Final Materials PDF
Document Details
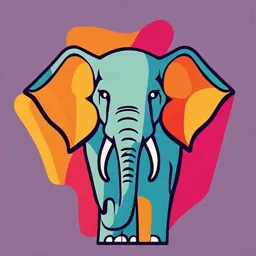
Uploaded by StrongerObsidian4943
Birzeit University
2017
Tags
Summary
This document provides a summary of Biochemistry, covering topics like peptides, proteins, and enzyme function.
Full Transcript
1 | The Foundations of Biochemistry 2 | Water © 2017 W. H. Freeman and Company Living vs. Non living matter Elements Human body Earth’s Crust Oxygen 65% 49% Carbon 18% 1% Hydrogen 10% 1% Nitrogen 3% Trace Calcium...
1 | The Foundations of Biochemistry 2 | Water © 2017 W. H. Freeman and Company Living vs. Non living matter Elements Human body Earth’s Crust Oxygen 65% 49% Carbon 18% 1% Hydrogen 10% 1% Nitrogen 3% Trace Calcium 2% 3% Iron pI = net negative change pH < pI = net positive charge Clicker Question 5, Response Which statement is correct about peptides? B. Peptides have their amino acid sequences written from the N-terminus. Peptides are named beginning with the amino- terminal (N-terminal) residue. Peptides: A Variety of Functions Hormones and pheromones: – insulin (sugar uptake) – oxytocin (childbirth) – sex-peptide (fruit fly mating) Neuropeptides – substance P (pain mediator) Antibiotics: – polymyxin B (for Gram - bacteria) – bacitracin (for Gram + bacteria) Protection, e.g. toxins – amanitin (mushrooms) – conotoxin (cone snails) – chlorotoxin (scorpions) Estimating the Number of Amino Acid Residues number of residues = molecular weight/110 average molecular weight of amino acid = ~128 molecule of water removed to form peptide bond = 18 128 – 18 = 110 Some Proteins Contain Chemical Groups Other Than Amino Acids conjugated proteins = Table 3-4 Conjugated Proteins Class Prosthetic group Example contain permanently Lipoproteins Lipids β1-Lipoprotein of blood associated chemical (Fig. 17-2) components Glycoproteins Carbohydrates Immunoglobulin G – non–amino acid part (Fig. 5-20) Phosphoproteins Phosphate groups Glycogen phosphorylase (Fig. 6-39) = prosthetic group Hemoproteins Heme (iron porphyrin) Hemoglobin (Figs 5-8 to 5-11) lipoproteins contain lipids Flavoproteins Flavin nucleotides Succinate dehydrogenase (Fig. 19-9) Metalloproteins Iron Ferritin (Box 16-1) glycoproteins contain Zinc Alcohol dehydrogenase (Fig. 14-12) Calmodulin (Fig. 12-17) sugars Calcium Molybdenum Dinitrogenase (Fig. 22-3) Copper Complex IV (Fig. 19-12) metalloproteins contain specific metals Specific Activity Describes the Purity of the Protein of Interest Proteins in a complex mixture often require more than one purification to completely isolate the protein of interest. During purification, determination of the location of the protein of interest can be performed by tracking its behavior. If a protein has a specific function (e.g., binding insulin), the fraction that binds insulin best after each purification step will contain the most of the protein of interest. The function of the protein is called the “activity.” The ratio of activity to total protein concentration is called the “specific activity.” Unseparated Proteins Are Detected and Quantified Based on Their Functions can monitor enzyme purification by assaying specific activity: – activity = total enzyme units in a solution – specific activity = number of enzyme units per mg of total protein 5 red in 100 5 red in 20 Specific Activity 1 unit of enzyme activity: amount of enzyme causing transformation of 1 mol of substrate / min at 25 oC Activity: Total units of enzyme in a solution Specific Activity: number of enzyme units / mg of total protein In a purification, many steps are used After each step, total protein ↓(sometimes activity ↓) but specific activity ↑ Sequential Purification Steps Decrease Sample Size Table 3-5 A Hypothetical Purification Table for an Enzyme Fraction Specific volume Total Activity activity Procedure or step (mL) protein (mg) (units) (units/mg) 1. Crude cellular extract 1,400 10,000 100,000 10 2. Precipitation with ammonium sulfate 280 3,000 96,000 32 3. Ion-exchange chromatography 90 400 80,000 200 4. Size-exclusion chromatography 80 100 60,000 600 5. Affinity chromatography 6 3 45,000 15,000 Note: All data represent the status of the sample after the designated procedure has been carried out. “Activity” and “specific activity” are defined on page 90. final specific activity:starting specific activity ratio = purification factor percentage of the final activity/percentage starting Video: activity = percent yield 1) Protein Purification Clicker Question 13, Response What measurement increases during purification of an enzyme? C. specific activity The specific activity is the number of enzyme units per milligram of total protein. The specific activity is a measure of enzyme purity: it increases during purification of an enzyme and becomes maximal and constant when the enzyme is pure. 3.4 The Structure of Proteins: Primary Structure Levels of Structure in Proteins four levels: – primary structure = covalent bonds linking amino acid residues in a polypeptide chain – secondary structure = recurring structural patterns – tertiary structure = 3D folding of polypeptide – quaternary structure = 2+ polypeptide subunits Video: 1) Protein Structure and Folding Clicker Question 14, Response What is the highest level of protein structure in human insulin, which has two polypeptides of different mass linked by several disulfide bonds? D. quaternary Insulin has two polypeptide subunits. When a protein has two or more polypeptide subunits, their arrangement in space is referred to as quaternary structure. Principle 4 Shaped by evolution, amino acid sequences are a key resource for understanding the function of individual proteins and for tracing broader functional and evolutionary relationships. Studying Protein Structure Using Proteases proteases = catalyze hydrolytic cleavage of peptide bonds Table 3-6 The Specificity of Some Common Methods for Fragmenting Polypeptide Chains Reagent (biological source) Cleavage points Trypsin (bovine pancreas) Lys, Arg (C) Chymotrypsin (bovine pancreas) Phe, Trp, Tyr (C) Staphylococcus aureus V8 protease (bacterium S. aureus) Asp, Glu (C) Asp-N-protease (bacterium Pseudomonas fragi) Asp, Glu (N) Pepsin (porcine stomach) Leu, Phe, Trp, Tyr (N) Endoproteinase Lys C (bacterium lysobacter enzymogenes) Lys (C) Cyanogen bromide Met (C) 4 The Three- Dimensional Structure of Proteins © 2021 Macmillan Learning General Aspects of Protein Structure 1. aa sequence determines 3D structure 2. Protein function depends on its structure 3. An isolated protein usually exists in one or few stable structures 4. Noncovalent interactions are the most important forces stabilizing protein structure 