Final CR Lecture Notes.docx
Document Details
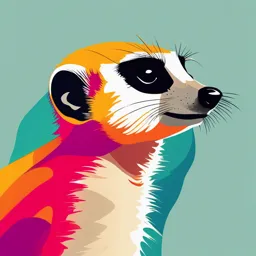
Uploaded by BrilliantVibrance
Full Transcript
`Final CR Lecture Notes 4. Heart Beat and ECG Learning Objectives Describe the structural and functional properties of cardiac muscle, and explain how it differs from skeletal muscle. Briefly describe the events of cardiac muscle cell contraction. Describe how the heart beat is generated. Name the c...
`Final CR Lecture Notes 4. Heart Beat and ECG Learning Objectives Describe the structural and functional properties of cardiac muscle, and explain how it differs from skeletal muscle. Briefly describe the events of cardiac muscle cell contraction. Describe how the heart beat is generated. Name the components of the conduction system of the heart, and trace the conduction pathway. Draw a diagram of a normal electrocardiogram tracing; name the individual waves and intervals, and indicate what each represents. Name some abnormalities that can be detected on an ECG tracing 1. Control of Heart Beat SA Node: group of specialised cells in the wall of the right atrium near the entrance of the SVC. This is the impulse generating tissue (modified cardiac muscle cells). Spread over atria takes 60ms Receives blood from the right coronary artery. AV Node: Electrical activity starts in the SA node, spreads across the atria to the atrioventricular node. Here it is delayed to allow ventricles to fill by 60ms (now 120ms in total). First degree AV block: PR interval is lengthened by 200 milliseconds. Then it travels to the bundle of his, which splits into left and right branches in the interventricular septum. Further divide into purkinje fibres: cause contraction of both ventricles Action Potentials: last about 100 times longer: 200 milliseconds. In SA Node cells: constant inward sodium influx at rest. Simultaneous potassium current outwards prevent depolarization. However, this outward potassium current decays with time until it reaches the threshold level (near -40mV) and the cell depolarizes. The heart rate depends on the rate of decay of the outward potassium current. Parasympathetic (vagal): inhibit closure of potassium channels (muscarinic receptors) Slows heart rate Sympathetic: increase closure of potassium channels (beta adrenoceptor). Speeds up heart rate. Ventricular muscle: unique shape Prolonged depolarization: plateau (prolonged entry of calcium): enter through L type. Longer refractory period: keeps cells synchronous. 2. Electrocardiogram ECG Leads: there are 12 leads that each show a different picture of the heart. A lead is the voltage recorded by two points. ECG: mainly generated by start and ending of action potentials. Bipolar Limb Leads (Frontal Plane) Lead I: Right to left axillae (- to +) Lead II: Right axilla and leg (- to +) Standard ECG. Lead III: records the signal between the left axilla and leg. (- to +) Unipolar Limb Leads (Frontal Plane) Unipolar: amplitude of the signal is calculated between one physical recording point and one virtual reference point (middle of chest). aVR: right axilla to centre (+ to -) Always has a large Q wave and a small/non-exist R wave aVL: left axilla to centre (+ to -) Often very small. aVF: foot (+ to -). Chest Leads: V1 to V6. They are also unipolar leads: virtual reference in the centre of the chest. In order: V1: FOURTH IC space, right side of the sternum Mainly negative (large S wave) V2: FOURTH IC space, left side of sternum V3: Directly between V2 and V4 V4: FIFTH IC at the midclavicular line. V5: Level with V4 at the anterior axillary line V6: level with V5 at the left mid-axillary line. Mainly positive Transfers from negative V1 to positive V6. These different leads view different regions of the heart. E.g. V3 and V4: anterior aspect. Overview of different leads: IMPORTANT. Lead 2: Standard ECG Contain the PQRST waves. P wave: atrial depolarization Should be smooth or round Positive in leads I, II, III Notched/peaked P waves in COPD, CHF. Q wave: negative. Usually small or absent in lead II (if QRS heads upwards). PQ: atrial contraction R wave: positive. Usually present in I, II, II. S wave: negative. Also, present in I, II, II, QRS: ventricular depolarization. ST: ventricular contraction (normally flat and curves upward). T: due to difference in time of repolarisation of ventricles QRS complex: should last <100ms PR interval: should be 120-200ms (greater than this: indicates a form of heart block). 3. Cardiac Vector Take size of QRS complex on two leads: draw them on a triangle. 