Neural Crest Cells PDF
Document Details
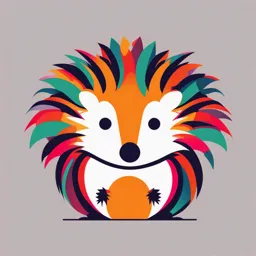
Uploaded by ReasonableConsonance
Tags
Summary
This document provides an overview of neural crest cells, including their functional domains, migration pathways, and differentiation. It details the initiation, substrate recognition, and final differentiation, as well as the different cell types the neural crest cells can develop into, such as sympathetic neurons, and parasympathetic neurons. It also explores models for neural crest cell differentiation and clonal analysis.
Full Transcript
The Neural Crest functional domains the trunk neural crest pathways of migration initiation of migration pathway restriction differentiation final differentiation Cranial Ectodermal Placodes - the otic vesicle Chapt 17: p. 525-530; 536-539 Chapt 18: p. 574-578 (Chapt 15: p. 44...
The Neural Crest functional domains the trunk neural crest pathways of migration initiation of migration pathway restriction differentiation final differentiation Cranial Ectodermal Placodes - the otic vesicle Chapt 17: p. 525-530; 536-539 Chapt 18: p. 574-578 (Chapt 15: p. 441-445; 452-454 Chapt 16: p. 485-487) The neural crest The neural crest is derived from cells between the epidermal ectoderm and the neural ectoderm. These cells dissociate and migrate to produce many different tissue types. Four functional domains (4 overlapping anatomical regions): 1) Cranial: face cartilage, bone, cranial nerves & ganglia, connective tissue of pharyngeal arches 2) Cardiac (near somites 1-3): heart arteries and connective tissue of aortic arches, melanocytes 3) Trunk (near somite 6 to the tail end): melanocytes, dorsal root ganglia, adrenal gland, nerves surrounding aorta 4) Vagal (neck, near somites 1-7), and Sacral (pelvic, posterior to somite 28): gut enteric ganglia Functional domains of the neural crest The trunk neural crest: pathways of migration Pathway 1: ventral (through the sclerotomes). → all trunk neural crest derivatives, except melanocytes Pathway 2: dorsolateral (between the epidermis and somites). → melanocytes Trunk neural crest (2) (1) Pathway 1: a closer look Pathway 1 migration route: Dorsoventrally around the neural tube. - Some cells: migrate through and remain associated with the sclerotome > become dorsal root ganglia (sensory neurons) Note: the sclerotome is made of loosely connected cells, while the dermamyotome is more solid. - Some cells: migrate more ventrally between the sclerotome and the dermamyotome > become sympathetic ganglia or adrenal medulla. Pathways of migration Segmental restriction Segmental restriction Pathway 1 cells migrate only through the anterior portion of the sclerotome. Figure: fluorescence microscopy of migrating trunk neural crest cells detected with a fluorescent antibody against the neural crest-specific HNK-1 antigen A: Frontal section of a 2-day chick embryo. → migrating cells localize to anterior sclerotome B: Cross section through the anterior sclerotome. → migrating HNK-1 expressing cells C: Cross section through the posterior sclerotome. → no migrating cells Ventral pathway A Ventral pathway B C The migration process The four steps in the migration process: 1) Initiation of migration 2) Substrate recognition by migrating neural crest cells 3) Differentiation 4) Final differentiation The migration process 1) Initiation of migration: The surrounding neural plate and epidermal tissues induce the changes in the neural crest cells that are necessary for their migration Where Wnt signals induce BMP expression will induce: FoxD3 - necessary for the specification of neural crest cells Slug - activates factors that repress Cadherin expression = breaking tight junctions, causing the neural crest cells to dissociate later, RhoA induces changes in the neural crest cell cytoskeleton (= migration by actin polymerization) Initiation of migration + Wnt BMP RhoA The migration process 2) Substrate recognition by migrating neural crest cells: cells recognize the extracellular matrices of other cells: fibronectins, laminins, collagens and proteoglycans (all promote migration) the repulsive signal Ephrin is responsible for the segmental Restriction The pathway of neural crest cell migration and the range of Ephrin expression do not overlap Ephrin is expressed only by cells in the posterior sclerotome neural crest cells express the Ephrin receptor Segmental restriction A P The migration process 3) Differentiation: pre-migratory neural crest cells are capable of differentiating into several cell types (they are multipotent) Examples: Vagal neural crest → parasympathetic (cholinergic) neurons (secrete and respond to acetylcholine) Trunk neural crest → sympathetic (adrenergic) neurons (secrete and respond to norepinephrine) Multipotency of pre-migratory cells After reciprocal transplantation of pre-migratory cells: Vagal neural crest cells > produce adrenergic neurons in sympathetic ganglia Trunk neural crest cells > produce cholinergic neurons of parasympathetic division So: one population of neural crest cells can generate different neuronal types: pre-migratory cells express enzymes for the synthesis of both neurotransmitters (acetylcholine and norepinephrine) differentiation depends on signals encountered by the cells during their migration Multipotency of a pre-migratory neural crest cell Signal: Models