Module 1: Sample Preparation and Presumptive Tests PDF
Document Details
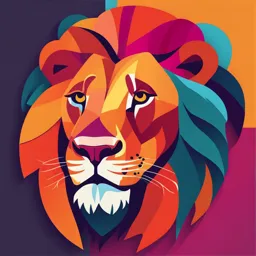
Uploaded by MizzScholar24
Tags
Summary
This module introduces the procedures for the initial examination of drug-related evidence, including sample acquisition, note-taking, preparation, and preliminary/confirmatory testing. It covers sample storage, drug stability, and potential consequences of degradation. Focuses on the chemistry behind preliminary tests like spot tests, and the importance of controls and validation.
Full Transcript
Module 1: Sample Preparation and Presumptive Tests Page 1 Module Overview Introduction Welcome to Module 1. In this module we will introduce you to the steps that are required in the initial examination of toxicological samples and drug-related evidence to include sample acquisition, note taking, sa...
Module 1: Sample Preparation and Presumptive Tests Page 1 Module Overview Introduction Welcome to Module 1. In this module we will introduce you to the steps that are required in the initial examination of toxicological samples and drug-related evidence to include sample acquisition, note taking, sample preparation and preliminary and confirmatory testing. We will also discuss sample storage requirements and drug stability factors that can play a critical role in the outcome of the forensic testing processes that will be covered during this course. Our primary objective in this module is to help you understand the procedures involved in the initial examination of drug related evidence, and the screening of these samples for subsequent instrumental analyses. The ultimate goal of drug analyses is to unequivocally identify the drug or drugs present in a sample by determining the chemical structure of the substances present and to determine, where possible, the amount of those substances present. Such qualitative and quantitative confirmatory tests are conducted after the samples are initially screened using preliminary drug tests. Preliminary drug tests allow drugs to be classified into a particular chemical group, but do not unequivocally identify the presence of a specific chemical compound. Preliminary test results can then be used as a guide to the appropriate confirmatory tests or methods that need to be applied to determine and verify the structure of the chemical compounds present in a drug related sample. Objectives At the end of this module students should: Be able to describe and record evidence received Be able to determine appropriate storage conditions until analysis Understand the need for appropriate controls and the nature of positive and negative control samples Understand the significance of false positive and false negative tests Understand the significance sample/drug stability plays in relationship to forensic testing Understand the difference between preliminary and confirmatory drug tests Understand the chemistry of preliminary drug tests such as spot tests, microcrystal tests, and the microscopic analyses of cannabis Module 1: Sample Preparation and Presumptive Tests Page 2 Sample Acquisition, Storage and Quality Control Sample Acquisition, Storage and Stability Acquisition Forensic samples may originate from law enforcement and/or medical examiner investigations, workplace drug testing or through the monitoring of athletes for potential doping. Appropriate handling and storage of these samples, from collection to final disposition of the samples after testing (based on applicable retention laws) is critical to ensure their validity and their admissibility in any potential court proceedings. When samples are submitted to a forensic laboratory for testing, the first steps taken normally involve assessing all associated specimen documents, including collection and chain of custody forms as well as conducting a thorough review of all packaging and integrity seals. Additionally, upon submission laboratory personnel may utilize an electronic laboratory information management system (LIMS) to take comprehensive notes that document all pertinent sample related information. The laboratory is responsible for ensuring secure storage of any submitted samples. The laboratory should also take reasonable steps to improve the stability of any compounds that may be present in the specimens by storing and processing them under suitable environmental conditions. Description When seized drug or biological samples are acquired for testing the appearance and features of the substance must also be recorded. These features may include: For seized drug samples: Weight/Volume/Amount Number of packages Nature of the substance (e.g. plant material, powder, tablet, liquid) Color Odor (if obvious) Condition (e.g. wet or dry) For toxicology samples: Sample type (e.g. blood, urine, vitreous humor, hair, etc.) Anticoagulants/Preservatives added for sample stability Volume Type of container used for collection Condition (e.g. blood color, if blood contains clots) Any measurements made at this stage of the examination (physical dimensions, weights, temperature) should be made with due regard for the accuracy and precision of the measuring device. For example there is no point in reporting the weight of a sample to four decimal places if the balance is only accurate to two decimal places. Such practices can also lead to difficult questions under cross-examination concerning the accuracy and precision of instruments used in the analysis. Storage Exposure to environmental factors such as air, temperature, humidity and light must all be considered when storing drug samples. Biological specimens are generally refrigerated when stored for short periods of time prior to testing. For longer-term storage, they may be frozen. Other samples, such as hair, nails and dried blood collected on filter paper are less temperature sensitive and can be stored at room temperature. These samples should also be collected and stored in appropriate containers to prevent potential contamination and degradation from occurring. Practicality plays a role in the type of collection containers normally utilized, so the forensic toxicologist must be aware of possible interferences that might occur between a drug and container during storage and testing. Seized drug evidence should also be stored in a manner that will preserve the sample’s integrity. Fresh plant material, for example, should be stored in paper versus plastic bags to prevent mold growth. Powder drug samples, tablets, capsules, etc., should be kept dry, stored at stable temperatures, and protected from light. Liquids should be stored at an appropriate temperature (refrigerated or frozen), protected from light and air as necessary. Storage temperatures should be monitored and, preferably, recorded at least once a day in addition to measures taken to minimize contact with air, humidity and light. Efforts should always be made to prevent potential contaminants from coming into contact with the sample and to improve the sample’s stability. Both of which may impact the testing results obtained. The goal of storage preservation methods is, as much as possible, to yield test results reflective of what was contained in the specimen at the time of collection. Contamination One of the biggest concerns of any forensic laboratory and the point of attack for many defense attorneys is the possibility of sample contamination. A major crime laboratory handles many thousands of drug cases each year, often in a limited amount of laboratory space. Every effort must be made to avoid the possibility of contamination between samples and/or between the sample and a drug standard. The seriousness of possible contamination resulting in a false positive and the subsequent accusation of an innocent person cannot be emphasized enough. Such circumstances, although isolated, have resulted in the demise of several major laboratories and dozens of capable analysts during recent years. When conducting preliminary tests, it is important to consider the pitfalls associated with these, usually very sensitive tests. A false positive is identifying a sample as being positive for a particular drug when in fact none is present. This could either be associated with contamination of the case sample with the drug in question, or failure of the analytical test to produce an accurate result. A false negative is often considered to be a less serious error, and can be defined as failing to identify the presence of a drug when it is known to be present in the sample. False negatives are associated with the failure of the analytical test and can result in critical data being lost. False negatives and false positives can be addressed by the inclusion of positive and negative controls in the testing regimen and an understanding of the limitations of the testing regimen through appropriate method validation. A positive control is a substance that is known to react in the same way as a positive sample. For example, if testing a urine sample for the presence of amphetamine, drug free urine spiked with a standard solution of amphetamine will suffice as a positive control. In this example, a negative control could consist of a drug free urine sample. Appropriate validation studies may include an evaluation of the sensitivity and specificity of the method. Stability Many drugs are not chemically stable and can be subject to chemical, physical or microbial degradation, regardless of whether or not the drug is in a solid dosage form or biological matrix. Factors playing a role in catalyzing these reactions can include temperature, light, air, humidity, and pH of the solution the drug may be contained in. Sample acquisition timing (especially in postmortem cases), collection and storage procedures as well as procedural aspects, including type and duration, of analytical testing processes, must be taken into account when interpreting test results. All have the potential for producing an environment conducive to these types of drug degradation. Chemical degradation can result in loss of pure drug by breakdown to another compound (such as cocaine to the metabolite benzoylecgonine). Physical decomposition can result in: Changes in the crystal form of a drug Aggregation of drug particles in a suspension Loss of volatile chemical compounds to the atmosphere and container (alcohol) Microbial contamination can occur in any sample containing water. Mechanisms of Drug Degradation Most of the chemical instability of drugs can be attributed to hydrolysis, oxidation or photochemical changes due to the ubiquitous nature of water, oxygen, and light in our environment. Hydrolysis Drugs that possess an ester or an amide bond are particularly susceptible to hydrolysis Ester hydrolysis is dependent on the pH of the solution and is catalyzed by acids or bases Examples of drugs susceptible to hydrolysis include heroin, cocaine, and methylphenidate Oxidation Drugs that possess an amine, alcohol, aldehyde functional groups, for example Examples of drugs susceptible to oxidation include ethanol Photolysis Photochemical reactions occur when a molecule absorbs a photon of light energy to produce an unstable excited state molecule. This extra energy can be lost by several different mechanisms: Fluorescence Physical decay (loss of energy in the form of heat) Chemical decay (breakdown of the drug via oxidation, reduction, hydrolysis, fragmentation, rearrangement) Examples of susceptible drugs include hydrocortisone, ketoprofen, sodium nitroprusside, and chlorpromazine Ultraviolet light is the most energetic light in the spectrum and can induce chemical decay. This process can occur when the drug is in both aqueous and organic solvents. Temperature Temperature can accelerate these degradation reactions. A reaction rate’s temperature dependence is revealed through Arrhenius’ equation: k= reaction rate A= frequency of molecular collisions factor EA= activation energy R= gas constant T= temperature The rate of a chemical reaction is related to the absolute temperature in Kelvin (Arrhenius). Reaction rates increase by a factor of 2-3 for every 10°C rise in temperature. The Arrhenius equation is applicable to solution reactions providing that the temperature range is between 30-50°C. Module 1: Sample Preparation and Consequences of Drug Degradation Presumptive Tests Page 3 Consequences of Drug Degradation Solid State Degradation Degradation of drugs in the solid state (tablets) can result in physical or chemical changes. Solidstate decomposition can be divided into two types: Degradation at the tablet surface True solid degradation The most common situation involves reactions in adsorbed surface moisture resulting in saturated or concentrated drug solutions. The surface solution phase may result from: melting of the drug or other component and /or adsorbed moisture from the atmosphere. Physical Decomposition Involves no change in the covalent bonds of the drug but may involve changes in the crystal lattice structure. A crystalline drug has a repeating arrangement of individual molecules (crystal lattice) and a characteristically narrow melting point range. An amorphous form of a drug has no specific arrangement and melts over a range of 10 or more degrees. Amorphous forms will melt at lower temperatures than pure crystalline substances. A drug is polymorphic if the pure drug can exist in more than one different crystalline form. Polymorphic forms of a drug have different solubilities. The rate of transformation of one form to another is extremely variable and is often accelerated by the presence of water and/or heat (known to occur with some steroids and antibiotics). Physicochemical factors that affect drug decomposition rates include, temperature, catalysts, pH and nature of solvent(s) present. Excipients, Diluents and Cutting Agents Other compounds present in the drug sample can affect the stability of the drug as a result of catalytic effects, changing surface pH, or direct reaction with the drug. In pharmaceutical preparations, these compounds are added to provide bulk, but may also aid in protection against hydrolysis, oxidation and photolysis. Examples include antioxidants such as citric acid and buffering agents and preservatives, which can aid in microbial prevention. Starches added may contain a lot of moisture, which could also lead to degradation. Aspirin, a good acetylating agent, reacts with other drugs containing hydroxyl or amine groups (codeine, acetaminophen, phenylephrine, and caffeine). Preventative Measures and Considerations As has been discussed, drug degradation can take place by a variety of different reaction mechanisms When a sample is received by the laboratory for testing, there should be a thorough inspection of the evidence, recording anything that could contribute to breakdown of the sample such as the presence of moisture or possible exposure to heat and air. Temperature, pH, humidity, lighting, containers, and other materials utilized may need to be considered during the collection, preparation and processing of forensic samples. During collection additives and preservatives can be added to biological samples to preserve the sample’s integrity and prevent microbial growth. In blood samples preservatives are added to prevent any possibility of ethanol loss from microbial action, for example. In urine samples, preservatives may prevent an increase in ethanol concentration when both glucose and microbes such as yeast (Candida albicans) are present. Postmortem samples may be exposed to microbial contamination prior to collection so the type and conditions of samples collected should be considered. A good example of where that has to be considered is also with ethanol. Central blood samples (e.g. heart or chest cavity) are not generally preferred for this purpose, as the possibility of microbial contamination, even shortly after death, is high primarily due to its close proximity to the microbes in the gut; thus, peripheral blood and samples such as urine and vitreous humor should also be collected. Headspace above the sample in the collection container may need to be minimized. A large headspace can result in potential drug losses through oxidative processes during long-term storage, as has been observed for ethanol in blood. Diffusion of a volatile drug from the sample to the headspace can also occur, especially for extremely volatile compounds. For example, halogenated hydrocarbons used as propellants or in HVAC and refrigeration systems may be abused as inhalants. If inhalants are suspected as a cause of death, additional specimens such as lung along with other more routine specimens may be appropriate to collect with a portion stored in airtight headspace vials. Sample container composition must also be considered as this can also play a role in drug loss and potential interference. Some drugs (e.g. THC) can adsorb onto certain types of glass or plastic containers. Plasticizers, such as phthalates, can leach into the sample and interfere with analytical detection. Teflon versus rubber stoppers/caps may also be used, as some rubber components (e.g. benzothiazole) may also leach into the samples, especially over long-term storage. During analytical processing, these compounds may interfere with sensitive drug detection so it may be preferable to use one type of container and/or lid over another for these reasons. To prevent photolysis, laboratories can use amber vials and glassware and/or aluminum foil wrapping in addition to dark storage areas. A drug’s stability can also be affected during testing processes and should be taken into account when designing and validating methods and when interpreting analytical test results. During preparation of biological samples drug degradation or transformation may occur. In particular, during the extraction procedure and/or when hydrolyzing drug-glucuronide conjugates. Instrumental methodology, such as thermal degradation in GC inlets, may also impact certain drugs under certain conditions. Laboratories may need to evaluate the factors that might impact a drug’s stability, regardless of the medium or matrix, if sufficient data has not yet been published. The FDA, states, “The purpose of stability testing is to provide evidence on how the quality of a drug substance or drug product varies with time under the influence of a variety of environmental factors, such as temperature, humidity, and light, and to establish a retest period for the drug substance or a shelf life for the drug product and recommended storage conditions”. (Reference: https://www.fda.gov/downloads/drugs/guidances/ucm073369.pdf (https://www.fda.gov/downloads/drugs/guidances/ucm073369.pdf) ). While the FDA provides guidance for industries on this subject, the Organization of Scientific Area Committees (OSAC) are now providing guidelines specific to forensic laboratories in regards to drug stability testing during method validation. (Reference: https://www.aafs.org/asb-standard/standard-practices-method-validation-forensic-toxicology (https://www.aafs.org/asb-standard/standard-practices-method-validation-forensic-toxicology) ) These recommendations include evaluating factors such as storage conditions, freeze/thaw cycles and processed sample stability. Samples being stored frozen that undergo several freeze/thaw cycles can experience changes in drug stability. During analysis, a number of tests may need to be conducted and several freeze/thaw cycles of the sample may have to be completed, this can lead to drug loss. Buprenorphine, alprazolam, and tramadol are examples of drugs that have shown the potential to become unstable following 2-3 freeze/thaw cycles. A laboratory that utilizes frozen specimens may need to assess these cycles if the existing literature does not sufficiently address the drugs within the scope of testing. Most laboratories run large batches of samples as a part of their normal daily routines, while others may require 24-hour, 7 days'/week operations. In either laboratory environment, the duration of the testing process may become very lengthy. Samples, ready for testing, may remain on an instruments autosampler for several hours while awaiting testing. During instrumental analysis, instruments may also have mechanical issues that could lead to unforeseen delays in the completion of testing. In these instances, the laboratory has to be certain that the additional time samples spend awaiting testing, be it a few hours or a few days, will not affect the outcome of the results generated from a run made at a later time. Drugs that can become unstable after a period of time, such as cocaine, can result in the production of an inactive metabolite from a pharmacologically active parent drug. This instance is a great example of how reported results could change, leading to an incorrect interpretation of the contents of the sample originally obtained. Researching a drug’s stability and performing stability studies should always be considered to provide laboratory personnel with a clear understanding of potential areas of concern. This is critical in forensics to avoid the potential for incorrect results, interpretations and undo legal consequences. Reflection: 1. Define the term negative control. 2. Provide a hypothetical example of a positive control. 3. List three mechanisms of chemical degradation. 4. List three forms of drug contamination. 5. Discuss preventative measures that can be taken to lessen degradation and/or loss of a drug. Module 1: Sample Preparation and Presumptive Tests Page 4 Preliminary Tests Preliminary Tests Principles of Color Tests or Spot Tests These preliminary tests are some of the oldest tests for drug or toxicological identification. Color tests involve the reaction of a drug sample, a protein free filtrate or an extract with a reagent or a series of reagents to produce a color or change in color. These preliminary identifying tests have several advantages: + Such tests are rapid and simple + Sophisticated equipment is not necessary + Require minimal training + Require minimal sample A negative result for a color test may be used to rule out a drug or drug intoxication. Occasionally, the color produced by reaction with a reagent may be specific for a particular drug, but usually the color change is indicative of a class or classes of drugs (hence they are used solely as presumptive tests). Drug color tests can be conducted on about 1 mg of dry sample, aqueous solutions of the sample, solvent solutions of the sample or solvent extracts of the sample, and are conducted in test tubes or on white porcelain spot test plates. Some of these tests are also used as sprays or locating agents for thin layer chromatography. As well as being used for the preliminary identification of chemical substances, with minor modifications, these tests may be used in the preliminary identification of chemical substances in biological fluids, tissue and stomach contents. Here is a brief video illustrating the use of the presumptive Marquis and Mandelin color tests: The Home Scientist 018 - Forensic Presumptive Drug Testing I (http://youtu.be/1DU3R8kkBI8) (http://youtu.be/1DU3R8kkBI8) The Centre for Forensic Science at University of Technology Sydney (UTS), had developed a color test for synthetic cathinones which utilizes copper (II) nitrate, 2,9-dimethyl-1, 10-phenanthroline (neocuproine) and sodium acetate. The color change requires heat and takes approximately 10 minutes to complete, going from light blue to yellow orange. Here is a brief video illustrating the use of this presumptive color test: will require a JoVE login to view (see Modules - JoVE Instructional/Informational Videos to create your JoVE account). Color Spot Test As a Presumptive Tool for the Rapid Detection of Synthetic Cathinones Log in 02:00 We use cookies to enhance your experience on our website. By continuing to use our website or clicking “Continue”, you are agreeing to accept our cookies. &t=1&s=1 Learn More Continue After watching the video go to https://www.jove.com/ (https://www.jove.com/) or click on the video title above to Sign Out of JoVE Some Examples of Commonly Used Drug Identification Color Tests Color Test 1. Begin with an alcohol wiped, 2. Add a few milligrams of the unknown test powder. clean, white spotting tile or dish. 3. Using a Pasteur pipette 4. Add one drop of the spot test solution to the test sample powder on the withdraw a milliliter of spot test clean plate. solution. 5. Observe the color change. 6. In this case the powder becomes blue on addition of cobalt thiocyanate solution indicating the presence of "caine" compounds, possibly lidocaine, procaine or cocaine. Reflection: 1. Why are color tests considered to be preliminary tests and not confirmatory drug identification tests? 2. List two advantages of color tests. 3. Name the color test used to screen for opiate drugs. 