6. The Heart as a Pump Learning Objectives Explain how the heart is adapted to supply the pulmonary and systemic circulations. Draw a diagram of the pressure profiles in the left atrium, left ventricle and the aorta for a single cardiac cycle. Label the vertical axis in units of pressure. Indicate the points at which the cardiac valves open and close, and the periods of iso-volumetric contraction and relaxation. Explain the origin and significance of heart sounds Describe Starling’s law of the heart and explain how cardiac output is balanced on the two sides. Explain the concepts of preload and afterload. Note the differences between pulmonary and systemic capillaries. Outline the main processes ensuring adequate venous return to the heart 1. Circulation Systemic Circulation: left heart pumps through the aorta to capillary beds of all body tissues. Pressure is high due to vasoconstriction of systemic vessels 120/80 mmHg Generated by moderate size, thick muscular walls. Pulmonary Circulation: right heart pumps through the pulmonary arteries to lungs. No need for high pressure (only lungs are involved) 25/8 mmHg Pulmonary arterial, pressures are lower because the total vascular resistance is much lower than in the systemic circulation. This means a lower pressure is needed in the right heart. Generated by large diameter, thin elastic walls. Larger drop in pressure in the systemic blood pressure: smaller diameter of tubes but greater overall diameter (more of them). 2. Starlings Law and Pumping The heart will pump out all the blood that is delivered into the atria. The more blood, the greater stretched of the ventricle and the stronger the force of contraction. Mechanism: filaments have excess overlap at rest. Stretching increases the amount of overlap of actin and myosin filaments: increases the force of contraction. Preload: the degree of stretching experienced by the ventricle during diastole. Proportional to end-diastolic volume. Normal EDV = 120 ml. Increased preload increased stroke volume (how much blood the heart pumps out per beat increased cardiac output. Normal stroke volume = 70 ml End-systolic volume: residue after ventricles contract = 50 ml (also known as residual volume) NOTE: the ventricles eject the same volume of blood. This balances the output of the two sides of the heart. After Load: the effective flow impendence (resistance) of the aorta and large arteries (depends on diameter and elasticity). Reciprocal of impendence is compliance. The higher the compliance: the lower the afterload. Older people: elasticity of aorta declines (increased also by smoking). The greater the afterload, the longer the period of isovolumetric contraction (ventricles contract but there is no volume change as there is not enough pressure to open the valves). Enlarged heart: enlarged ventricles: no corresponding increase in ventricular wall thickness so the ventricles will contract more weakly than a smaller heart, until Starlings mechanism no longer works. Inotropy: force of contraction of ventricular muscle. Increase in contractility: increase in stroke volume. Can be increased by blood calcium levels, beta adrenergic agonists etc… 3. Valves Kept in position by chordae tendinae (fibrous tendons) attached to papillary muscles (first to contract during systole: pull valves closed). Atrio-ventricular valves = tricuspid and mitral Semilunar = aortic valve and pulmonary artery valve. Valve Sequences: closure/opening of valves produces a lub dub sound. Lub: closing of AV valves, opening of the semilunar valves (S1) Dub: opening of AV valves, closing of the semilunar valves (S2). Gallops (S3 and S4) Low frequency sounds associated with diastolic filling. (S3): faint low-pitched sound heard about 1/3 in diastole. Coincides with period of rapid ventricular filling due to turbulent flow. (S4): fourth heart sound. Sometimes heard immediately before S1. Due to turbulent flow within the ventricle in children. 4. Cardiac Cycle Atrial systole atrial diastole ventricular contraction (consists of phase 1: first contraction that closes the AV until the pressure rises and phase 2: pressure high enough to open semilunar valves) ventricular diastole NOTE: atrial contraction is not essential for normal cardiac output at rest. 5. Jugular Venous Pulse No valves between vena cava and right atrium, or pulmonary veins and left atrium. Contraction of the RA/LA causes back pressure in the form of a faint pulse with three peaks: A: atrial contraction (before tricuspid closes) C: rise in pressure after tricuspid closes V: valve bulges due to ventricular contraction. 