5. There are common structural patterns in protein architecture The Relationship between Protein Structure and Function in principle, proteins can assume an uncountable number of special arrangements, or conformations chemical or structural functions relate to unique three- dimensional structures 4.1 Overview of Protein Structure Principle 1 Protein structures are stabilized by noncovalent interactions and forces. Formation of a thermodynamically favorable structure depends on the influences of the hydrophobic effect, hydrogen bonds, ionic interactions, and van der Waals forces. Natural protein structures are constrained by peptide bonds, whose configurations can be described by the dihedral angles φ and ψ. Protein Conformations limited number of conformations predominate under biological conditions conformations = thermodynamically the most stable, that is, lowest free energy (G) native = proteins in any functional, folded conformations A Protein’s Conformation Is Stabilized Largely by Weak Interactions stability = tendency of a protein to maintain a native conformation unfolded proteins have high conformational entropy chemical interactions stabilize native conformations: – strong disulfide (covalent) bonds are uncommon – weak (noncovalent) interactions and forces are numerous hydrogen bonds hydrophobic effect ionic interactions Packing of Hydrophobic Amino Acids Away from Water Favors Protein Folding hydrophobic effect = predominating weak interaction solvation layer = highly structured shell of H2O around a hydrophobic molecule – decreases when nonpolar groups cluster together – decrease causes a favorable increase in net entropy hydrophobic R chains form a hydrophobic protein core Polar Groups Contribute Hydrogen Bonds and Ion Pairs to Protein Folding repeating secondary structures ( helices and sheets) optimize hydrogen bonding interaction of oppositely charged groups = ion pair = salt bridge – strength increases in an environment of lower dielectric constant, ε – polar aqueous solvent: ε ~ 80 – nonpolar protein interior: ε ~ 4 Individual van der Waals Interactions Are Weak but Combine to Promote Folding van der Waals interactions = dipole-dipole interactions over short distances individual interactions contribute little to overall protein stability high number of interactions can be substantial The Peptide Bond Is Rigid and Planar 3 covalent bonds separate the carbons of adjacent amino acid residues: C —C—N—C resonance between the carbonyl oxygen and the amide nitrogen partial negative charge and partial positive charge sets up a small electric dipole Peptide C—N Bonds Cannot Rotate Freely 6 atoms of the peptide group lie in a single plane partial double-bond character of C—N peptide bond prevents rotation, limiting range of conformations Dihedral Angles Define Peptide Conformations 3 dihedral angles: – φ (phi) = between −180 and +180 degrees – ψ (psi) = between −180 and +180 degrees – ω (omega) = ±180 degrees for trans peptide bonds (0 for the rare cis peptide bonds) φ ψ ω Prohibited Conformations many φ (phi) and ψ (psi) values are prohibited by steric interference – φ and ψ cannot both = 0 degrees – In a fully-extended polypeptide, both φ and ψ are 180 degrees 4.2 Protein Secondary Structure Principle 2 Protein segments can adopt regular secondary structures such as the α helix and the β conformation. These structures are defined by particular values of φ and ψ and their formation is impacted by the amino acid composition of the segment. All of the φ and ψ values for a given protein structure can be visualized using a Ramachandran plot. Protein Secondary Structure secondary structure = describes the local spatial arrangement of the main-chain atoms (backbone) in a segment of a polypeptide chain – regular secondary structure: φ and ψ remain the same throughout the segment – common types: helix, conformation, turn, random coils The Helix Is a Common Protein Secondary Structure helix = simplest arrangement, maximum number of hydrogen bonds – backbone wound around an imaginary longitudinal axis – R groups protrude out from the backbone – each helical turn = 3.6 residues, ∼5.4 Å The Helix: Top View The inner diameter of the helix (no side Helical chains) is about 4–5 Å wheel Too small for anything to fit “inside” The outer diameter of the helix (with side chains) is 10–12 Å Fits well into the major groove of dsDNA Residues 1 and 8 align nicely on top of each other What kind of sequence gives an helix with one hydrophobic face? Amino Acid Sequence Affects Stability of the Helix amino acid residues have an intrinsic propensity to form an helix Helical wheel interactions between R chains spaced 3–4 residues apart can stabilize or destabilize helix – charge, size, and shape of R chains can destabilize – formation of ion pairs and hydrophobic effect can stabilize Proline and Glycine Occur Infrequently in an Helix proline = introduces destabilizing kink in helix – nitrogen atom is part of rigid ring – rotation about N—C bond not possible glycine = high conformational flexibility, take up coiled structures Amino Acid Residues Near the End of the Helix Segment Affect Stability small electric dipoles in each peptide bond align through hydrogen bonds negatively charged amino acids often found near the NH3+ terminus positively charged amino acids often found near the COO– terminus The Conformation Organizes Polypeptide Chains into Sheets conformation = backbone extends into a zigzag – strand = single protein segment – sheet = several strands in conformation side by side Adjacent Polypeptide Chains in a Sheet Can Be Antiparallel or Parallel antiparallel = opposite orientation – occur more frequently parallel = same orientation H bonds form between backbone atoms of adjacent segments Turns Are Common in Proteins turns = connect ends of two adjacent segments of an antiparallel sheet – 180° turn – involves 4 residues – hydrogen bond forms between first and fourth residue – Gly (residue 2) and Pro (residue 3) often occur in turns Protein Tertiary and Quaternary Structure tertiary structure = overall three-dimensional arrangement of all the atoms in a protein – weak interactions and covalent bonds hold interacting segments in position quaternary structure = arrangement of 2+ separate polypeptide chains in three-dimensional complexes Video: 1) What is a Protein? (from PDB-101) Classifying Proteins four major types of protein groups based on polypeptide chains: – fibrous proteins = arranged in long strands or sheets – globular proteins = folded into a spherical or globular shape – membrane proteins = embedded in hydrophobic lipid membranes – intrinsically disordered proteins = lacking stable tertiary structures Fibrous Proteins Are Adapted for a Structural Function give strength and/or flexibility to structures simple repeating element of secondary structure H2O insoluble due to high concentrations of hydrophobic residues Table 4-2 Secondary Structures and Properties of Some Fibrous Proteins Structure Characteristics Examples of occurrence α Helix, cross-linked by Tough, insoluble protective α-Keratin of hair, feathers, nails disulfide bonds structures of varying hardness and flexibility β Conformation Soft, flexible filaments Silk fibroin Collagen triple helix High tensile strength, without Collagen of tendons, bone stretch matrix The Structure of α-Keratin in Hair α-keratin helix is a right-handed α helix two strands of α-keratin, oriented in parallel, wrap about each other to form a supertwisted coiled coil – supertwisted helical path is left-handed Hair Contains Many α-Keratin Filaments rich in hydrophobic residues: Ala, Val, Leu, Ile, Met, Phe cross-links stabilized by disulfide bonds Chemistry of Permanent Waving Video: 1) Perming hair and tetiary structure of keratin The Structure of Collagen collagen = found in connective tissue – secondary structure = left- handed, repeating tripeptide unit Gly–X–Y, where X is often Pro and Y is often 4-Hyp – 3 aa per turn – tertiary and quaternary structure = right-handed twisting of 3 separate polypeptides – Higher tensile strength than a steel wire of equal cross section Some Proteins Undergo Assisted Folding chaperone proteins = facilitate correct folding pathways or ideal microenvironments – Hsp70 = bind to hydrophobic regions – chaperonins = required for the folding of proteins that do not fold spontaneously Defects in Protein Folding Are the Molecular Basis for Many Human Genetic Disorders amyloid fiber = protein secreted in a misfolded state and converted to an insoluble extracellular fiber amyloidosis diseases: type 2 diabetes, Alzheimer disease, Huntington disease, and Parkinson disease Formation of Disease-Causing Amyloid Fibrils native = high degree of β-sheet structure misfolded β amyloid promotes aggregation, forming an amyloid fibril Neurodegenerative Conditions Alzheimer disease = associated with extracellular amyloid deposition by neurons, involving the amyloid-β peptide Parkinson disease = misfolded form α-synuclein aggregates into spherical filamentous masses called Lewy bodies Huntington disease = involves the intracellular aggregation of huntingtin, a protein with long polyglutamine repeat Cystic Fibrosis cystic fibrosis = caused by defects in the membrane- bound protein cystic fibrosis transmembrane conductance regulator (CFTR) – deletion of a Phe residue causes improper protein folding Death by Misfolding: The Prion Diseases prion protein (PrP) = misfolded brain protein Pyramidal cells Comparable in the human section from a cerebral cortex patient with Creutzfeldt-Jakob “mad cow” disease 5 Protein Function © 2021 Macmillan Learning Functions of Globular Proteins Storage of ions and molecules – myoglobin, ferritin Transport of ions and molecules – hemoglobin, glucose transporter Defense against pathogens – antibodies, cytokines Muscle contraction – actin, myosin Biological catalysis – chymotrypsin, lysozyme Proteins Function by Interacting Dynamically with Other Molecules two types of interactions: – protein acting as a reaction catalyst, or enzyme, alters the chemical configuration or composition of a bound molecule – neither the chemical configuration nor the composition of the bound molecule is changed 5.1 Reversible Binding of a Protein to a Ligand: Oxygen- Binding Proteins Principle 1 The functions of many proteins involve the reversible binding of other molecules. A molecule bound reversibly by a protein is called a ligand. A ligand may be any kind of molecule, including another protein. The transient nature of protein-ligand interactions is critical to life, allowing an organism to respond rapidly and reversibly to changing environmental and metabolic circumstances. Oxygen Can Bind to a Heme Prosthetic Group oxygen: – poorly soluble in aqueous solutions – diffusion through tissues is ineffective over large distances – transition metals have strong tendency to bind O2 (iron, copper) – None of the 20 proteinogenic amino acids can directly bind O2 Heme Prosthetic Group heme = protein- bound prosthetic group – present in myoglobin and hemoglobin – consists of a complex organic ring structure, protoporphyrin, with a bound Fe2+ atom Coordination Bonds of Iron six coordination bonds: – four to nitrogen atoms in the flat porphyrin ring – two perpendicular to the porphyrin Clicker Question 1 The heme prosthetic group: A. consists of protoporphyrin and an iron (II) ion. B. is found only in myoglobin and hemoglobin. C. contains a single iron atom in its ferrous (Fe2+) state that has a total of 4 coordination bonds. D. is only found bound to protein. Clicker Question 1, Response The heme prosthetic group: A. consists of protoporphyrin and an iron (II) ion. Heme consists of a complex organic ring structure, protoporphyrin, to which is bound a single iron atom in its ferrous (Fe2+) state. Graphical Representations of Ligand Binding [L] Y= (5-5) [L] + K1 a [L] at which ½ of the available ligand-binding sites are occupied (Y = 0.5) corresponds to 1/Ka Dissociation Constant dissociation constant (Kd) = reciprocal of Ka – equilibrium constant for the release of ligand – lower Kd = higher affinity [P][L] kd when [L] = Kd, ½ Kd = = (5-6) [PL] ka of the ligand- binding sites are occupied [PL] = [P][L] (5-7) Kd Y= [L] [L] + Kd (5-8) Clicker Question 4 Protein A has a Ka = 6.0 μM–1 for binding ligand L, and the protein B has a Kd = 4.0 μM for binding ligand L. Based on this information, which statement is true? A. Protein B binds L with higher affinity. B. Protein A is half-saturated with L when [L] is 6.0 μM–1. C. The Ka of protein B for L is 0.25 μM–1. D. When [L] = 1 μM, Y = 0.17 for protein A. Clicker Question 4, Response Protein A has a Ka = 6.0 μM–1 for binding ligand L, and the protein B has a Kd = 4.0 μM for binding ligand L. Based on this information, which statement is true? C. The Ka of protein B for L is 0.25 μM–1. Ka = 1/Kd For protein B, Ka = 1/(4.0 μM) = 0.25 μM–1. Representative Kd Values Principle 4 The binding of a protein and a ligand is often coupled to a conformational change in the protein that makes the binding site more complementary to the ligand, permitting tighter binding. The structural adaptation that occurs between protein and ligand is called induced fit. Hemoglobin Undergoes a Structural Change