fMRI Summary Task 2 (PDF)
Document Details
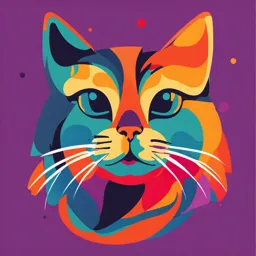
Uploaded by EnergeticStanza9637
Maastricht University
Tags
Summary
This document summarizes the concepts behind T1 and T2 weighted images in fMRI, focusing on the principles of repetition time (TR) and echo time (TE), and explaining spin echo sequences. It also discusses slice selection techniques.
Full Transcript
Task 2: The physicist in you 4. The patient emits a signal, which is received and used for … To distinguish different tissues, we use different pulse sequences. By varying the sequence of RF pulses applied & collected, different types of images can be created. The image is either...
Task 2: The physicist in you 4. The patient emits a signal, which is received and used for … To distinguish different tissues, we use different pulse sequences. By varying the sequence of RF pulses applied & collected, different types of images can be created. The image is either determined by T1 or by T2(*) Repetition Time (TR) is the amount of time between successive RF pulse sequences applied to the same slice. Time to Echo (TE) is the time between the delivery of the RF pulse and the receipt of the echo signal T1-weighted image is determined by Time to Repetition (TR): In the figure above are two tissues with different relaxation times (A = red = solid tissue like fat, and B = green = fluid). We now send a 90 RF pulse, switch it off an wait a certain time until we send another 90 RF pulse (this time is TR). → If TR is long (left graph): tissue A and B have regained all of their longitudinal magnetization by the time of the second pulse and the transversal magnetization after the second pulse will be the same for both tissues, thus A cannot be differentiated from B (would look the same in an image). → If TR is short (right graph): the longitudinal magnetization of tissue A has regained more than that of tissue B. The second pulse tilts the vector again by 90 degrees, and the transversal magnetic vector of tissue A is larger than that of tissue B. The larger vector of A reaches closer to the antenna causing a stronger signal than the signal of the vector of B. Thus A and B can be differentiated. 5 T2-weighted image is determined by the Time to Echo (TE) Spin Echo Sequences: consists of one 90RF pulse and at least one 180 pulse We start with again with a 90-degree pulse, after the pulse is turned off, the longitudinal magnetization increases again and the protons dephase Then, after a specific time (TE/2, half of TE) we add a 180-degree pulse This pulse makes the protons turn around, so that they precess in the exact opposite direction: Faster processing protons are now behind the slower ones If we wait another time TE/2, the faster ones will have caught up with the slower ones: at this point in time the protons are nearly in phase again, which results in in a stronger transversal magnetization, and thus in a stronger signal, which is called the Spin Echo The strength of the spin echo depends on the T2 properties of tissue: Protons in fluid dephase slower than protons in fatty tissue and thus result in a stronger signal (here white in the image) → If TE is short: the shorter the TE, the stronger is the spin echo signal (as it decreases with time). However, the difference in tissue A and B is not big enough yet to differentiate them from another, thus they look the same in a picture → If TE is long: We need to wait long enough to get a sufficient contrast between the two tissues. However, at that moment the overall signal already decreased and is thus weaker, resulting in a bad signal-to-noise ratio Short TR short TE = T1 weighted, long TR long TE = T2 weighted, short TR long TE = no image We can apply a 180-degree pulse again and again, but the overall resulting signal decreases with time: This is because our 180-degree pulse neutralizes the effect of constant inhomogeneities of the external magnetic field and inconstant inhomogeneities cannot be evened out, as they may influence some protons differently before and after the 180 pulse Thus, some of the protons may still be behind or in front of the majority of the protons that will be in phase at the same time Therefore, from echo to echo, the intensity of the signal goes down due to T2-effects, the curve is called a T2-curve. If there was no 180 degree pulse then the signal would decay much faster and the curve was a T2*-curve T2(*)-Curve: Pulse Sequence: 6 5. … Reconstruction of the picture This picture simply shows the free induction decay: we only detect the images at the transversal plane, the magnetization has to be on the axis of the coil. Slice selection: We can select a slice to be examined by using a gradient field (RF pulse that has a range of frequencies), which is superimposed on the external magnetic field. Protons along this gradient field are exposed to different magnetic field strengths, and thus have different precession frequencies. As they have different precession frequencies, we can send in an RF pulse that contains only those frequencies, which excite the protons in the slice which we want to image. Slice thickness can be altered in two ways: (1) by changing the band width of the RF pulse: the wider the range of frequencies of the gradient field, the thicker the slices (2) by modifying the steepness of the gradient field: If we have a steeper gradient field, i.e., one that has more difference in field strength over a specific distance, the precession frequencies will also vary to a larger degree. → The desired slice thickness depends on the wanted resolution, amount of brain you want to cover and possible duration of the scan → If the slice is too thin, the signal is too weak as there are not enough protons, by increasing the tesla you can use thinner slices, that’s why they have higher resolution Where does the signal come from? Now we have selected position and thickness of our slice. But how can we find out, from what point of our slice a certain signal is coming from - some information that we must have to construct a picture? → We use two other gradients, the frequency encoding, and the phase encoding gradient → Frequency encoding gradient is sent in after the slice selection gradient. It is applied in the direction of the y-axis. This results in different precession frequencies along the y-axis, and thus different frequencies of the corresponding signals (columns) → Phase encoding gradient is turned on for a short time after the RF pulse along the x-axis. During this short time, the protons along the x-axis precess with different frequencies. When this gradient is switched off, they go back to their former precession frequency, which was the same for all of them. (Rows) however, the protons and their signals are now out of phase, which can be detected. (Note: which gradient is applied in what direction (y-axis, x-axis) can be varied!). 7 By means of the Fourier transformation, a computer can analyze the mixture of signals that come out of a slice, and determine the intensity of the components that either have different frequencies or different phase: There is no one to one translation of encoding the voxel, you always have to do it for the whole image to get all the different spatial frequencies → Now, it is known where in a given slice a signal with a certain frequency or certain phase comes from. And as the Fourier transformation gave us the corresponding signal intensities, we can now assign a certain signal intensity to a specific location, which results in our MR picture 8