6. Venous Return to the heart Mediated by three main factors: One-way valves in the veins: stop blood from falling backwards. Muscular pumps: contraction of muscles in the limbs Thoraco-abdominal pump: during inspiration, pressures in thoracic cavity are reduced, pulling blood into the inferior vena cava. 7. Smooth Muscle and Cardiac Muscle Learning Objectives List the structures where smooth muscle is found Describe the heterogeneity of smooth muscle and link to function Describe the excitation - contraction coupling in smooth muscle and briefly contrast with cardiac and skeletal muscle Explain briefly the electrophysiology of smooth muscle Describe how external factors may contract or relax smooth muscle Describe the innervation of smooth muscle 1. Cardiac Muscle Contains intercalated discs adjoining cardiac myocytes as well as specialised cell-cell junctions (e.g. desmosomes and gap junctions link cells electrically. Permit passage of ions). Have automaticity (spontaneous APs) and rhythmicity (APs in regular and repetitive manner). Electrical Syncytium: gap junctions, intercalated discs allows for rapid and synchronous depolarisation of cells as waves of depolarization can spread to adjacent cells in a synchronous fashion. Action Potentials in CM: their size and shape can differ. There are many ion channels involved in the overall make-up of the response: depolarising currents, overall response and repolarising currents. Calcium signalling: depolarisation of membrane opens voltage gated calcium channels (L-type). Rise in intracellular calcium: triggers further calcium release from the sarcoplasmic reticulum. Calcium then associates with troponin C in the sarcomere: causes contraction. 2. Smooth Muscle (involuntary non-striated muscle) Smooth muscle structure: consist of a loose lattice: thick and thin filaments: run obliquely. Intermediate filaments: assist in the transmission of force generated by contraction Dense bodies: serve as attachments for thick and thin filaments. Contains two types of attachment: Mechanical attachment between cells Gap Junctions: provide a pathway for passage of electrical signals. Location: fond in bladder, gut, uterus, blood vessels, bronchi, urethra, bladder. Function: regulation of the diameter of blood vessels, airways, food through GI tract, uterus. Contraction: innervated by the autonomic nervous system. Varicosities: nerves make multiple contacts with the smooth muscle cell at these points. These release neurotransmitters in space surrounding muscle. Organisation: two types in different locations. Multiunit smooth muscle: each cell receives synaptic input. Little electrical coupling: each cell contracts independently of its neighbour. Allow for fine control and gruel responses (e.g. muscle of the eye). Single unit smooth muscle: ANS innervates single cell within a bundle. AP spreads via gap junctions. Contracts a s a functional syncytium. Found in wall so visceral organs: low, steady contractions. Action Potentials in Smooth Muscle Cells: varies (like cardiac APs in size and shape). Can be: A simple spike A simple spike followed by a plateau Spikes on top of slow waves. NOTE: some smooth muscle cells can initiate electrical activity. These action potentials in smooth muscle lasts much longer (10-15ms) than in skeletal muscle (2ms). Depolarisation depends again on opening of voltage gated calcium channels. Calcium channels open more slowly than sodium channels, so longer duration than in skeletal muscle. Excitation Contraction Coupling: rise in intracellular calcium occurs through two mechanisms: Depolarisation of membrane (opens VG channels) Agonist induced release of calcium via IP3. Calcium binds to calmodulin and activates the enzyme myosin-light-chain kinase. This phosphorylates a regulatory region on myosin light chains. Increases ATPase activity: myosin head groups bind to actin. Control of Smooth Muscle: includes hormones, neural stimulation by the ANS and local factors. Smooth muscle: expresses a wide variety of neurotransmitter and hormone receptors. 8. Haemodynamics Learning Objectives Define the terms systolic and diastolic blood pressure, pulse pressure and mean blood pressure and state values for these in the normal healthy young adult Explain the relationships between cardiac output, peripheral resistance and blood pressure Explain the concept of arterial compliance, and describe the relationship between pulse pressure, stroke volume and compliance Know the importance of Poiseuille's Law relating vessel radius and resistance to flow, and the relevance of this to changes in pressure in the circulation Be able to comment on the importance of Laplace's Law relating vessel radius and pressure, and how this relates to aneurysm formation. 