.J., Lim, S., Cho, Y.S., 2017. myofibroblasts. Int J. Dev. Biol. 49, 151–159. Schwann Cell Precursors from Human Pluripotent Stem Cells as a Potential Rizvi, T.A., Huang, Y., Sidani, A., Atit, R., Largaespada, D.A., Boissy, R.E., Ratner, N., Therapeutic Target for Myelin Repair. Stem Cell Rep. 8, 1714–1726. 2002. A novel cytokine pathway suppresses glial cell melanogenesis after injury to Kucenas, S., Takada, N., Park, H.C., Woodruff, E., Broadie, K., Appel, B., 2008. CNS- adult nerve. J. Neurosci. 22, 9831–9840. derived glia ensheath peripheral nerves and mediate motor root development. Nat. Saito, D., Takase, Y., Murai, H., Takahashi, Y., 2012. The dorsal aorta initiates a Neurosci. 11, 143–151. molecular cascade that instructs sympatho-adrenal specification. Science 336, Kumar, A., Godwin, J.W., Gates, P.B., Garza-Garcia, A.A., Brockes, J.P., 2007. Molecular 1578–1581. basis for the nerve dependence of limb regeneration in an adult vertebrate. Science Singh, A.P., Dinwiddie, A., Mahalwar, P., Schach, U., Linker, C., Irion, U., Nusslein- 318, 772–777. Volhard, C., 2016. Pigment Cell Progenitors in Zebrafish Remain Multipotent Kuo, B.R., Erickson, C.A., 2010. Regional differences in neural crest morphogenesis. Cell through Metamorphosis. Dev. Cell 38, 316–330. Adh Migr. 4, 567–585. Sommer, L., 2011. Generation of melanocytes from neural crest cells. Pigment Cell Lanser, M.J., Sussman, S.A., Frazer, K., 1992. Epidemiology, pathogenesis, and genetics Melanoma Res 24, 411–421. of acoustic tumors. Otolaryngol. Clin. North Am. 25, 499–520. Steel, K.P., Barkway, C., 1989. Another role for melanocytes: their importance for normal Laranjeira, C., Sandgren, K., Kessaris, N., Richardson, W., Potocnik, A., Vanden Berghe, stria vascularis development in the mammalian inner ear. Development 107, P., Pachnis, V., 2011. Glial cells in the mouse enteric nervous system can undergo 453–463. neurogenesis in response to injury. J. Clin. Invest 121, 3412–3424. Stolfi, A., Ryan, K., Meinertzhagen, I.A., Christiaen, L., 2015. Migratory neuronal Levin, M.D., Lu, M.M., Petrenko, N.B., Hawkins, B.J., Gupta, T.H., Lang, D., Buckley, progenitors arise from the neural plate borders in tunicates. Nature 527, 371–374. P.T., Jochems, J., Liu, F., Spurney, C.F., Yuan, L.J., Jacobson, J.T., Brown, C.B., Thomas, A.J., Erickson, C.A., 2009. FOXD3 regulates the lineage switch between neural Huang, L., Beermann, F., Margulies, K.B., Madesh, M., Eberwine, J.H., Epstein, J.A., crest-derived glial cells and pigment cells by repressing MITF through a non- Patel, V.V., 2009. Melanocyte-like cells in the heart and pulmonary veins contribute canonical mechanism. Development 136, 1849–1858. to atrial arrhythmia triggers. J. Clin. Invest 119, 3420–3436. Turchini, J., Cheung, V.K.Y., Tischler, A.S., De Krijger, R.R., Gill, A.J., 2018. Pathology Li, H., Zhao, X., Yan, X., Jessen, W.J., Kim, M.O., Dombi, E., Liu, P.P., Huang, G., Wu, J., and genetics of phaeochromocytoma and paraganglioma. Histopathology 72, 2016. Runx1 contributes to neurofibromatosis type 1 neurofibroma formation. 97–105. Oncogene 35, 1468–1474. Uesaka, T., Nagashimada, M., Enomoto, H., 2015. Neuronal Differentiation in schwann Li, W., Kohara, H., Uchida, Y., James, J.M., Soneji, K., Cronshaw, D.G., Zou, Y.R., cell lineage underlies postnatal neurogenesis in the enteric nervous system. J. Nagasawa, T., Mukouyama, Y.S., 2013. Peripheral nerve-derived CXCL12 and VEGF- Neurosci. 35, 9879–9888. A regulate the patterning of arterial vessel branching in developing limb skin. Dev. Vermeren, M., Maro, G.S., Bron, R., McGonnell, I.M., Charnay, P., Topilko, P., Cohen, J., Cell 24, 359–371. 2003. Integrity of developing spinal motor columns is regulated by neural crest Ma, M.S., Boddeke, E., Copray, S., 2015. Pluripotent stem cells for Schwann cell derivatives at motor exit points. Neuron 37, 403–415. engineering. Stem Cell Rev. 11, 205–218. Vesalius, A., 2003. On the Fabric of the Human Body: A Translation of De Humani Maris, J.M., Hogarty, M.D., Bagatell, R., Cohn, S.L., 2007. Neuroblastoma. Lancet 369, Corporis Fabrica Libri Septem. Vol. III, Book III: The Veins and Arteries; Book IV: 2106–2120. the Nerves. (transl. W F Richardson in collaboration with J B Carman) Vesalius A Marmigère, F., Ernfors, P., 2007. Specification and connectivity of neuronal subtypes in Novato. Norman Publishing. the sensory lineage. Nat. Rev. Neurosci. 8 (2), 114–127. Wahlbuhl, M., Reiprich, S., Vogl, M.R., Bosl, M.R., Wegner, M., 2012. Transcription Maro, G.S., Vermeren, M., Voiculescu, O., Melton, L., Cohen, J., Charnay, P., Topilko, P., factor Sox10 orchestrates activity of a neural crest-specific enhancer in the vicinity of 2004. Neural crest boundary cap cells constitute a source of neuronal and glial cells its gene. Nucleic Acids Res 40, 88–101. of the PNS. Nat. Neurosci. 7, 930–938. Warner, T.F., Hafez, G.R., Finch, R.E., Brandenberg, J.H., 1981. Schwann cell features in Martinez-Morales, J.R., Rodrigo, I., Bovolenta, P., 2004. Eye development: a view from neurotropic melanoma. J. Cutan. Pathol. 8, 177–187. the retina pigmented epithelium. Bioessays 26, 766–777. Wicks, N.L., Chan, J.W., Najera, J.A., Ciriello, J.M., Oancea, E., 2011. UVA Masaki, T., Qu, J., Cholewa-Waclaw, J., Burr, K., Raaum, R., Rambukkana, A., 2013. phototransduction drives early melanin synthesis in human melanocytes. Curr. Biol. Reprogramming adult Schwann cells to stem cell-like cells by leprosy bacilli 21, 1906–1911. promotes dissemination of infection. Cell 152, 51–67. Woodhoo, A., et al., 2009. Notch controls embryonic Schwann cell differentiation, Mauti, O., Domanitskaya, E., Andermatt, I., Sadhu, R., Stoeckli, E.T., 2007. p