on Binding Oxygen two conformations of Greater number hemoglobin: of ion pairs – R state = O2 has a higher affinity for hemoglobin – T state = more stable when O2 is absent, predominant conformation of deoxyhemoglobin Ion Pairs Stabilize the T State T state is stabilized by a greater number of ion pairs, many of which lie at the α1β2 (and α2β1) interface Conformational Change in Hemoglobin O2 binding to hemoglobin in the T state triggers a conformational change to the R state – αβ subunit pairs slide past each other and rotate – the pocket between the β subunits narrow – some ion pairs that stabilize the T state break and some new ones form Hemoglobin Binds Oxygen Cooperatively For effective transport, affinity must vary with pO2 Hemoglobin has a hybrid sigmoid binding curve for oxygen Clicker Question 9, Response Leghemoglobin is an oxygen-binding protein in root nodules that contain bacteria and fix atmospheric nitrogen. Which statement is true if leghemoglobin is like myoglobin and not hemoglobin? B. The oxygen-binding curve is hyperbolic. For a monomeric protein such as myoglobin, the fraction of binding sites occupied by a ligand is a hyperbolic function of ligand concentration. Thus, if leghemoglobin is like myoglobin, the oxygen-binding curve is hyperbolic. Principle 6 Interactions between ligands and proteins may be regulated. Allosteric Proteins allosteric protein (e.g., hemoglobin) = binding of a ligand to one site affects the binding properties of another site on the same protein – modulators = ligands that bind to an allosteric protein to induce a conformational change – homotropic = normal ligand and modulator are identical – heterotropic = modulator is a molecule other than the normal ligand Clicker Question 10 Which molecule is a homotropic modulator of oxygen binding to hemoglobin? A. oxygen B. H+ C. carbon dioxide D. hemoglobin E. carbon monoxide Clicker Question 10, Response Which molecule is a homotropic modulator of oxygen binding to hemoglobin? A. oxygen Cooperative binding of oxygen to hemoglobin, a multimeric protein, is a form of allosteric binding. The binding of one ligand affects the affinities of any remaining unfilled binding sites. Oxygen can be considered as both a ligand and an activating homotropic modulator. Carbon Monoxide Can Bind to Hemoglobin CO has ~250-fold greater affinity for hemoglobin than does O2 The tight binding of CO to hemoglobin means that COHb can accumulate over time as people are exposed to a constant low-level source of CO. Cooperative Ligand Binding Can Be Described Quantitatively for a protein with n binding sites, the equilibrium becomes: P + nL ⇌ PLn (5-12) The Ka and Y for Cooperative Ligand Binding the expression for the association constant becomes: [PLn] Ka = (5-13) [P][L]n the expression for Y is: [L]n Y= n+ (5-14) [L] Kd The Hill Equation [L]n Y= n+ (5-14) [L] Kd rearranging, then taking the log of both sides, yields: Y = [L]n (5-15) 1 −Y Kd the Hill equation: Y log = n log [L] – log K d (5-16) 1 −Y Hill Plots and Hill Coefficients Hill plot = plot of log [Y/(1 −Y)] versus log [L] Hill coefficient = nH = slope of a Hill plot – If nH = 1, ligand binding is not cooperative – nH > 1 indicates positive cooperativity – nH < 1 indicates negative cooperativity Clicker Question 11 A newly discovered protein has multiple subunits, each with a single ligand-binding site. Binding of ligand to one site increases the binding affinity of other sites for the ligand. The Hill coefficient (nH) is: A. equal to 1. B. greater than 1. C. less than 1. D. not able to be calculated. Clicker Question 11, Response A newly discovered protein has multiple subunits, each with a single ligand-binding site. Binding of ligand to one site increases the binding affinity of other sites for the ligand. The Hill coefficient (nH) is: B. greater than 1. The Hill coefficient (nH) is the slope of a Hill plot. An nH of greater than 1 indicates positive cooperativity in ligand binding. This is the situation observed in the newly discovered protein, in which the binding of one molecule of ligand facilitates the binding of others. Adapting the Hill Equation to the Binding of O2 to Hemoglobin n substituting pO2 for [L] and P 50 for Kd: Y log = n log pO2 – n log P50 (5-17) 1 −Y Hill coefficient = nH = slope of a Hill plot – If nH = 1, ligand binding is not cooperative – nH > 1 indicates positive cooperativity – nH < 1 indicates negative cooperativity Hill Plots for Myoglobin and Hemoglobin Hemoglobin Also Transports H+ and CO2 hemoglobin carries two end products of cellular respiration: H+ and CO2 carbonic anhydrase (mainly in RBCs) catalyzes the hydration of CO2 to bicarbonate: CO2 + H2O ⇌ H+ + HCO3– The Bohr Effect the structural effects of H+ and CO2 binding to hemoglobin favor the T state – the binding of H+ and CO2 is inversely related to the binding of O2 Bohr effect = describes the effect of pH and [CO2] on the binding and release of O2 by hemoglobin HHb+ + O2 ⇌ HbO2 + H+ A major contribution to the Bohr effect is made by (His HC3) of the β subunits. When protonated, this residue forms one of the ion pairs — to (Asp FG1) — that helps stabilize deoxyhemoglobin in The Antibody-Antigen Interaction Is the Basis for a Variety of Important Analytical Procedures two types of antibody preparations are used: – polyclonal antibodies produced by injecting a protein into an animal contain a mixture of antibodies that recognize different parts of the protein – monoclonal antibodies Synthesized by a population of identical B cells (a clone) homogeneous, all recognizing the same epitope Clicker Question 21 Polyclonal antibodies: A. are synthesized by a population of identical B cells. B. all recognize the same epitope. C. are identical to monoclonal antibodies. D. are used as analytic reagents in Western blot assays. Clicker Question 21, Response Polyclonal antibodies: D. are used as analytic reagents in Western blot assays. Polyclonal antibodies are used in Western blot assays. The specific reaction of the antibody with its antigen makes it possible to identify and quantify a specific protein in a complex sample. 