1. Definitions Systole/Systolic Blood Pressure: contraction of the heart. Maximum pressure in arteries (120 mm Hg). Diastole/Diastolic Blood Pressure: relaxation of the heart. Minimum pressure in arteries (80 mm Hg). Blood pressure is not constant but pulsatile. 120/80: ideal blood pressure. It is high and steady. It can be regulated by relaxing/constricting the input arterioles. (NOTE: local vasodilation = increase in local blood flow). Pulse Pressure: difference between systole and diastole. Mean Arterial Pressure: diastolic + 1/3 of pulse pressure. Hypertension: one or two readings above 140 mm Hg or 90 mm Hg. Prehypertension: a systolic pressure from 120 to 139 mm Hg, diastolic pressure from 80 to 89 mm Hg. 2. Compliance Pulse pressure is smoothed out due to the stretchiness of elastic fibres (and elastin). This reduces work. Windkessel Effect: the effect of compliance of small elastic arteries. The walls of elastic arteries distend when blood pressure rises and recoil when blood pressure falls during diastole. 3. Poiseuille’s Law = Small changes in diameter cause large changes in flow. The flow of fluid through tube depends on the fourth power of the radius of the tube. E.g. if you double the radius: the flow increases by 16 times. In small arterioles: a small change (of 25 to 20 um) means a significant change in flow. 4. Cardiac Output The total blood flow out of the heart (litres/min). C.O. = heart rate x stroke volume. Heart rate is typically 70/minute, stroke volume typically 70 ml. Therefore C.O. = 4900ml/min. C.O. approximately 5 litres/min in a healthy resting man. Measured by: Doppler ultrasound: calculates blood velocity in the aorta Transoesophageal Doppler: makes uses of a probe (oesophagus to thoracic level). Factors affect cardiac output: Heart rate: autonomic innervation, hormones, fitness levels, age Stroke volume: heart size, fitness levels, gender, contractility, duration of contraction, preload. Oxygen Requirements of vital organs: Brain = 700ml/minute of blood (14% resting cardiac output). Heart = 200ml/minute of blood (4% resting C.O.) Kidneys = 1250ml/minute (25% of resting C.O.) This takes up about 40-45% of total C.O. Rest of body: 2.7 to 3.0 L/min. Where this goes depends on the digestive state. Fasting: less than 0.5L/min to gut. Digesting: at least 1.5 L/min goes to gut. Cardiac Output and Exercise In exercise C.O., can increase x4, oxygen uptake by x10. Ventricular filling still take place fully even if time is shortened due to the contraction of the atria. 5. Viscosity of Blood and Diameter of Arterioles: Work of Heart Viscosity: thickness of blood Depends mainly on the haematocrit (proportion of red cells in blood, usually ~45%). Polycythaemia: if haematocrit rises to 60/70%. Can be due to excessive production of RBC (absolute) or decrease in volume of plasma (relative). Viscosity also depends on the mechanical properties of erythrocytes. Capillaries may be 5 um or less small than the diameter (7 um) of red cells, so these cells must bend/deform to enter. If cells are too large: clog the capillary (sickle cell). Mechanism: calcium entry into erythrocytes: triggers the release of NO: relaxes RBC. If blood does not move through a vessel: clots form in stagnant blood Individuals with AF: high risk of stroke due to clots forming in auricles of atria. 6. Laplace’s Law Pressure that an elastic vessel can withstand depends on the tension produced in the wall by their elasticity divided by the radius of the vessel. The smaller the radius: the greater pressure the wall can withstand. 9. The Sympathetic Nervous System Learning Objectives Describe the anatomy of the sympathetic nervous system, with reference to the innervation of blood vessels. Describe the transmitters used by the sympathetic nervous system. Describe the role of the sympathetic nervous system in the control of blood pressure and cardiac output Describe the changes in the blood flow to muscle during exercise Describe how the sympathetic nervous system reacts after injury or haemorrhage 1. Anatomy of the Sympathetic Nervous System Thoracolumbar output: (T1-L2) originates (preganglionic motor) at the lateral horns of the vertebrae. Neurons project to the sympathetic chain: series of ganglia anterior to the vertebrae. These ganglia are connected vertically by the sympathetic trunk. Preganglionic postganglionic