4. What color change would you expect with Ehrlich’s reagent in the presence of LSD? Module 1: Sample Preparation and Microscopic Examination of Cannabis Presumptive Tests Page 5 Microscopic Examination of Cannabis In analyzing suspected cannabis samples, a microscopic examination, used to identify the presence of characteristic morphological features, is generally conducted in addition to the Duquenois-Levine color test. Cannabis samples may contain stalks, stems, seeds, flowering parts, and leaves. Microscopically, the leaves consist of covering (clothing), cystolithic and glandular trichomes or hairlike projections found on the surface of various parts of the plant. Cystolithic hairs, found primarily on the upper side of the leaves, are small bear claw shaped hairs, which have a calcium carbonate base (cystolith). Clothing hairs are long, sharply pointed hairs, generally found on the underside of the leaves. Glandular hairs, generally found on flowering and fruiting tops, leaves and stems, have a spherical shaped head and contain an oily resin in which cannabinoids like THC can be found. Below is a microscopic representation of these three types of hairs or trichomes: Reflection: 1. Name the three trichomes or hairs that can be microscopically observed on the cannabis plant. 2. Which cellular structures of cannabis are specific to the flowering and fruiting tops of the plants? 3. Which cellular features of cannabis do not contain cannabis oil? Module 1: Sample Preparation and Microscopic Examination of Cannabis Presumptive Tests Page 6 Microcrystal Tests Developed nearly 170 years ago, these chemical precipitation tests can be used to identify chemical substances in trace or large quantities. The tests produce crystal derivatives from simple molecular addition compounds of the substance tested and a test reagent. Crystals are then observed using a properly aligned and adjusted polarizing microscope. Many microcrystal tests have been considered to be so characteristic that they could be employed as confirmatory tests. However, few analysts have such microscopy or crystallography expertise that they can unequivocally identify a drug microcrystal using microscopy alone. Over the years, these tests have been refined and improved by using precipitating compounds in strong acid solutions instead of aqueous solution. Microcrystal tests are useful for identifying the presence of compounds that contain basic nitrogen and readily precipitate from aqueous solution. These reagents are also reactive with compounds in which oxygen atoms have basic properties making them capable of forming oxonium salts. Direct and Indirect Testing Microcrystal tests for chemical identification purposes can be classified into direct and indirect tests. - Direct tests: include precipitation tests and color tests; the substance being tested is responsible for the crystals produced. - Indirect tests: include negative tests, reagent tests, and derivative tests. 1. Negative tests - tests where the test is the failure of a specific action or reaction to occur. 2. Reagent tests - a color change or precipitate formation is due to some change in the reagent caused by the test substance. 3. Color tests are largely reagent tests and involve a change in color of the reagent by an oxidation or reduction reaction. 4. Derivative tests the drug-reagent derivative formed, becomes the compound to be tested. The tests generally involve adding a drop of reagent to a drop of a solution of the test substance on a flat glass microscope slide and observing the product at frequent intervals until crystals form or the solution evaporates and microscopic information can be acquired. These tests are useful for quick reactions in which the crystals are expected to form immediately or within a matter of minutes. One to ten microliter drops of the drug in solution may be used together with a drop of reagent of similar size. For situations where crystal development takes longer, small drops may be inadequate due to rapid solute evaporation, in these cases the test spot may be placed in a humidified chamber or the reaction medium can be changed to one that evaporates more slowly. The Chemistry of Microcrystal Testing When the test substance has dispersed or dissolved in the test reagent, and the mixture becomes saturated, crystal formation occurs. Supersaturation may occur as a result of evaporation of the solvent, and any impurities present in the test sample will also be concentrated and may hinder or distort crystal development. Traditionally, only direct microcrystal tests have been used in seized drug cases for the preliminary identification of opiates, CNS stimulants, barbiturates, sedatives and tranquilizers. Forensically, the microcrystal test is an efficient means of preliminary identification due to the directness, simplicity, sensitivity and rapidity of the technique as well as the ability to conserve evidence. Quantities needed for identification of alkaloids are in the range of 1 µg to 100 µg. Common Reagents Alkaloid precipitating agents are aqueous solutions of acids, salts or bases with sufficient acid to prevent basic hydrolysis. The agents precipitate ptomaines, proteins, basic nitrogenous compounds or amines. Alkaloids form acid salts that are generally water-soluble; many of the free alkaloids are water insoluble. Tests can be made on an aqueous solution of the free alkaloid if it is soluble. Precipitation tests can also be used, with alkaloids giving precipitates in a number of ways: Reactions with basic reagents may free the alkaloid from its combination with acid The alkaloid may combine with oxygen acids of the metalloids of group's 4,5b, 6a, and 7 of the periodic table Via halogenation By forming double or complex salts with group B metals of the periodic table (salts are halides, cyanides, thiocyanates and nitrates) The alkaloid may react with certain organic compounds that are mostly acidic in nature When the alkaloid-reagent reaction involves the formation of double or complex salts with metals, the precipitating agents are nearly always chloroacids or double chlorides. The most common reagents are gold and platinic chloride. Module 1: Sample Preparation and Presumptive Tests Page 7 Microcrystal Tests Continued Microcrystal Tests Continued Advantages and Pitfalls of Microcrystal Tests + Sensitive and selective + Usually take place spontaneously and rapidly - Impurities, diluents and/or adulterants may interfere leading to distortion or inhibition of crystal formation making identification difficult - An extraction may be needed to remove interferences - Considerable training and experience in chemical microscopy is needed to identify drugs based on the crystal form of the products of specific test reagent reactions - The technique possesses some degree of subjectivity - Crystals of one type may show a wide variation in form - One substance with one reagent may give more than one type of crystal product Microscopy and Crystallography Microcrystal reaction products are usually examined by polarized light microscopy. Without a cover slip, at 100x magnification, crystals can be seen forming at the edge of the reaction drop. Microscopic examination can be used to determine the size and shape (aka habit), color and aggregation characteristics. Distortions of the crystal habit or form resulting in crystal di- or polymorphism, can be caused by: Changes in ambient temperature and humidity Concentration of the reagent or substance tested A colored test reagent may result in the formation of colored crystals. Examination by polarized microscopy shows crystallographic features than may be used to identify specific compounds, such as: Dichroism and pleiochroism Sign of absorption Birefringence Angle of extinction Sign of elongation Refractive index Other microscopic parameters The methods are largely time consuming and require a great deal of expertise and experience in microscopy. Cocaine hydrochloride and chloroauric Morphine sulfate and mercuric acid solution iodide solution For more information regarding microcrystal tests, see McCrone's A Modern Compendium of Microcrystal Tests for Illicit Drugs and Diverted Pharmaceuticals (https://www.ncjrs.gov/pdffiles1/nij/grants/249854.pdf) or the Dennis Kunkel microscopy site: http://www.denniskunkel.com/ (http://www.denniskunkel.com/) for photomicrographs of caffeine, nicotine, aspirin and tetrahydrocannabinol. Florida State University also provides a photomicrograph gallery of a range of pharmaceuticals including cocaine, dimethyltryptamine, and heroin on their Molecular Expression website at http://micro.magnet.fsu.edu/pharmaceuticals/index.html (http://micro.magnet.fsu.edu/pharmaceuticals/index.html) (although these are not necessarily the product of microcrystal testing). Reflection: 1. List the advantages of microcrystal tests in forensic drug identification. 2. Outline the differences between direct and indirect microcrystal testing. 3. List two disadvantages of microcrystal tests. 4. List four crystalloptic parameters that can be used to identify or distinguish drug microcrystals. Module 1 Quiz This concludes Module 1. Please click on the Module 1 Quiz link to take the quiz. Module 2: Sample Extraction & Thin Layer Chromatography Page 1 Module Overview Introduction Hello and welcome to Module 2. In this module you will be introduced to the chemistry of drug ionization, drug extraction and preliminary testing by thin layer chromatography. Our objective is to familiarize you with the methods routinely utilized in the extraction of unidentified chemical substances that may be present in bulk form, in biological fluids or tissues and the methods routinely used to prepare these samples for instrumental analyses. In this module - you will also be introduced to the first part of the case study. Objectives At the end of this module, students will: Understand the principles of drug ionization Understand the principles of partitioning of hydrophilic, lipophilic and amphoteric drugs Understand the principles of methods used to prepare bulk or biological samples for chemical extraction, including liquid-liquid extraction and solid phase extraction Understand the techniques of preliminary drug testing using thin layer chromatography Module 2: Sample Extraction & Thin Layer Chromatography Page 2 Sample Extraction Procedures Sample Extraction Procedures To begin analysis of a case sample, it may be necessary to extract or remove the drug or metabolite (analyte) from interfering compounds (excipients, bodily fluids, tissues, drug mixtures). This is achieved by extracting the analyte from an aqueous solution into an organic solvent. How well the analyte extracts into the solvent depends largely on the degree of ionization of that compound. This in turn is dependent on the pH of the environment in which the compound is placed and its pKa, which can be further explained by the Bronsted-Lowry Theory of Acids and Bases. Bronsted-Lowry Theory of Acids and Bases (Acid-Base Equilibria) An acid is a substance, charged or uncharged, capable of donating a proton: a base is a substance charged or uncharged, capable of accepting a proton from an acid. Acids and bases can be either neutral compounds or charged ions An acidic substance can only behave as an acid in the presence of a base as defined by the Bronsted Lowry theory Similarly a basic substance can only behave as a base when in the presence of an acid A proton can only be donated when there is another substance present to accept it. This may be another dissolved substance or the solvent itself. Many compounds including drugs are acidic or basic when dissolved in water. The relative strengths of acids and bases are measured by the tendencies of these substances to give up and accept protons. Hydrochloric acid is a strong acid in water since it readily gives up its proton, whereas acetic acid is a weak acid because it does not readily dissociate. The strength of an acid, however, varies in different solvents. For example, hydrochloric acid is a weak acid in acetic acid and acetic acid is a strong acid in liquid ammonia. Consequently, the strength of an acid depends not only on its ability to give up a proton but also on the ability of the solvent to accept it. This is called the basic strength of the solvent. The mechanism by which an acid transfers a proton to a base is called dissociation or ionization. Acids and bases are crudely classified as strong or weak depending on the completeness of the ionization process (extent of ionization). Strong acids completely ionized in aqueous solution: HNO3, HCl, HClO4, H2SO4 H2O + HNO3 -> H3O+ +NO3- Weak acids partially ionized in aqueous solution, resulting in a