6 Enzymes © 2021 Macmillan Learning Catalysis Think of sugar (C12H22O11): Our bodies use it to give energy within seconds (converting it to CO2 + H2O) But a bag of sugar can be on the shelve for years even though the process is very thermodynamically favorable The difference is catalysis 6.1 An Introduction to Enzymes Enzymes in 1897, Eduard Buchner: – demonstrated cell-free yeast extracts could ferment sugar to alcohol – showed fermentation was promoted by molecules that continued to function when removed from cells the name enzymes (meaning: “in yeast”) was given to the molecules detected by Buchner Principle 1 Enzymes are powerful biological catalysts. Rate accelerations by enzymes are often far greater than those by synthetic or inorganic catalysts. Like all catalysts, enzymes increase reaction rates, lowering reaction activation barriers. Enzymes do not affect the equilibria of reactions. Most Enzymes Are Proteins catalytic activity depends on the integrity of the native protein conformation molecular weight = ranges from 12,000 to >1 million some enzymes require additional chemical components: – cofactor = 1+ inorganic ions, such as Fe2+, Mg2+, Mn2+, or Zn2+ – coenzyme = complex organic or metalloorganic molecules that act as transient carriers of specific functional groups Inorganic Ions as Cofactors Table 6-1 Some Inorganic Ions That Serve as Cofactors for Enzymes Ions Enzymes Cu2+ Cytochrome oxidase Fe2+ or Fe3+ Cytochrome oxidase, catalase, peroxidase K+ Pyruvate kinase Mg2+ Hexokinase, glucose 6-phosphatase, pyruvate kinase Mn2+ Arginase, ribonucleotide reductase Mo Dinitrogenase Ni2+ Urease Zn2+ Carbonic anhydrase, alcohol dehydrogenase, carboxypeptidases A and B Coenzymes as Transient Carriers of Atoms or Functional Groups Table 6-2 Some Coenzymes That Serve as Transient Carriers of Specific Atoms or Functional Groups Examples of chemical Dietary precursor in Coenzyme groups transferred mammals Biocytin CO2 Biotin (vitamin B7) Coenzyme A Acyl groups Pantothenic acid (vitamin B5) and other compounds 5′-Deoxyadenosylcobalamin (coenzyme B12) H atoms and alkyl groups Vitamin B12 Flavin adenine dinucleotide Electrons Riboflavin (vitamin B2) Lipoate Electrons and acyl groups Not required in diet Nicotinamide adenine dinucleotide Hydride ion (:H–) Nicotinic acid (niacin, vitamin B3) Pyridoxal phosphate Amino groups Pyridoxine (vitamin B6) Tetrahydrofolate One-carbon groups Folate (vitamin B9) Thiamine pyrophosphate Aldehydes Thiamine (vitamin B1) Describing Enzymes and Their Additional Chemical Components prosthetic group = coenzyme or metal ion that is very tightly or covalently bound to the enzyme protein holoenzyme = complete catalytically active enzyme together with its bound coenzyme and/or metal ions apoenzyme or apoprotein = the protein part of a holoenzyme Videos: 1) Cofactors | Coenzymes | Holoenzyme | Apoenzyme 2) Coenzymes, Cofactors & Prosthetic Groups Function and Interactions Clicker Question 3 An apoenzyme: A. is the nonprotein component of a holoenzyme. B. always requires an inorganic ion for its activity. C. requires a cofactor for its activity. D. is an enzyme consisting of RNA rather than protein. Clicker Question 3, Response An apoenzyme: C. requires a cofactor for its activity. A coenzyme or metal ion that is very tightly or even covalently bound to the enzyme protein is called a prosthetic group. A complete, catalytically active enzyme together with its bound coenzyme and/or metal ions is called a holoenzyme. The protein part of such an enzyme is called the apoenzyme or apoprotein. An apoenzyme requires a cofactor for its activity. Clicker Question 4 Some enzymes require an inorganic ion for catalytic function. When this inorganic ion is very tightly or covalently bound by the enzyme it is called a(n): A. apoenzyme. B. prosthetic group. C. holoenzyme. D. catalyst. Clicker Question 4, Response Some enzymes require an inorganic ion for catalytic function. When this inorganic ion is very tightly or covalently bound by the enzyme it is called a(n): B. prosthetic group. A coenzyme or metal ion that is very tightly or even covalently bound to the enzyme protein is called a prosthetic group. Enzymes Are Classified by the Reactions They Catalyze enzymes are divided into seven classes, each with subclasses, based on the type of reaction catalyzed each enzyme has a four-part Enzyme Commission number (E.C. number) and a systematic name most enzymes have trivial names Table 6-3 International Classification of Enzymes Class number Class name Type of reaction catalyzed 1 Oxidoreductases Transfer of electrons (hydride ions or H atoms) 2 Transferases Group transfer 3 Hydrolases Hydrolysis (transfer of functional groups to water) 4 Lyases Cleavage of C—C, C—O, C—N, or other bonds by elimination, leaving double bonds or rings, or addition of groups to double bonds 5 Isomerases Transfer of groups within molecules to yield isomeric forms 6 Ligases Formation of C—C, C—S, C—O, and C—N bonds by condensation reactions coupled to cleavage of ATP or similar cofactor 7 Translocases Movement of molecules or ions across membranes or their separation within membranes Example: ATP + D-glucose → ADP + D-glucose 6-phosphate Formal name: ATP:glucose phosphotransferase Common name: hexokinase E.C. number: 2.7.1.1 2 → class name (transferases) 7 → subclass (phosphotransferases) 1 → phosphotransferase with –OH as acceptor 1 → D-glucose is the phosphoryl group acceptor Clicker Question 5 is NOT an E.C. class name for enzymes. A. Transferases B. Polymerases C. Lyases D. Isomerases E. Translocases Clicker Question 5, Response is NOT an E.C. class name for enzymes. B. Polymerases Polymerases is not one of the 7 E.C. classes of enzymes. 6.2 How Enzymes Work Principle 3 Enzymatic reactions occur in specialized pockets called active sites. These pockets are similar to ligand binding sites, except that a reaction occurs there—the conversion of a substrate, a molecule that is acted on by an enzyme, to a product. Video: 1) How Enzymes Work (from PDB-101) Enzyme-Catalyzed Reactions Take Place within the Active Site active site = provides a specific environment in which a given reaction can occur more rapidly substrate = the molecule that is bound to the active site and acted upon by the enzyme Enzymes Affect Reaction Rates, Not Equilibria simple enzymatic reactions can be written as E + S ⇌ ES ⇌ EP ⇌ E + P (6-1) where E, S, and P represent the enzyme, substrate, and product and ES and EP are transient complexes of the enzyme Ground State and Transition State ground state = starting point for either the forward or reverse reaction transition state (‡) = the point at which decay to substrate or product are equally likely Energy Changes in a Reaction Coordinate Diagram biochemical standard free- energy change = ∆G′° = the standard free-energy change at pH 7.0 activation energy (∆G‡) = difference between the ground state energy level and the transition state energy level Clicker Question 6 What is the free-energy starting point for a reverse reaction designated as? A. transition state (‡) B. ground state C. biochemical standard free energy D. activation energy (∆G‡) Clicker Question 6, Response What is the free-energy starting point for a reverse reaction designated as? B. ground state The starting point for either the forward reaction or the reverse reaction is called the ground state, the contribution to the free energy of the system by an average molecule (S or P) under a given set of conditions. Catalysts Lower the Activation Energy and Increase the Reaction Rate Catalysts Do Not Affect Reaction Equilibria E + S ⇌ ES ⇌ EP ⇌ E + P any enzyme that catalyzes the reaction S → P also catalyzes the reaction P → S enzymes accelerate the interconversion of S and P enzymes are not used up in the process the equilibrium point is unaffected Reaction Intermediates reaction intermediate = any species on the reaction pathway that has a finite chemical lifetime – example: ES and EP complexes Rate-Limiting Steps rate-limiting step = the step in a reaction with the highest activation energy that determines the overall rate of the reaction activation energies are barriers to chemical reactions Clicker Question 7 The conversion of sucrose to CO2 and water has a very large and negative ∆G′°.Why is the conversion NOT spontaneous? A. Only some reactions have a negative ∆G′°. B. Conversion requires heat and O2. C. Conversion requires the presence of cofactors. D. There is a very large activation energy barrier. Clicker Question 7, Response The conversion of sucrose to CO2 and water has a very large and negative ∆G′°.Why is the conversion NOT spontaneous? D. There is a very large activation energy barrier. The difference between the ground state energy level and the transition state energy level is the activation energy (∆G‡). The rate of a reaction reflects this activation energy: a higher activation energy corresponds to a slower reaction. Activation energies are energy barriers to chemical reactions. Without such energy barriers, sucrose would spontaneously form CO2 and water. Principle 2 Enzymes exhibit a very high degree of specificity. Each enzyme catalyzes only one chemical reaction, or sometimes a few closely related reactions. Reaction activation barriers are thus lowered selectively. Enzymes Lower Activation Energies Selectively Throughout evolution enzymes have developed to lower activation energies selectively to increase rates for reactions needed for cell survival Clicker Question 8 What does an enzyme change relative to an uncatalyzed reaction? A. the equilibrium constant B. the rate of the reaction C. the pH D. the free energy change of the reaction Clicker Question 8, Response What does an enzyme change relative to an uncatalyzed reaction? B. the rate of the reaction The role of enzymes is to accelerate the interconversion of S and P. The enzyme is not used up in the process, and the equilibrium point is unaffected. However, the reaction reaches equilibrium much faster when the appropriate enzyme is present because the rate of the reaction is increased. Reaction Rates and Equilibria Have Precise Thermodynamic Definitions reaction equilibria are linked to the standard free-energy change for the reaction, ∆G′° reaction rates are linked to the activation energy, ∆G‡ Thermodynamics Relates Keq and ∆G ° equilibrium constant, Keq = describes an equilibrium such as S ⇌ P under standard conditions: = [P] (6-2) K′eq [S] from thermodynamics: ∆G′° = −RT ln K′eq (6-3) A large negative ∆G′° ➔ a favorable reaction eq. (much more P than S at eq.) Does not mean the reaction will take place at a rapid rate The Relationship between K eq and ∆G ° Table 6-4 Relationship between K′eq and ∆G′° (∆G′° = –RT ln K′eq) K′eq ∆G′° (kJ/mol) 10–6 34.2 10–5 28.5 10–4 22.8 10–3 17.1 10–2 11.4 10–1 5.7 1 0.0 101 –5.7 102 –11.4 103 –17.1 Clicker Question 9 Which statement is true regarding the relationship between biochemical standard free-energy change and the equilibrium constant Keq? ∆G′° = –RT ln K′eq A. The relationship between free-energy change and equilibrium is exponential. B. A decrease in Keq translates into a more negative free energy. C. The higher the temperature, the less the reaction favors product formation. D. Small changes in free energy can lead to large changes in equilibria. Clicker Question 9, Response Which statement is true regarding the relationship between biochemical standard free-energy change and the equilibrium constant Keq? ∆G′° = –RT ln K′eq D. Small changes in free energy can lead to large changes in equilibria. According to the equation relating K′eq to ∆G′° for the overall reaction, small changes in ∆G′° can lead to large changes in K′eq. Rate Constants and Rate Equations the rate of any reaction is determined by the concentration of reactant(s) and the rate constant, k for the unimolar reaction S → P, a rate equation expresses the rate of the reaction V = k[S] (6-4) Where: V is the velocity or rate of the reaction (units of concentration*time–1) and [S] is the concentration of the substrate First-Order Reactions first-order reaction = rate depends only on the concentration of S k has units of reciprocal time, such as s–1 Clicker Question 10 If a first-order reaction for the unimolar reaction S → P has a rate constant k of 0.05 s–1, how is this interpreted qualitatively? A. 0.05% of the available S will be converted to P in 1 second. B. 5% of the available S will be converted to P in 1 second. C. 0.05% of the available P will be converted to S in 1 second. D. 5% of the available P will be converted to S in 1 second. Clicker Question 10, Response If a first-order reaction has a rate constant k of 0.05 s–1, how is this interpreted qualitatively? B. 5% of the available S will be converted to P in 1 second. The factor k is a proportionality constant that reflects the probability of reaction under a given set of conditions. For a first-order reaction, the rate constant k has units of reciprocal time. If a first-order reaction has a rate constant k of 0.05 s–1, this may be interpreted qualitatively to mean that 5% of the available S will be converted to P in 1 second. Second-Order Reactions second-order reaction = rate depends on the concentration of two different compounds or the reaction is between two molecules of the same compound k has units of M–1s–1 the rate equation becomes V = k[S1][S2] (6-5) Clicker Question 11 A reaction where the rate depends on the concentration of two molecules is called a: A. first-order reaction. B. second-order reaction. C. third-order reaction. D. fourth-order reaction. Clicker Question 11, Response A reaction where the rate depends on the concentration of two molecules is called a: B. second-order reaction. If a reaction rate depends on the concentration of two