in these ganglia. Supply the vasoconstrictor nerves to arterioles in blood vessels (chest, upper limbs, neck). Cervical Ganglia: three ganglia at the top of the sympathetic trunk. Not connected to cervical ventral roots but only to the thoracic chain below. The largest of these: superior cervical ganglion. Sympathetic supply to the head These sympathetic fibres nerve plexuses around the carotid arteries: enter the skull. Preganglionic neurons acetylcholine postganglionic nicotinic receptor noradrenaline on smooth muscle via alpha 1 adrenoceptors. Heart: upper thoracic sympathetic chain: cardiac plexus around aorta/atria. Lungs: like the cardiac plexus: pulmonary plexus around large bronchioles. Below the diaphragm: sympathetic ganglia: long preganglionic axons (abdominal). Known as pre-vertebral ganglia (e.g. coeliac ganglion: lies below the diaphragm in front of the aorta). Adrenal Gland: some preganglionic neurons: don’t stop at the ganglia, travel out to the adrenaline nerve. Adrenal gland sits on top of kidneys. Each gland has a cortex (outer part) and medulla (inner part). Adrenaline is secreted from the medulla. Made: noradrenaline dopamine DOPA tyrosine. Sweat Gland: exception that postganglionic nerve fibres are noradrenergic. They use acetylcholine. 2. Functions of the Sympathetic Nervous System SNS is tonically activate at low levels (BASAL TONE). Functions in regulating the distribution of blood. Postural hypertension; can be caused by drugs that inhibits the SNS. No tonic vasoconstriction of blood vessels in e.g. the legs, which causes the blood pressure to drops. The brain and the heart are exceptions to this tonic regulation of blood by the SNS. Exercise: muscles need more oxygen. Chemical factors are released: local vasodilators. This would cause for a drop-in blood pressure if this was not regulated by the SNS system (increases heart rate, therefore C.O.) Produces the fight or flight response, as well as constricting blood vessels, releasing glucose from liver into blood, increasing cardiac output and respiration as well as dilating airways. Other actions: Dilation of the pupil Ejaculation (erection mediated by parasympathetic system). Inhibition of peristalsis in the gut. 3. Adrenaline Receptors. Receptors for adrenaline/noradrenaline. Types: Alpha Receptors: noradrenaline mainly acts on alpha receptors. Two types of alpha receptor: Alpha 1: main receptor on smooth muscle (causes vasoconstriction). ANTAGONISTS (e.g. prazosin): reduce blood pressure. Also, causes contraction of non-vascular smooth muscle (ureter, uterus, hair etc…) Alpha 2: pre-sympathetic nerve terminals. Reduced noradrenaline release by negative feedback. AGONISTS (e.g. clonidine) given to lower b.p. as they inhibit noradrenaline release. Also, inhibits insulin release in the pancreas. Beta Receptors: adrenaline works mainly on beta receptors. Three types: Beta 1: found in the heart: increases force and rate. ANTAGONISTS (e.g. propanol): reduce heart rate and force. Also, involved in increases renin secretion from JGA. Beta 2: found in bronchial smooth muscle: cause bronchodilation. AGONISTS (e.g. salbutamol) give to relax smooth muscle in asthmatics. Decreases motility in GI tract, stimulation of insulin secretion Beta 3: stimulates lipolysis in adipose tissue cells. 4. Exercise and Injury Exercise: both alpha and beta active. Increased energy supply to exercising muscles. Alpha receptors: redistribute blood to muscle Beta 1: increases the cardiac output. Beta 2: relax bronchial smooth muscle: increase ventilation and oxygen uptake. Beta 2 + 3: increase glucose release from liver, increase glucose uptake in muscle. Injury: SNS draws blood from venous supply (through venoconstriction). This will maintain CO and BP. If blood loss is severe; arterial vasoconstriction of vessels in non-essential organs (gut and skin): maintain BP and perfusion of vital organs, increase renin and ADH release. Stress: activates the HPA (hypothalamo-pituitary adrenal) axis: releases ACTH. Stimulates cortisol release from the adrenal cortex. This releases glucose and adrenaline. 10. Neuronal Control of Blood Pressure Learning Objectives Describe the physiological sensors and effectors for the neuronal control of arterial blood pressure. Describe the position/innervation of the aortic/carotid sinus baroreceptors, their central connections, role of the brainstem in the control of blood pressure. Describe the role of the vagus nerve and the sympathetic nervous system in the control of blood pressure. Two systems that regulate BP: neuronal (moment to moment regulation: standing up vs sitting down) and hormonal (slower time: is the renin-angiotensin-aldosterone system. 