dynamic equilibrium: H3BO3, HCN, H2S H2O + HCN H3O+ + CN- Strong bases completely ionized in aqueous solution: OH- NaOH + H2O -> Na+ + OH- + H2 O Weak bases partially ionized in solution, also resulting in a dynamic equilibrium: NH3 NH3 + H2O NH4+ + OHThese examples of acids and bases are inorganic compounds. There are many examples of organic acids and bases, including drugs and other pharmaceuticals. Acids - amobarbital, aspirin, ibuprofen, penicillins, warfarin Bases - atropine, codeine, epinephrine, fentanyl, streptomycin Other drugs such as steroids and vitamins A, D and E do not have groups that ionize significantly in solution. Module 2: Sample Extraction & Thin Layer Chromatography Page 3 Solvent Effects Solvent Effects Proton transfer can occur in solvents other than water. The relative strength of an acid or base depends on the environment of the acid or base. Examples of Solvent Effects on Acid or Base Strength HCl is a strong (fully ionized) acid in water: H2O + HCl -> H3O+ + ClHCl is a weak acid in glacial acetic acid: HAc + HCl -> H2Ac+ + ClAcetic acid is a weak (partly ionized) acid in water: H2O + HAc -> H3O+ + AcAcetic acid is a strong acid in liquid ammonia: HAc + NH3 -> NH4+ + AcNH3 is a weak base in water: H2O + NH3 -> NH4+ + OHNH3 is a strong base in glacial acetic acid: HAc + NH3 -> NH4+ + AcThe acids in these examples (HCl and HAc) are only able to donate a proton because the solvent can accept protons. This has been proven by experiments in the gas phase where there is no solvent. The strengths of the acids and bases in the gas state are very different to their strengths in the liquid state. The acidity of any acid depends on the basicity of the base that accepts the proton. The basicity of any base depends on the acidity of the acid that donates the proton. Protolytic Reactions Reactions in which an acid reacts with a basic solvent or in which a base reacts with an acidic solvent are known as protolysis or protolytic reactions. These reactions lead to the formation of new acids and bases called conjugates. Every acid in its deprotonated form is called a conjugate base. Similarly, every base in its protonated form is called a conjugate acid. Acid HA +H20 Acid 1 base conjugate H3 0+ +AConjugate acid 2 Conjugate base of 1 Base B +H20 Base 1 Conjugate acid 2 OHConjugate base 2 +BH+ Conjugate acid of 1 Ionization Constants Weak acids and bases are incompletely or slightly ionized in solution. The extent of ionization is described by the equilibrium ionization constant, Ka. Acids [H2O] is included in the ionization constant Ka as [H2O] can be treated as though it were a constant: pKa = -logKa For strong acids, Ka > 1 or pKa < 0 Bases For strong bases, Kb > 1 or pKb < 0 Also, pKa + pKb = pKw = 14 Therefore, if pKb is known for a base, we can calculate pKa for the conjugate acid. Similarly, if pKa is known for an acid, we can calculate pKb for the conjugate base. However, if given the pKa for an unknown drug, we must decide from inspection of other chemical or structural data if the drug is an acid or a base before using the pKa value in calculations. Module 2: Sample Extraction & Thin Layer Chromatography Page 4 Acid Bases and pH Examples of weakly acidic drugs Salicylic acid C7-H6-O3 Barbiturate C12-H12-N2-O3 Examples of weakly basic drugs Cocaine C17-H21-N-O4 Amphetamine C9-H13-N Amphoteric drugs (those having both acid and base properties) Morphine Alkanes Alkenes Alkynes Ketones Amides Ethers C17-H19-N-O3 BZE C16-H19-N-O4 Groups that do not significantly ionize in water -CH2-CH2-CH=CH-C C-CO-CO-NH-CH2-O-CH2- Acid Bases and pH Ionization Constants Weak acids and bases are incompletely or slightly ionized in solution. The extent of ionization is described by the equilibrium ionization constant, Ka. Definition of pH A pH value is a number that is a measure of the acidity of a solution. It is defined by the equation pH = -log10 [H+] This is not strictly correct as a single proton does not exist in aqueous solution but is hydrated: H+ + H2O --> H3O+ (hydronium ion) so pH = -log10 [H3O+] (H+ and H3O+ may be used interchangeably) The pH Scale The range of values for [H+] in aqueous solutions at room temperature (20-30°C) is from 100 - 10-14. Therefore, the pH scale is from 0-14. Note that if an acid is added to water pH < 7 if a base is added to water pH > 7 The pH scale depends on the temperature of the solution for which the pH is being measured. pH + pOH = 14 Henderson-Hasselbalch Equation In reviewing the dissociation or ionization of a drug in solution, the dissociation of a weak acid in solution can be represented by the following: For weak bases, the dissociation in solution can be represented as: The Henderson-Hasselbalch equations for acids and bases can be used to reveal the percentage or ratio of unionized and ionized forms of a drug when in solution. This is important to consider when developing extraction techniques. The Henderson-Hasselbalch equations are used to show the relationship between the pH of the solution or environment the drug is placed in during sample preparation and extraction, the pKa of the drug being extracted, and the ratio of the unionized and ionized forms of that drug. Using this equation, both acidic and basic drugs can be discussed, or compared, in terms of their pKa’s. Knowing the above equations for acids and bases, a more explanatory way to write the HendersonHasselbalch equations in discussing their importance to extraction processes is: Using the drug’s pKa and the pH of the solution (or the environment) the drug is in, the ratio or percentage of the ionized and unionized forms of the drug can be determined. For example, if the solution the drug is in has a pH of 3 and the drug’s pKa is 3, then the log of [A-]/[HA] or [BOH]/[B+] would be zero, meaning the concentrations of each and/or ratios are equivalent; that is, 50% of the drug will be in an ionized form and 50% in an unionized form. When the pH changes, the drug’s state of ionization will be different depending on it being an acid or base. Keeping the above equations in mind, here are a few additional examples for an acidic drug and a basic drug under varying pH environments. If an acidic drug such as aspirin, which has a pKa of 3.5, were in a solution with a pH of 1.5, the ratio of ionized to unionized would be equal to one. Using the Henderson-Hasselbalch equation to show this calculation: This means that for every 1 ionized molecule in the solution, there would also be close to 100 unionized molecules. Additionally, if the solution’s pH were higher than aspirin’s pKa, for example, 5.5, the result would be just the opposite, with 1 unionized molecule for every 100 ionized molecules in the solution. If a basic drug like cocaine, with a pKa of 8.6, was in a solution with a pH of 10.6, the ratio of unionized to ionized would be 100, meaning for every 1 ionized molecule, there would be close to 100 unionized molecules. If the solution’s pH were 6.6, then for every 1 unionized molecule there would be 100 ionized molecules. These examples were used to specifically to show that when the pH and pKa are at least two units apart, the drugs of interest would be close to 100% unionized or 100% ionized, depending on the scenario. The importance of this pH and pKa relationship and how it can be used in extracting drugs of interest will be clarified further in the module’s discussion of specific extraction techniques. This relationship is also important in pharmacology as the state of the drug, ionized or unionized, will determine how the body will absorb, distribute and eliminate the drug. Reflection: 1. What are the definitions of an acid and a base? 2. What is the term given to the mechanism by which an acid transfers a proton to a base? 3. What is the Bronsted-Lowry theory? 4. What is the Bronsted-Lowry definition of acids and bases? 5. What are Henderson-Hasselbalch equations for acids and bases? 6. When extracting an acidic drug with a pKa of 3, what should the pH be to extract the unionized form of the drug, primarily? 7. Give an example of an acidic and a basic drug. 8. Answer True or False - If the Ka = 0.001, the drug is a strong acid. - If the pKa is 4.0, the drug is a weak acid. - pH is dependent upon temperature Module 2: Sample Extraction & Thin Layer Partitioning (Extractions) Chromatography Page 5 Partitioning (Extractions) Liquid-Liquid Extraction (LLE) If an immiscible organic solvent is added to an aqueous solution containing a drug, the drug will partition between the two phases. A partition coefficient (K) can be calculated from the concentration of the drug in the organic phase divided by the concentration in the aqueous phase. This partition coefficient is dependent on the nature of the drug and the organic solvent, temperature and the degree of ionization of the drug. The degree to which a drug partitions between an aqueous and an organic medium depends on the relative hydrophilic ("water-loving") and lipophilic ("fatloving"), nature of the compound. Polar drugs, ie those with ionized functional groups such as OH-, N+ tend to preferentially partition into the aqueous phase; whereas, non-polar compounds will partition into the organic solvent. When a drug enters the body, metabolic processes, occurring primarily in the liver, act to modify the drug to make it as polar as possible, enhancing its partitioning into the aqueous phase, leading to its subsequent elimination in the urine. When conducting an extraction to remove a drug from a biological matrix, the drug must be made as non-polar as possible so that it can be extracted into the organic phase, which is more suited for instrumental analysis. K = C1/C2 K = Equilibrium Constant C1 = Concentration of drug in organic layer C2 = Concentration of drug in aqueous layer Drugs partition between aqueous and non -aqueous solvents. A compound that is ionized (hydrophilic) has a greater affinity for the aqueous phase than an unionized (lipophilic) compound, which will tend to move into the organic phase. The degree of ionization depends on the pH of the environment. For acids: H+ + A- HA unionized ionized organic soluble water-soluble Add acid and extract with organic solvent (ex. anti-inflammatory drugs and barbiturates). For bases: BOH B+ + OH- Add base and extract with organic solvent (ex. cocaine, amphetamine, codeine) Amphoteric compounds such as morphine and benzoylecgonine exhibit both acid and base properties. While these compounds can be extracted to some extent using acid or base extraction procedures, optimal extraction can only be achieved by carefully adjusting the pH to get the drug into an unionized form. For morphine, this is around a pH of 8. Here is a short video demonstrating liquid-liquid extraction using a separatory funnel: Exp 2 Sep Funnel (http://youtu.be/-1euX89H100) (http://youtu.be/-1euX89H100) Advantages of Liquid-Liquid Extraction -Low volumes of solvent in smaller scale extractions such as with LCMSMS sample preparation methods -Vast amount of literature references to assist in deciphering appropriate conditions for use -Relatively simple to conduct -Low cost -Capable of being optimized for different classes of drugs Disadvantages of Liquid-Liquid Extraction - Immiscible solvents are required - Time consuming and cumbersome in some instances - Glassware clean up required - Larger volumes of solvents needed for traditional methods - Less selective than other extraction methods For these reasons a great deal of extractions are now achieved using solid phase extraction. Solid Phase Extraction (SPE) Solid phase extraction also involves the partitioning between two phases, specifically, a liquid phase and a stationary sorbent phase contained in a polypropylene tube. The stationary phase consists of bonded silica sorbent material held in place by an upper and lower frit. The mobile phase being the liquid sample and various solvents used. During this extraction process, pH adjustments are made in order to isolate the drug(s) of interest. Solid phase cartridges are manufactured to contain different types and amounts of sorbent bed for use in a variety of applications. Solid phase extractions were first developed from the principles of High Performance Liquid Chromatography separations (HPLC). For this reason, many of the early extraction procedures described in the literature described the use of C18 sorbents as the extraction media; however, many different sorbents are now available, allowing a great degree of flexibility and selectivity in the extraction