different compounds, or if the reaction is between two molecules of the same compound, the reaction is second order. The Relationship between Rate Constants and Activation Energy from transition-state theory k = kT e–∆G‡/RT (6-6) h where k is the Boltzmann constant and h is Planck’s constant the relationship between the rate constant k and the activation energy ∆G‡ is inverse and exponential a lower activation energy means a faster reaction rate A Few Principles Explain the Catalytic Power and Specificity of Enzymes enzymes enhance rates in the range of 5 to 17 orders of magnitude Table 6-5 Some Rate Enhancements Produced by Enzymes Cyclophilin 105 Carbonic anhydrase 107 Triose phosphate isomerase 109 Carboxypeptidase A 1011 Phosphoglucomutase 1012 Succinyl-CoA transferase 1013 Urease 1014 Orotidine monophosphate decarboxylase 1017* *Uncatalyzed rxn has a half-life of 78 million YEARS! The catalyzed rxn occurs in milliseconds Principle 4 Two concepts explain the catalytic power of enzymes. First, enzymes bind most tightly to the transition state of the catalyzed reaction, using binding energy to lower the activation barrier. Second, enzyme active sites are organized by evolution to facilitate multiple mechanisms of chemical catalysis simultaneously. Interactions between Enzymes and Substrates 1. binding energy, ∆GB = energy derived from noncovalent enzyme-substrate (ES) interaction – mediated by hydrogen bonds, ionic interactions, and the hydrophobic effect – formation of each weak interaction in the ES complex is accompanied by release of a small amount of free energy that stabilizes the interaction – major source of free energy used by enzymes to lower the activation energy 2. covalent interactions between enzyme and substrate lower the activation energy Catalytic functional groups on an enzyme may form a transient covalent bond with a substrate and activate it for reaction, or a group may be transiently transferred from the substrate to the enzyme Noncovalent Interactions between Enzyme and Substrate Are Optimized in the Transition State Dihydrofolate reductase “lock and key” hypothesis = enzymes are structurally complementary to their substrates – would make for a poor enzyme – Rarely perfect fit Enzymes Must Be Complementary to the Reaction Transition State the full complement of interactions between substrate and enzyme is formed only when the substrate reaches the transition state Clicker Question 12 How does the concept of “induced fit” support the current theory of substrate-enzyme interaction? A. Enzyme structure is rigid, allowing for competition for the active site and easy ejection of the final product. B. Enzyme conformational changes demonstrate feedback regulation of the enzyme and the ability to “turn off” activity. C. Having a rigid structure allows the enzyme to bind the substrate and stabilize the ES complex. D. Enzyme conformation changes allow additional stabilizing interactions with the transition state and enhance the activity. Clicker Question 12, Response How does the concept of “induced fit” support the current theory of substrate-enzyme interaction? D. Enzyme conformation changes allow additional stabilizing interactions with the transition state and enhance the activity. Some weak interactions are formed in the ES complex, but the full complement of such interactions between substrate and enzyme is formed only when the substrate reaches the transition state. Enzyme conformational changes allow for additional stabilizing interactions between the enzyme and the transition state. The Role of Binding Energy in Catalysis the sum of the unfavorable activation energy ∆G‡ and the favorable binding energy ∆GB results in a lower net activation energy weak binding interactions between the enzyme and the substrate drive enzymatic catalysis Clicker Question 13 Binding energy is defined as the: A. energy derived from noncovalent enzyme-substrate interaction. B. energy derived from release of the product and release from the EP interaction. C. energy released from the conversion of ES to EP. D. difference in the uncatalyzed activation energy and catalyzed activation energy. Clicker Question 13, Response Binding energy is defined as: A. the energy derived from noncovalent enzyme-substrate interaction. The energy derived from noncovalent enzyme-substrate interaction is called binding energy, ∆GB. Binding energy is a major source of free energy used by enzymes to lower the activation energies of reactions. The Active Site of an Enzyme optimized binding energy in the transition state is accomplished by positioning a substrate in a cavity (the active site), removed from H2O Enzyme Specificity specificity = ability to discriminate between a substrate and a competing molecule – given by binding energy Binding Energy Overcomes the Barrier to Reaction barrier to reaction, ∆G‡ includes: – the entropy of molecules in solution – the solvation shell of hydrogen-bonded water that surrounds and stabilizes most biomolecules in aqueous solution – the distortion of substrates that must occur in many reactions – the need for proper alignment of catalytic functional groups on the enzyme Rate Enhancement by Entropy Reduction entropy reduction = large restriction in the relative motions of two substrates that are to react binding energy constrains substrates in the proper orientation to reaction Desolvation of the Substrate desolvation = replacement of the solvation shell of structured water around the substrate with weak bonds between substrate and enzyme – replaces most or all hydrogen bonds between the substrate and H2O that would otherwise impede the reaction Clicker Question 14 What is the primary source of the energy enzymes used to reduce activation energies? A. desolvation of the substrate B. kinetic energy from the transition state C. noncovalent enzyme-substrate interaction D. covalent enzyme-product interaction Clicker Question 14, Response What is the primary source of the energy enzymes used to reduce activation energies? C. noncovalent enzyme-substrate interaction Weak binding interactions between the enzyme and the substrate provide a substantial driving force for enzymatic catalysis. Distortion of the Substrate substrate must undergo distortion (electron redistribution) to react – causes an unfavorable free-energy change binding energy compensates thermodynamically for this The Induced Fit Mechanism induced fit = mechanism by which the enzyme itself undergoes a conformational change when the substrate binds, induced by multiple weak interactions with the substrate – enhances catalytic properties Hexokinase Clicker Question 15 