for neural crest cell differentiation A. Multipotency hypothesis all neural crest cells have the potential to form any neural crest derivative their determination depends on the environmental signals received (pre-migratory cells are undetermined, and their migration pathway determines their fate) B. Selection hypothesis neural crest cells are determined before migration begins any population of pre-migratory neural crest cells is a mixture of already determined cells the environment of the migration pathway enhances the pro- liferation and survival of appropriately determined cells Models for neural crest cell differentiation Models for neural crest cell differentiation Clonal analysis of neural crest cells Clonal analysis: a single cell is cultured in a medium that supports its survival and proliferation without providing any signals that are known to restrict cell potency in any particular way. - When the cell has proliferated to form a colony of clones, the descendants are analyzed to see what types of cells they have differentiated into: cell morphology immunostaining for characteristic proteins Clonal analysis of trunk neural crest cells Cohen and Konigsberg (1975) established such cultures from trunk neural crest cells from quail: a segment of neural tube with adhered trunk neural crest cells was kept in tissue culture until the neural crest cells had migrated away from the neural tube the neural tube was then removed the neural crest cells were re-plated at low density so that individual cells could form new colonies Three types of clones were observed: all pigmented cells (melanocytes) all unpigmented cells (sympathetic neurons of the adrenal medulla) some pigmented and some unpigmented cells within the same clone Determination of pre-migratory neural crest cells by region-specific ECM components Experiment: 1. Sterile nitrocellulose microcarriers (400 X 150 X 4 um) are implanted into the trunk region of axolotl embryos along the two main migration routes of neural crest cells. 2. The carriers are left in the embryos to adsorb extracellular matrix (ECM) components for 10-12 hrs, and removed just before the onset of neural crest cell migration. 3. Pre-migratory neural crest cells removed from early embryos are cultured on ECM-covered microcarriers for 5 days. Determination of pre-migratory of neural crest cells by region-specific ECM components Results: neural crest cells cultured with ECM from the subepidermal space (the dorsolateral pathway) developed into xanthophores or melanocytes neural crest cells cultured with ECMs from the dorsal root ganglion area (the ventral pathway) developed into neurons neural crest cells cultured without ECM remained undifferentiated Current view Before migration the neural crest is a mixed population of - multipotent cells, and - cells with a more restricted potential Final determination probably involves both mechanisms: pre-specification of cell fate & selection for cell survival Current model: Multipotent cell Restricted cell (bipotent) signal 1 or signal 2 cell type 1 cell type 2 = differentiated cell types The migration process 4. Final differentiation: a direct effect of the environment. Example: effect of signals (environment) on adrenal medulla precursors. The adrenal medulla is the portion of the adrenal gland that secretes catecholamines (epinephrine and norepinephrine, which regulate smooth muscle contractions and cardiac activity, increase glycolysis and lipolysis, and decrease glycogenesis in preparation for muscular activity, as part of the sympathetic division“fight-or-flight reaction”). the tissue contains both adrenergic neurons and chromaffin cells (catecholamine-secreting cells) Bipotent cell FGF2, NGF Adrenergic disable glucocorticoid response neuron pathway to neural fate (neurotransmitter secreting) Adrenomedullary Glucocorticoids (chromaffin) cell inhibit factors that induce (hormone secreting) neural differentiation induce expression of adrenomedullary-specific enzymes Differentiation of the adrenal medulla precursors FGF: fibroblast growth factor NGF: nerve growth factor Cranial ectodermal placodes Cranial placodes are local and transient thickenings of the epidermal ectoderm in the head and the neck. derived from competent tissue that surrounds the anterior neural plate cells within these placodes have neurogenic potential: they are competent for induction by neighboring tissues sensory placodes form the distal neurons of ganglia associated with hearing, balance, taste and smell neural crest cells contribute the glial cells of these sensory ganglia Cranial ectodermal placodes Induction of the chick otic placode The otic placode develops into the sensory cells of the inner ear. the cranial paraxial mesoderm underlying the competent ectodermal tissue secretes Fgf19, which is received by both presumptive otic vesicle and adjacent neural plate in response, this adjacent neural plate secretes Wnt8c and Fgf3, which act synergistically with Fgf19 to induce the formation of the otic placode the location of Fgf19 secretion is controlled by Fgf8 from the endodermal region beneath the cranial paraxial mesoderm Induction of the chick otic placode (cranial paraxial) Formation of the otic vesicle from the otic placode in the human embryo Side view of a 28-day-old human embryo. The otic vesicle becomes the inner ear.