approach. The selection of the solid phase cartridges used is critical to the application and should include an understanding of the interactions that take place between the drugs of interest and the solvents with the sorbent bed chosen. Below are some common types of solid phase cartridges: Types of Bonded Phases: Non Polar Phases Bonded Phase Volume C18; 100 mg 1.0 ml C18; 200 mg 3.0 ml C18; 500 mg 6.0 ml C8; 200 mg 3.0 ml C8; 500 mg 6.0 ml Cyclohexyl CH; 200 mg 3.0 ml Ethyl C2; 200 mg 3.0 ml XAD-2; 1000 mg 5.0 ml Alumina Phases (Basic); 100 mg 1.0 ml (Acidic); 100 mg 1.0 ml (Neutral); 100 mg 1.0 ml Polar Phases Diol 2(-OH); 200 mg 3.0 ml Silica; 200 mg 3.0 ml Cyanopropyl; 200 mg 3.0 ml Ion Exchange Phases Anion, SAX; 200 mg 3.0 ml Cation, SCX; 200 mg 3.0 ml NH2; 200 mg 3.0 ml Mixed Mode Phases SCX + SAX; 200mg 3.0 ml SCX + C8; 200mg 3.0 ml SAX + C8; 200mg 3.0 ml A typical solid phase extraction sequence is described on the next page. Module 2: Sample Extraction & Thin Layer Chromatography Page 6 Extraction Sequence Extraction Sequence Biological samples must first be pretreated in preparation for solid phase extraction. This step ensures the samples will flow easily through the sorbent bed and will be in the pH needed for the retention of the drugs of interest during the initial step of the extraction process. Sample pretreatment can involve protein precipitation, dilution, hydrolysis, homogenization, sonication, and centrifugation of the sample. Conditioning The sorbent must also be pretreated or “conditioned” to provide an environment suitable for the reproducible retention of all analytes of interest. This is achieved by washing the bed with a suitable solvent, such as methanol. Methanol is particularly effective since it can interact with both the silanol and the carbon groups that make up the bonded phase. Several other solvents can also be used for this purpose including acetonitrile and isopropanol. As this is critical to retention, once wetted, the sorbent bed should not be allowed to dry out. Change of pH Environment A buffer is then passed through the extraction cartridge to introduce a pH environment conducive to the retention of the analytes of interest onto the column. For example, if a cation exchange column is being used for the extraction of basic drugs, an acidic environment will cause the drug to become positively charged and, therefore, be retained by the stationary phase. Sample Application (Load) Again without allowing the column to dry out, the sample is applied and pulled through the cartridge. The vacuum would be adjusted to allow the sample to pass through the column in a dropwise fashion. Rinse (Wash) Endogenous compounds present in the sample matrix, as well as analytes in which we have no interest can then be removed by washing the extraction cartridge with a suitable solvent. Any combination of aqueous and/or organic solvents can be used for this purpose, the goal being to remove as much of the weakly retained interferences as possible without eluting the analytes of interest. The sorbent bed would then be dried for a period of time. Elution Finally, the analyte of interest is eluted from the column and collected into a tube using a suitable solvent. The strength of the eluting solvent can be adjusted to optimize the elution of the analyte of interest while at the same time minimizing the amount of endogenous material removed from the column. Here is a brief YouTube video outlining the steps used in SPE: SPE Steps (https://www.youtube.com/watch?v=Gkdow0i4F68) (https://www.youtube.com/watch?v=Gkdow0i4F68) A more detailed video of solid phase extractions can be found on National Institute of Standards and Technology’s (NIST)’s website: Solid Phase Extraction (https://www.nist.gov/video/solid-phase-extraction). Solvent strength can be determined by reference to an elutropic series. For example, in the following series, hexane is the least and water the most polar solvent. The polarity of aqueous solvents can be increased further by the addition of acids or bases. See the video below on Separation/Extraction Techniques. Module 2: Sample Extraction & Thin Layer Chromatography Page 7 Example of a Solid Phase Extraction Example of a Solid Phase Extraction The following is an example of a solid phase extraction procedure that can be used for samples containing acidic and/or basic drugs. The solid phase cartridges used for this extraction consist of a mixed mode sorbent with an octyl-bonded phase (C8 chain) with a strong cation exchanger (benzene sulfonic acid) sorbent bed. Knowing the pKa of the drugs you are extracting for and the pH of the sorbent environment you create during the process, will allow you to know the degree to which the drugs will be ionized and how they will interact with the sorbent bed. This will also be important to know during wash and elution steps in determining if the drugs will be retained or eluted from the bed. A guideline to consider is that when the pH of the environment is at least two units or above the pKa of a basic drug, the basic drug will be close to 100% unionized. When the pH is 2 units below an acidic drug’s pKa, the acidic drug will be close to 100% unionized. Drugs are easily eluted with organic solvents when unionized and the extraction efficiency will be at its best under these conditions. Step 1: Sample Pretreatment Add 3 mL of sample to a capped tube. Internal standard solution is added followed by 3 mL of 0.1 M (pH = 6.0) potassium phosphate buffer. The samples can be vortexed and centrifuged. Explanation: This addition of the buffer places the sample’s drugs in an acidic environment. The basic drugs will be positively charged and attach to the cation exchange portion of the sorbent. The acids will be unionized and attach to the hydrophobic C8 chain of the sorbent. Step 2: Column Conditioning 1) Add 2 mL of methanol to sorbent bed under vacuum while ensuring the bed does not dry out. 2) Add 2 mL of 0.1 M phosphate buffer (pH = 6) passed through sorbent bed under vacuum while ensuring the bed does not dry out. Explanation: The methanol rinse opens the coiled hydrophobic portion of the phase (C8 chain) and activates it toward interaction with a polar matrix (blood and urine). The phosphate buffer removes excess methanol and places the sorbent bed in an environment as similar to the matrix as possible. If the bed dries out, resolvate or start over with a new cartridge. Step 3: Add Sample Apply sample onto column under vacuum that’s adjusted to allow the sample to pass through the outlet of the cartridge dropwise. Explanation: Setting the vacuum at a pressure that allows the sample to pass through in this manner will ensure that the sample has sufficient time to interact with the sorbent bed. Step 4: Wash Step Add 1 mL of deionized water thru column under vacuum. Explanation: This wash step will rinse away anything not adsorbed, including interferences from the blood and urine matrices such as fatty acid, salts proteins and carbohydrates. Step 5: Acidify the Sorbent Bed Add 0.5 mL of 0.01 M acetic acid (pH = 3.3) through the sorbent bed under vacuum Explanation: This step will complete the removal of any remaining polar interferences. As the pH is more than 2 pKa units lower than any basic drug, this step ensures that the basic drugs become positively charged and will be retained by the cation exchange portion of the stationary phase. For the same reason, the acidic drugs would be in an unionized form making them organic solvent soluble and would be attracted to the hydrophobic portion of the stationary phase (C8 chain). Step 6: Dry the Sorbent Bed 1) Dry the sorbent bed under full vacuum for at least 4 minutes 2) Add 50 μL of methanol to the sorbent bed under no vacuum 3) Dry the sorbent bed again under full vacuum for 1 minute Explanation: These three steps of drying the column are necessary to ensure that all water is removed from the sorbent bed because water can interfere with the contact the organic solvent will have with the sorbent during the elution steps. Step 7: Elute Acidic Drugs Add 4 mL of 1:1 acetone/chloroform to sorbent bed under vacuum Explanation: Nonpolar solvents will disrupt the acid’s nonpolar interaction with the sorbent’s C8 chain and will wash any acidic drug into the collection tubes. The ionized basic drugs would be retained by sorbent’s cation exchange. Step 8: Elute Basic Drugs Add 4 mL of 2% ammoniated ethyl acetate (pH = 12) to sorbent bed with no vacuum Explanation This step ensures that the basic drugs become unionized by raising the pH and can then be removed by the organic solvent. No vacuum at this step ensures that more volatile drugs are not lost during this portion of the process. Note: Studies are generally conducted to determine the best choice of elution solvent systems to ensure the most efficient extraction process. Protein Binding Some drugs are significantly bound to proteins in the blood. In order to liberate sufficient drug from the proteins to allow its successful analysis, it is often necessary to treat the biological sample prior to its extraction. Heating with acids or incubating with a proteinase enzyme can be used to liberate protein bound drugs. Whether or not this is necessary and the approach taken depends on the nature and stability of the analyte in question. Percent Recovery or Extraction Efficiency The percentage recovery of an extraction procedure can be calculated by spiking a sample for example urine with a known concentration of the drug or drugs of interest. After extraction, the extract is analyzed and the concentration of the drug is determined. If the urine sample was spiked with 500ng/ml of cocaine and after extraction the concentration of cocaine in the extract is determined as 400ng/ml, the extraction efficiency of the method is 400/500 = 80%. Note that this is only theoretical extraction efficiency since the drug has not passed through the body and has not therefore been subject to metabolism or protein binding which will reduce the efficiency of the extraction. Additional Solid and Liquid Phase Extraction Techniques QuEChERS stands for Quick, Easy, Cheap, Effective, Rugged and Safe and can be used as a low cost sample cleanup method for the analysis of biological samples. This technique combines liquid- liquid and dispersive solid phase and has been used primarily for pesticide residue analysis in the food safety industry and more recently by labs testing cannabis for pesticides. Here is a link that provides some insight into how this technique is performed by labs for the food industry: Water's QuEChERS-Dispersive Solid Phase Extraction (http://www.waters.com/waters/en_US/QuEChERS-Dispersive-Solid-Phase-Extraction/nav.htm? cid=10072671&locale=en_US) Microextractions Liquid and solid based microextractions are also used by many laboratories to extract drugs of interest in preparation for qualitative and quantitative instrumental analysis. Microextractions alleviate the use of large amounts of solvents and dealing with subsequent waste, as would be the case with standard SPE or LLE. These techniques are rapid, less expensive, and use minimal sample volumes. Concerns about the sorbent bed drying out or maintaining flow rate through the sorbent bed are resolved, as no vacuum manifold is needed. An evaporation step is also not needed which precludes any potential loss of volatile compounds of interest. In solid phase microextractions (SPME), analytes adsorb onto the stationary phase, just like solid phase extractions; however, there are normally no solvents used during the process. With this technique, the solid phase is coated on a small silica fiber attached to a syringe. With this technique, a small portion of the sample is placed in a capped vial. The syringe with the fiber attached is either placed in the sample solution or it is positioned in the headspace above the solution. The fiber remains in place for a period of time, allowing the sample to adsorb onto the coating of the fiber. That fiber is then removed and can be directly inserted into the injection port of an instrument where the drugs desorb and are analyzed. The advantages are that there are no solvents needed in this process and it is much simpler and faster than traditional SPE. More recently, methods for the detection of synthetic cannabinoids and cathinones have been developed using SPME techniques. Another solid phase based technique is microextraction by packed sorbent (MEPS). The solid phase is packed into a syringe in this instance, which can be used directly with instrumentation. Instead of being a single-use cartridge like those in SPE, these can be used more than 100 times. Liquid phase microextractions (LPME) use solvents to extract the drugs; however, a very small amount of solvent is needed- microliters versus milliliters. There are several types of liquid microextractions to include single-drop microextraction (SDME), hollow-fiber supported LPME (HFLPME), and dispersive liquid–liquid microextraction (DLLME) along with variations of each. For more on forensic microextractions see LCGC’s article, “ Microextraction and Its Application to Forensic Toxicology Analysis (http://www.chromatographyonline.com/microextraction-and-its-application-forensic-toxicology-analysis-0? pageID=1) ” Advantages Advantages of solid phase compared to liquid -liquid extractions: + Selectivity - Extraction processes can be designed to selectively extract an analyte or class of analytes. + Reproducibility - SPE eliminates emulsion formation; reduces the dependence on operator technique; improves extraction reproducibility. + Speed - Vacuum manifolds allow the simultaneous extraction of multiple samples; low volumes of solvent employed reduce the time for evaporation steps; SPE techniques are easily automated unlike liquid extractions Disadvantages -Requires additional equipment such as vacuum or positive pressure manifold -Can require more solvents than LLE with larger volumes (our buprenorphine blood quant puts any LLE to shame on this one) -Additional sample preparation may be needed -Cartridges can clog with viscous samples -Operator dependent (some skill involved) Reflection: 1. Define extraction efficiency. 