How does the induced fit mechanism of enzyme catalysis work? A. The enzyme assumes a conformation identical to the substrate. B. The enzyme undergoes a conformational change to maximize weak interactions to the substrate. C. The substrate binds the active site of the enzyme. D. The enzyme undergoes entropy reduction to accommodate substrate. Covalent Interactions and Metal Ions Contribute to Catalysis catalytic functional groups aid in the cleavage and formation of bonds by a variety of mechanisms: – general acid-base catalysis – covalent catalysis – metal ion catalysis Enzyme Kinetics enzyme kinetics = the discipline focused on determining the rate of a reaction and how it changes in response to changes in experimental parameters Factors Affecting Enzymatic Activity 1. Enzyme Concentration 3. Temperature 2. Substrate Concentration 4. pH Substrate Concentration Affects the Rate of Enzyme-Catalyzed Reactions pre–steady state = initial transient period during which ES builds up steady state = period during which [ES] and other intermediates remain constant steady-state kinetics = the traditional analysis of reaction rates Initial Velocities of Enzyme- Catalyzed Reactions initial rate (initial velocity), V0 = tangent to each curve taken at time = 0 – reflects a steady state at the beginning of the reaction, [S] is regarded as constant Effect of [S] on the V0 of an Enzyme-Catalyzed Reaction [S], M V0, M/s 0 0 0.1 9 0.2 15 0.5 26 0.7 30 1.0 34 1.3 37 1.5 38 2 40 5 46 Plotting V0 vs. [S] the plateau-like V0 region is close to the maximum velocity, Vmax Clicker Question 20 After you mix a substrate and enzyme together, there is an initial transient period, the pre–steady state. What happens during the pre–steady state? A. [Et] decreases until it reaches a constant level. B. [S] decreases until it reaches a constant level. C. [P] increases until it reaches a constant level. D. [ES] increases until it reaches a constant level. Clicker Question 20, Response After you mix a substrate and enzyme together, there is an initial transient period, the pre–steady state. What happens during the pre–steady state? D. [ES] increases until it reaches a constant level. When the enzyme is first mixed with a large excess of substrate, there is an initial transient period, the pre– steady state, during which the concentration of ES builds up until it reaches a constant level. General Theory of Enzyme Action Proposed by Michaelis and Menten step 1 – enzyme and substrate combine to form a complex in a reversible, relatively fast step: k1 E + S ⇌ ES (6-7) k-1 step 2 – the complex breaks down to yield the free enzyme and the reaction product in a slower step: k2 ES ⇌ E + P (6-8) k-2 because the second step limits the overall reaction rate, the overall rate is proportional to [ES] The Saturation Effect Vmax is observed when virtually all the enzyme is present as the ES complex – further increases in [S] have no effect on rate – responsible for the plateau observed Clicker Question 21 Which statement is false about the saturation effect? A. The maximum initial rate of the catalyzed reaction is observed. B. Virtually all of the enzyme is present as the ES complex. C. It is responsible for the linear increase of V0 with an increase in [S]. D. Further increases in [S] have no effect on rate. Clicker Question 21, Response Which statement is false about the saturation effect? C. It is responsible for the linear increase of V0 with an increase in [S]. The saturation effect is a distinguishing characteristic of enzymatic catalysts and is responsible for the plateau observed in the figure. The Relationship between Substrate Concentration and Reaction Rate Can Be Expressed with the Michaelis- Menten Equation the curve expressing the relationship between [S] and V0 can be expressed by the Michaelis-Menten equation: Vmax [S] V0 = (6-9) Km + [S] where V0 is the initial velocity, Vmax is the maximum velocity, [S] is the initial substrate concentration, and Km is the Michaelis constant the Michaelis-Menten equation is the rate equation for a one-substrate enzyme-catalyzed reaction V0, Vmax, [S], and Km are readily measured experimentally Deriving the Michaelis-Menten Equation the overall reaction for the formation and breakdown of ES simplifies to k1 k1 E + SE‡ˆ+ ˆS ˆ⇌ †ˆ ES ES ⎯k⎯ 2 →E + P (6-10) −1k k-1 V0 is determined by the breakdown of ES to form product, which is determined by [ES]: V0 = k2[ES] (6-11) Deriving the Michaelis-Menten Equation, [Et] [ES] is not easily measured experimentally [Et] = the total enzyme concentration (the sum of free and substrate-bound enzyme) free or unbound enzyme [E] = [Et] – [ES] because [S] >> [Et], the amount of substrate bound by the enzyme at any given time is negligible compared with the total [S] Deriving the Michaelis-Menten Equation, Step 1 rate of ES formation = k1([Et] – [ES])[S] (6-12) rate of ES breakdown = k–1[ES] + k2[ES] (6-13) Deriving the Michaelis-Menten Equation, Step 2 steady-state assumption = the rate of formation of ES is equal to the rate of its breakdown when equated for the steady state: k1([Et] – [ES])[S] = k–1 [ES] + k2[ES] (6-14) Deriving the Michaelis-Menten Equation, Step 3 solving for [ES]: k1[Et][S] – k1[ES][S] = (k–1 + k2)[ES] (6-15) k1[Et][S] = (k1[S] + k–1 + k2)[ES] (6-16) k1[Et][S] [ES] = k1[S] + k–1 + k2 (6-17) [ES] = [Et][S] [S] + (k–1 + k2)/k1 (6-18) Deriving the Michaelis-Menten Equation, Km the Michaelis constant, Km = (k–1 + k2)/ k1 substituting Km into the equation: [ES] = [Et][S] (6-19) Km + [S] A Double-Reciprocal, or Lineweaver- Burk, Plot for enzymes obeying the Michaelis-Menten relationship, a plot of 1/V0 versus 1/[S] yields a straight line 1 = Km 1 +V V0 Vmax[S] max (6-26) Clicker Question 25 The Km on a Lineweaver-Burk plot is: A. equal to the y intercept. B. equal to the slope of the line. C. the reciprocal of the y intercept. D. the negative reciprocal of the x intercept. Kinetic Parameters Are Used to Compare Enzyme Activities all enzymes that exhibit a hyperbolic dependence of V0 on [S] follow Michaelis-Menten kinetics where: Km = [S] when V0 = ½Vmax (6-23) Interpreting Km and Vmax Km can vary for different substrates of the same enzyme Table 6-6 Km for Some Enzymes and Substrates Enzyme Substrate Km (mM) Hexokinase (brain) ATP 0.4 D-Glucose 0.05 D-Fructose 1.5 – Carbonic anhydrase HCO3 26 Chymotrypsin Glycyltyrosinylglycine 108 N-Benzoyltyrosinamide 2.5 β-Galactosidase D-Lactose 4.0 Threonine dehydratase L-Threonine 5.0 The Dissociation Constant, Kd for reactions with two steps: k1 k1 E + SE‡ˆ+ ˆS ˆ⇌ †ˆ ES ES ⎯k⎯ 2 →E + P (6-10) −1k k-1 = k2 + k–1 Km (6-27) k1 when k2 is rate-limiting, k2