2. List three disadvantages of liquid-liquid extraction. 3. What effect could protein binding have on drug extraction and what steps can be taken to overcome this? 4. Why is it important to dry a sold phase cartridge prior to eluting drugs of interest with organic solvent? Module 2: Sample Extraction & Thin Layer Chromatography Page 8 Thin Layer Chromatography (TLC) Thin Layer Chromatography (TLC) TLC is one of the most widely used techniques for the separation and identification of drugs and can be applied, to pure drug, drug extracts and extracts of body fluids and tissues. This technique maintained popularity due to its simplicity, reliability, cost effectiveness and selectivity. TLC is a form of chromatography that uses a mobile phase moving across a thin, uniform layer of finely divided stationary phase by capillary action. Commercially available stationary phases are bonded to a glass plate or a plate made of some other supporting material. A mixture of drugs or drug extract is applied to the base of the plate and developed using the mobile phase. The primary use of TLC is as an identification or separation technique. Drugs migrate up the plate at different rates to different locations, enabling separation of drug mixtures due to factors such as: Drug solubility in the mobile phase Drugs hydrogen bonding capabilities Degree of drug ionization (pKa) Advantages + Ability to run several samples simultaneously + Apparatus and the TLC equipment is cheap and simple + Technique offers great flexibility in the choice of mobile and stationary phases + Any sample dried on the plate will remain there + Dried sample is not prone to detection problems associated with non-elution, thermal instability and masking by the solvent front Disadvantages - Lower sensitivity and resolution than gas chromatography and high performance liquid chromatography - A fair amount of skill and experience is necessary to conduct the procedure and interpret the results - Results may be more subjective and dependent on the operator Stationary Phases Most stationary phases are adsorbents (silica and alumina) and drug separation is achieved via interactions between the drug and the surface of stationary phase particles. Stationary phases like cellulose work via a partitioning process between the stationary phase and the mobile phase, but there is usually a combination of interactions with both adsorption and partitioning occurring. When the stationary phase is more polar than the mobile phase this is referred to as a normal phase system. When the mobile phase is more polar than the stationary phase this is referred to as a reversed-phase system. Below are examples of widely used stationary phases: Stationary Phase Properties Silica gel Hydrated silicic acid; weakly acidic in nature; very popular adsorbent, due to hardness and resilience; composed of uniformly distributed particles, 20µm in diameter Diatomaceous earth a.k.a Kieselguhr; naturally occurring amorphous silicic acid from diatom skeletons; less adsorptive properties than silica Magnesium silicate a.k.a Florisil; often used as a last resort when other stationary phases have failed Cellulose Use partition process for separation; 50µm spherical particles optimal; run more slowly than silica plates of similar thickness Reversed-Phase Originally silica plates impregnated with heavy oils, paraffin, silicone oil and fats; good separation; slow to run, irreproducible; silica with long chain hydrocarbons bonded to the hydroxyl group (C18) produce high, reproducible resolution; methanol: water, acetonitrile: water often used as mobile phase Ion exchange resin Cation or anion exchange resins containing quaternary ammonium or sulfonic acid groups for ion exchange bonded to surface of plate; good separation of high MWT substances, and amphoteric compounds; mobile phase usually strong acid or alkali Module 2: Sample Extraction & Thin Layer Chromatography Page 9 TLC Continued TLC Continued Mobile Phase Mobile phase composition is dependent on the class/classes of drugs to be separated and the type of stationary phase chosen. Once the stationary phase is determined, mobile phases of increasing elution strength can be used until separation is achieved. Ion pairing agents can be added to the mobile phase for separation of drugs that are strong anions or cations. Mobile phase solvents should be pure and non-reactive with the drug substances being tested. Running TLC's Video 0:00 / 2:15 Locating Agent Identification Iodine vapor Brown spots appear in presence of any drug pH indicators, Bromothymol blue Yellow in acidic medium and blue in alkaline medium FPN reagent Phenothiazine Dragendorff spray Alkaloids Marquis reagent Numerous colors with different drugs Photographic film Radiolabeled drugs or metabolites A brief video describes the steps involved in preparing, developing, and visualizing solutions using thin layer chromatography: Thin Layer Chromatography (TLC) (http://youtu.be/rbp_Qc4jMAc) (http://youtu.be/rbp_Qc4jMAc) Rf Values The Rf value is the basic chromatographic measurement of a substance in TLC. Rf is defined as the distance the substance travels from the origin divided by the distance the solvent front travels from the origin. The distance traveled by the substance is measured from the center of the spot if the spot is round. For spots with tails the distance traveled should be determined from the center of the densest area. Rf= Distance the substance travels form the origin Distance the solvent front travels from the origin The value varies from 0 to 1, but usually Rf x100 is reported to avoid using decimals As the reproducibility of Rf values can be altered by a number of factors, the test sample should always be run with appropriate controls or reference standards on the same plate. Factors that affect reproducibility of Rf values are outlined in the table below: Factors Affecting Problem Reproducibility Stationary Phase Quality of binder or adsorbent, impurities in phase or differences in thickness of phase on the plate Mobile Phase Impurities in solvent - must prepare daily Developing Tank Ill-fitting lid, unequilibrated solvent vapors Temperature Solvents run faster when warm, volatile substances evaporate faster and Rf values tend to decrease Development Distance Ok if system kept saturated or get increased Rf with increase in running distance due to evaporation of solvent from upper part of plate Mass of sample Increased mass on plate will increase Rf, gross overloading will decrease Rf and result in spot tailing Reflection: 1. List the advantages of TLC. 2. Describe the properties of the following stationary phases used in TLC: Cellulose Silica gel Ion exchange resins 3. Name three locating agents used in TLC and their properties. 4. Define Rf Value. 5. Describe three parameters that affect reproducibility of a drugs Rf value in TLC - and describe their associated problems. Module 2 Quiz This concludes Module 2. Please click on the Module 2 Quiz link to take the quiz. Module 3: Immunoassays Module Overview Page 1 Introduction Welcome to Module 3 - Immunoassays. In this module, we will introduce you to the scientific principles, advantages, and disadvantages of several different immunoassay techniques used for screening drugs of abuse in body fluids such as blood and urine. Objectives At the end of this module students should: Understand the chemistry and conceptual principles of immunoassay techniques as they pertain to forensic toxicology analysis Understand the applications, advantages and disadvantages of immunoassay techniques utilized for the analysis of drugs and their metabolites in body fluid specimens Understand the processes involved in and the differences between the presented immunoassay techniques Understand the sample preparation required for testing samples using immunoassay techniques Understand the need for controls, and calibrators when performing immunoassay techniques Understand the terminology associated with immunoassay techniques Module 3: Immunoassays Principles Page 2 Principles When a foreign entity, such as a virus, enters an animal’s or human body an immune response is initiated. This immune response involves the body’s generation of antibodies specifically designed to bind to and assist with the deactivation and elimination of that foreign invader or antigen. This immune response, involving the formation of an antibody-antigen complex, is the underlying scientific basis for all immunoassay techniques. Production of Antibodies Used for Immunoassay Techniques The specificity an antibody has for a particular antigen is important to note. With this knowledge, manufacturers of immunoassay testing products create antibodies used for specific applications such as screening blood and urine samples for drugs of abuse. Antibodies are commercially produced through the immunization of animals (mice, rabbits and sheep, for example) with the antigens or drugs of interest. While this immunization process involves various steps to complete, ultimately, specific antibodies, used for testing antigens are produced. There are some additional considerations that may also come into play during the antibody production process. Drugs, for example, are considered haptens; that is, their molecular structures are too small to elicit an immune response on their own. They must be linked to a larger molecule, such as a carrier protein, for an immune response to be initiated. Some examples of carrier proteins, used commonly by manufacturers to create haptenprotein conjugates, are bovine serum albumin (BSA) and keyhole limpet hemocyanin (KLH). Following the immunization process, two types of antibodies, polyclonal and monoclonal, may be produced depending on the application needed. Polyclonal antibodies can be generated by the animal in-vivo following immunizations used to induce and enhance their production. These antigen-specific antibodies can then be extracted from serum drawn from the animal, which is also known as antiserum. The production of monoclonal antibodies also begins with an antigen immunization process; however, in this instance, specific antibody producing cells are removed from the animal’s spleen or lymph nodes and the monoclonal antibodies are produced in vitro through a tissue culture process. During this process, antigen specific cells are fused with myeloma cells creating cloned cells or hybridomas that can be used indefinitely for creating large amounts of monoclonal antibodies. The discovery of producing monoclonal antibodies in this manner resulted in a Nobel Prize for scientists César Milstein and Georges J. F. Köhler. The Antigen-Antibody Conjugate & Cross-Reactivity How the attachment between antigens and antibodies proceeds is the basis for immunoassay testing. Antigens have several structural sites that antibodies can link to, known as epitopes. Antibodies have only two structural sites, or paratopes, capable of linking to their respective antigens’ epitopes. Polyclonal antibodies are capable of linking to several of an antigens’ epitopes. Monoclonal antibodies, however, can only attach to one specific epitope on the antigen. Polyclonal antibodies have greater affinity for an antigen than monoclonal due to their multisite linkage. Additionally, as polyclonal antibodies are not specific to one epitope, they are also capable of cross-reacting with other antigens of similar molecular structure. For an unknown drug sample, polyclonal antibodies would be preferable to use as they can enhance the detection response due to their affinity for multiple epitopes. While cross-reactivity is also a possibility for monoclonal antibodies, it is to a much lesser degree due to their specificity for particular epitopes. During immunoassay testing for drugs, cross-reactivity can lead to a class of drugs being detected versus a specific drug of interest, which can lead to a change in the amount of antigen reported. Benzodiazepines, as well as many of their metabolites, are similar in molecular structure making them a good example of a class of drugs that may cross-react during immunoassay testing. When more than one benzodiazepine is present in a sample, there can be an increase in the immunoassay’s measured response while also potentially changing the interpretation of the test results. It is possible, in a case like this, to have a positive immunoassay result due to cross-reactivity that may not yield secondary confirmatory results, as the concentration of each of the benzodiazepines in the sample, on their own, may be too low to detect, but together cause a positive immunoassay screening result. Manufacturers of immunoassay kits do generally provide inserts that include information regarding the cross-reactivity of other drugs with similar chemical structure. The percent cross-reactivity reported by the manufacturer is normalized to the drug used as the calibrator for a specific immunoassay kit. For example, Oxazepam is often used as the calibrator for Benzodiazepine immunoassay kits and would be assigned a cross-reactivity of 100%. Comparatively, Alprazolam might have a cross-reactivity of well over 100% while a drug like Lorazepam might have a cross-reactivity of less than 10%. In both cases, this information would normally be considered when interpreting results for an unknown sample. Table of Cross-Reacting Substances* *Adapted from: Principles of Forensic Toxicology, American Association for Clinical Chemistry, 2020 Labels Immunoassay techniques typically used in a forensic toxicology laboratory may employ labeled antigens (competitive immunoassays) or labeled antibodies (noncompetitive immunoassays), depending on the technique chosen. The labels attached to the antigens and antibodies are important to these techniques as they serve as markers that aid in detection. Their detection can then be correlated to the presence of drugs (unlabeled antigens) contained in the sample. The name of the immunoassay technique provides insight into the type of label each uses during testing. For example, during Enzyme-Linked Immunosorbent Assay (ELISA), an enzyme label is used capable of producing a color change creating a measurable absorbance response detectable by a spectrophotometer. As you will see in later reading, the presence or activity of these labels can create a measurable response be it radiation, absorbance, or fluorescence. Competitive versus Noncompetitive Immunoassays Immunoassay techniques can be classified as competitive or noncompetitive. Competitive immunoassays involve competition between labeled antigens and the sample’s antigens for antibodies. Once this competitive interaction has had time to come to equilibrium, the process involved in detection can proceed. If there are a larger number of labeled antigens attached to the antibodies, then there will be less of the sample’s antigens attached to antibodies and vice-versa. The signal produced by the instrument during detection of the labeled antigens will be reflective of the amount of antigens that are in the sample. In some immunoassays the amount of drug is directly proportional to the instrumental response and in others, the amount is inversely proportional. Noncompetitive immunoassays, in comparison, involve only the binding of labeled antibodies and the sample’s antigens; that is, there is no addition of or competition with labeled antigens. The instrumental response will only be dependent on the labeled antibodies. Overall, competitive immunoassays are used more routinely in forensic toxicology laboratories and will be the majority of the immunoassay techniques discussed in this module. Additionally, how instrumental response relates to the amount of drug in the sample will also be further discussed within each immunoassay technique’s description. Heterogeneous versus Homogenous Immunoassays Additionally, immunoassays can either be heterogeneous or homogenous. Heterogeneous immunoassays require wash steps so that anything in solution that is unbound that could interfere with detection is removed prior to measurements being taken. In homogenous immunoassays, this separation step is not needed, as the unbound material does not affect the instrument’s measurement of the bound labeled antigens (competitive immunoassays) or antibodies (noncompetitive immunoassays). Reflection: 1. Define the following terms: Antigen Antibody Hapten Polyclonal antibodies Monoclonal antibodies Label Substrate Cofactor 2. Describe competitive versus non-competitive immunoassays. 3. Describe the difference between a heterogeneous and homogenous immunoassay. Module 3: Immunoassays Techniques Page 3 Techniques All immunoassays are based on the formation of an antigen-antibody complex with the addition of some form of label, be it a radioisotope, fluorophore, enzyme, or microparticle, that will ultimately be the source for detection and measurement of antigens (drugs) contained in samples being analyzed. Immunoassay techniques are primarily used in forensic toxicology laboratories as screening procedures, but can also be used for quantitative analysis. These techniques can be used for analyzing several types of body fluids including blood, plasma, serum, oral fluid, cerebrospinal fluid, vitreous humor, tissue homogenates and urine specimens. As with most analytical techniques, immunoassay technology has and continues to evolve to include increased automated processing and improved sensitivity and specificity for drugs of interest to forensic toxicology laboratories. While there are several types of immunoassay techniques in use, the focus will be on those commonly used by forensic toxicology labs. Enzyme-Linked Immunosorbent Assay (ELISA) is one of the most commonly used immunoassays in forensic toxicology laboratories and the variations used cover several aspects of immunoassay testing previously described. As with all immunoassays, ELISA is dependent on the formation of antibody-antigen complexes. ELISA is a heterogeneous immunoassay and may be competitive or noncompetitive. ELISA uses an enzyme label as well as a substrate for antigen detection. Substrates are chemicals used to react with the labels in order to produce the optically detectable change required for instrumental response. For example, the substrate and enzyme label interact to cause an initially clear solution to turn blue. A stop reagent, generally an acid, must be added following the substrate to stop the reaction from continuing. This addition also turns the blue solution to a yellow color. This visual change may be different depending on the enzyme label and substrate used. Ultimately, from this reaction, absorbance at specific wavelengths can be read by a spectrophotometer and the amount of the drug of interest determined. While there are several ELISA variations or formats available, competitive ELISA techniques are primarily used in forensic toxicology laboratories. The below example provides a typical competitive ELISA procedure. Competitive ELISA The samples are added to the antibody-coated wells followed by the enzyme conjugate (enzyme-labeled antigens). The enzyme conjugate and sample’s antigens then compete for binding for available antibodies. Once this competitive binding process has had time to complete, anything left unbound in the well is then washed away so that only the bound antigens from the sample and bound labeled antigens are remaining. Following the wash step, a substrate is added, which reacts with the enzymes and any remaining labeled antigens bound to antibodies. After a specified incubation period has passed, a stop reagent is added to complete the color reaction process. Detection includes a measurement of the now colored product and the absorbance response for this competitive ELISA technique will be inversely proportional to the amount of drug in the sample. If there were a high concentration of drug in the sample, the antibodies in the well would be bound to the drug and little to no enzyme conjugate would remain after the wash step to cause a color change with the substrate. If there were a low concentration or no drug in the sample, the antibodies in the well would be bound to a large amount of enzyme conjugate resulting in significant color change with the substrate. Commercially produced kits are generally used, which simplify the processing by containing polystyrene plates with pre-coated antibodies as well as buffers, diluents, labeled standards (specific to the drug of interest) and substrates. ELISA testing may proceed using a simultaneous technique, in which all solutions (sample’s antigens, respective antibodies and labeled antigens) are added at one time. ELISA may also proceed using a sequential addition in which the sample’s antigens are first incubated with their respective antibodies prior to the addition of labeled antigens. Radioimmunoassay (RIA) – RIA is a heterogeneous immunoassay and was the first immunoassay technique, developed in 1959. RIA can also be used as competitive technique to include radioactive labeled antigens competing with sample’s antigens for binding sites of the antibodies. RIA utilizes a radioisotope label thus the detector used must be able to detect radiation with the use of a gamma counter, for example. No substrate is needed for this immunoassay, but several wash steps must be completed during the process and prior to analytical measurements. This is critical to this technique because there is no difference in the signal produced by bound and unbound labeled antigens, which together in solution would lead to a falsely low response for the sample’s antigens present. This immunoassay technique is rarely, if ever used today due to the potential for radiation exposure to personnel and waste disposal requirements. Enzyme-Multiplied Immunoassay Technique (EMIT)- Unlike RIA and ELISA, EMIT is a homogenous assay and was developed in the 1970s. Methods using this technique have been developed most recently for screening samples for synthetic drugs, like synthetic cannabinoids. Like the other techniques, the reacting solution will contain antibodies for the drug of interest, and antigens from the sample being tested. This is a competitive immunoassay so the secondary step in the process is the addition of enzyme labeled antigens. In this technique, following the binding process, a reagent is added that contains substrates and cofactors. When these are added, the enzyme-labeled antigens first oxidize the solution’s substrates. This immunoassay is named “enzyme-multiplied” as the enzyme will continue to react with the substrate until the substrate is exhausted. The substrate reagent also includes a cofactor, which is added to combine with the hydrogen that is released during the oxidation of the substrate. It is the amount of reduced cofactor that is ultimately measured. A large amount of reduced cofactor present would be indicative of a higher concentration of sample’s antigens present, binding to the solution’s antibodies. With a large amount of cofactor, very little light can be transmitted through the sample during the detection process, creating a high absorbance reading. If there is little to no drug in the sample, the enzyme labeled antigens will bind with the antibodies and the enzyme’s activity will be reduced or deactivated leading to a lower absorbance response. Overall, the concentration of the drug would be directly proportional to the absorbance reading. Fluorescence Polarization Immunoassay (FPIA) is also a homogenous, competitive immunoassay technique. The label generally used for this technique is a fluorophore, which can absorb light at 485 nm and emit light in the range of 525-550 nm. In this instrument, filters are used to convert light into polarized light, traveling in one plane. These filters are placed before and after the sample detection area in an effort to maintain the polarized light plane from absorption through emission. Overall, the resulting instrumental response or polarization signal is dependent on whether or not the fluorophore labeled antigen is bound or unbound and the resulting orientation of emitted light. Unbound fluorophore labeled antigens are smaller molecules and will freely rotate in solution. Polarized light impinging on these rotating molecules will become depolarized and the emitted light will be partially or completely blocked from passing through the second filter, decreasing the polarization signal. When fluorophore labeled antigens are bound to antibodies, their molecular size becomes much larger and their rotation in solution decreases. When polarized light impinges on these larger, more static molecules, the plane is maintained from absorption through emission. The plane-polarized light can then pass through the second polarizing filter, increasing the polarization signal. With this technique, the concentration of drug in the sample is inversely proportional to the instrument’s response. Kinetic Interaction of Microparticles in Solution (KIMS)- KIMS is also a competitive immunoassay technique that involves a precipitation process. In this instance, the label addition creates an antigen-microparticle conjugate, which will compete with the sample’s antigens for the available antibodies. If the microparticle labeled antigens bind to antibodies (low drug concentration), a cross-linked molecular lattice (agglutinate) is formed capable of blocking transmitted light, thereby increasing the absorbance reading. If the competing sample’s antigens attach instead to the antibodies, the degree to which the lattice will form decreases and the absorbance reading will decrease. Overall, the instrument’s absorbance response will be inversely proportional to the amount of drug or sample’s antigens present. Cloned Enzyme Donor Immunoassay (CEDIA) is also a homogenous, competitive technique. In this instance the label is an enzyme, which is initially in two pieces when added to the solution. Detection is based on the recombination of these two inactive protein fragments to form a fully functional enzyme capable of reacting with a substrate. The larger of the two fragments is referred to as the enzyme acceptor (EA) while the smaller fragment is termed the enzyme donor (ED). The antigen in this technique is attached to the ED fragment. The solution in this immunoassay consists of the ED labeled antigens, competing sample’s antigens and antibodies. The EA is also added to the mixture in addition to a substrate for detection. If the ED labeled antigens bind with antibodies (low drug concentration), then the ED will not be available to recombine with EA. If there are unbound ED labeled antigens in the solution (high drug concentration), the enzyme fragments can recombine and become active. In this instance, the fully functional enzyme will react with the substrate creating a color change, from yellow orange to deep red, for example, which results in a subsequent absorbance change. The amount of drug present in the sample is directly proportional to the instrumental response. The following video illustrate the working mechanism of each assay discussed above. Comparison of Immunoassay Techniques Immunoassay Techniques RIA ELISA Heterogeneous X X EMIT CEDIA FPIA KIMS Homogenous X X Substrate Examples NA 3,3',5,5'Tetramethylbenzidine (TMB) Label Examples Iodine 25 Horseradish Peroxidase Glucose-6phosphate dehydrogenase Betagalactosidase Directly (Noncompetitive) Inversely (Competitive) Directly Directly Instrument Inversely Response Directly or Inversely Proportional to Sample Concentration? Glucose-6- Chlorophenol red‐ phosphate β‐D‐ (G6P) & galactopyranoside Nicotinamide (CPRG) adenine dinucleotide (NAD+) X X NA NA Fluorescein Microparticles Inversely Inversely Reflection: 1. List three (3) types of labels used during immunoassay techniques. 2. Describe the assay components of CEDIA. 3. How does ELISA differ from EMIT? 4. What is common to CEDIA, FPIA, and EMIT that is not common to RIA? 5. Describe why the instrumental response of Competitive ELISA is inversely proportional to the amount of drug in the sample. 6. List 5 biological samples that are suitable for immunoassay analysis. Module 3: Immunoassays Quality Control Page 4 Quality Control Calibrators and Control Solutions In general, most everything needed to conduct these immunoassay techniques is provided in a prepackaged kit. These kits may contain calibrators and control solutions that are in the same matrix (urine or plasma) as the samples to be tested. Controls and calibrators may also be prepared by the forensic toxicology laboratory, but should also be prepared using the same matrix as the sample, unless method validation has demonstrated the absence of matrix effects. Urine may be diluted to 50% to prepare calibrators for urine assay.Controls are prepared in drug free blood or urine. If the assay is a screening assay then a single calibrator is sufficient. For quantitative tests, multiple calibrators with concentrations that bracket those of the samples should be used. There should be enough control solutions run with samples in each assay to demonstrate discrimination above and below cut off for screening tests. In quantitative tests, the controls should show good assay performance in the low and high concentration of the linear range. Before applying an immunoassay technique in the lab, the lab should establish and verify a limit of detection, and linear range for the method. Cut-Off Levels During analysis, a control prepared at an established cutoff level should be included. This cutoff level, or decision point, is the concentration used to distinguish between positive and negative results when screening drugs with immunoassays. The cutoff used may be an established level provided by the kit’s manufacturer. The cutoff might also be an administrative level chosen by the laboratory following method validation to ensure the level used is at or above the limit of detection while also demonstrating good precision. Running control solutions to demonstrate discrimination above and below the cutoff is also a good recommendation. Applications Immunoassays have applications in postmortem investigations, workplace drug testing, and human performance investigation. Urine is usually the matrix of choice because it has fewer interfering compounds and decomposition products. Hair analysis and oral fluid applications are gaining momentum with immunoassay testing, in particular ELISA, as this heterogeneous assay provides an avenue that alleviates matrix effects while provided great sensitivity. Reflection: 1. Define: Cross-reactivity Cutoff levels 2. Why is urine usually the matrix of choice for immunoassay? 4. What two parameters may be used to determine drug "cutoff" levels? Strengths and Weaknesses of Immunoassay Techniques Module 3: Immunoassays Page 5 Strengths and Weaknesses of Immunoassay Techniques Immunoassay + Strengths - Weaknesses RIA Sensitive; good precision; less prone to matrix effects; less susceptible to adulteration Radiation exposure and waste disposal; limited shelf life; expensive to automate, availability of assays EMIT Homogenous; uses a label to increase sensitivity; easily automated; longer shelf life; greater dynamic range; less expensive; less susceptible to adulteration Matrix effects; potential for false negative and positive results; not well suited for matrices other than urine (e.g. blood requires protein precipitation) Single source kit provider, more expensive; potential for false positive results; not well suited for matrices other than urine (e.g. blood require protein precipitation); fluorescent salts in bile may yield false positive results, for example FPIA Homogeneous; uses a stable fluorophore label to increase sensitivity; less matrix effect; long shelf life CEDIA Homogeneous; longer shelf life; linear over wide range of concentrations; more than one wavelength measured for greater specificity; uses a label which increases sensitivity Can be affected by adulterants. KIMS Homogenous; long shelf life; uses stable microparticle label to increase sensitivity Increased maintenance; small linear range ELISA Less prone to matrix effects; easily automated; longer shelf life; uses a label to increase sensitivity; large variety of kit providers and target analytes More expensive; cumbersome assay run set-up (even when automated); can be affected by urine preservative, sodium azide Reflection: 1. List the advantages of ELISA. 2. List the weaknesses of EMIT. 3. What is the disadvantage of a heterogeneous assay? 4. List three homogenous immunoassays. Module 3 Quiz This concludes Module 3. Please click on the Module 3 Quiz link to take quiz. Module 4: Spectrophotometry in Forensic Chemistry Page 1 Module Overview Introduction Welcome to module 4. This module will introduce the concepts and principles of spectrophotometry in forensic chemistry. Topics for the next two weeks will include an introduction to UVvisible, infrared, and fluorescence spectrophotometry. The underlying scientific principles behind the techniques covered in this module can also be applied to the detectors commonly used in high performance chromatography (HPLC), which will be discussed in the next module. Objectives At the end of this module, students should: Understand the principles involved in ultraviolet, visible, fluorescence and infrared spectrophotometry Have a basic knowledge of the electromagnetic spectrum Understand and apply the Beer-Lambert law Understand the role of a chromophore in molecular absorption of electromagnetic radiation Be able to define the term auxochrome Be able to define the terms molecular absorptivity and specific absorbance Understand solvent effects on molecular absorbance Understand the effect of molecular structure and chemical environment on fluorescence Understand the different types of infrared absorbance bands Be able to describe common sample preparation techniques for infrared spectrophotometry Be able to describe the key components of spectrophotometers Understand the advantages and disadvantages of spectral techniques Module 4: Spectrophotometry in Forensic Chemistry Page 2 UV-Visible Spectrophotometry UV-Visible Spectrophotometry Ultraviolet (UV) and visible absorption spectrophotometry are used in pharmaceutical and biomedical analysis for the quantitation and characterization of drugs, diluents, metabolites and other related substances. This technique works on the principle that substances absorb or emit electromagnetic radiation at different wavelengths. The energy absorption or molecular absorption of a substance can be recorded to provide an absorbance spectrum and used to determine the concentration of a substance (or drug) in a solution. Electromagnetic Radiation Electromagnetic radiation is characterized by two physical quantities: a wavelength (λ) and a frequency (v) that are related to the velocity of light (c) by the equation λv = c. The velocity of light in a vacuum is constant at 2.99792 x 108 m/s. Electromagnetic radiation can be thought of as a stream of energy particles or packets of energy known as photons. The energy of the photon is proportional to the frequency of the radiation. Electromagnetic radiation travels as a wave and when it impinges on matter, interactions occur which affect the wave and the material. Some materials absorb the energy causing its molecules to oscillate, inducing electric and magnetic fields that in turn also generate an electromagnetic wave or light, which is emitted by the material. Changes in the electromagnetic radiation can be detected and used to generate absorption and emission spectra for a substance. Various wavelengths and their interactions with atoms and molecules make up the Electromagnetic spectrum. The Electromagnetic Spectrum Radiation Ultraviolet Frequency Wavelength (Hz) 1015-1017 200 - 400 nm Transition Outer electron Visible Near infrared 4-7.5 x 1014 400 - 800 nm 1012 -1014 1