1. The Baroreceptor System Fast homeostatic process that works through negative feedback. Works through baroreceptors: these are found in the carotid sinus (dilated base of ICA) above the bifurcation of the carotid arteries. Baroreceptors may also be present in the aortic sinus (just above the aortic valve). Next to the carotid sinus carotid body: contains the carotid chemoreceptors. There baroreceptors measure BP through stretch. Depending on the amount of stretch, they send a train of action potentials (through sensory nerve endings) through the brain. These travel through: Vagus: parasympathetic nerve (more in BB). Glossopharyngeal: primarily sensory (more in BB). 2. Increase in Blood Pressure Increases stretch in the baroreceptors: increased number of APs send to the brain via CN IX and CN X. These APs feed into the nucleus of the solitary tract (NTS) (integrating sensory for sensory nerves) located in the dorsal surface of the caudal (lower) medulla. This projects to the vasomotor centre. Located on the rostral ventrolateral medulla. Contains the set point for BP. It also projects to the nucleus ambiguus: contains vagal output neurons to the heart (involves in regulating heart rate). 3. Low Blood Pressure If the rate of firing is too low: the output of the vasomotor centre is increased. The VC projects down through the spinal cord in the lateral reticulospinal tract to sympathetic pre-ganglion neurons in the thoracolumbar region. NOTE: LRT arises from reticular formation from medulla. Also, the NTS will cause there to be an increase in SNS. Results in constriction of arterioles (raises total peripheral resistance (TPR)). NOTE: mean arterial pressure = CO x total peripheral resistance 4. High Blood Pressure If baroreceptor input is too high: vasomotor centre is inhibited. Leads to a reduction in sympathetic outflow. Reduces TPR. The nucleus ambiguus is also excited. This increases parasympathetic vagal output to the heart, effectively acing on the SA node to slow down the heart beat and reduce CO. 5. Hypertension If blood pressure is above 140/90. Mainly due to raised TPR by chronic narrowing/constriction of arterioles. Blood pressure in hypertension is still controlled, but the set point in the vasomotor centre is too high due to: Stretch receptors in carotid/aortic sinus being less sensitive. This leads to a smaller rate of firing: body thinks the blood pressure is too low and needs to increase it. Reduced Sensitivity of Carotid Sinus: can be due to atheroma formation. The walls stiffen so are less sensitive to stretch. An atheroma may also occur on the vasomotor centre. 6. Type A and Type B Personalities: stress may contribute to hypertension, increasing the output of the sympathetic nervous system. Type A personalities are much more likely to be affected. 11. Carriage of O2 in the Blood Learning Objectives Describe the structure of haemoglobin and explain why it is uniquely suitable for carriage of oxygen in blood Explain the significance of the subunit structure of haemoglobin and the consequences of mutations in the haemoglobin genes Comment on the significance of methaemoglobin in erythrocytes Draw a fully labelled diagram of an oxygen-haemoglobin dissociation curve. Explain how the curve shifts under the influence of temperature and pH Explain what is meant by the haematocrit and how it is regulated Explain the ways in which carbon dioxide is transported by the blood. 1. Introduction to Red Blood Cells Carriage of O2 = a problem because oxygen is a powerful oxidising agent. Haemoglobin: unique molecule that can reversibly combine with O2 without being oxidised. It is made up of four subunits: each with a haem prosthetic group attached. Bound together by hydrogen bonds, hydrophobic interactions It is found in erythrocytes: these are biconcave disks about 7 um in diameter, 2 um thick. Sizes may vary in diseases e.g.: microcytic anaemia and macrocytic anaemia One erythrocyte: contains about 270 million haemoglobin molecules. Mature RBCs have no nuclei or mitochondria: easily damaged by O2. Reticulocytes: are RBCS in the bone marrow (premature). Change into mature RBCS after a day in circulation. Glycolysis: RBCs produce ATP by glycolysis as they have no mitochondria. Lactic acid = lower pH. Methaemoglobin: RBCS contain antioxidants (vitamin C) to protect them against oxidative damage. However, still progressively damaged. Haemoglobin methaemoglobin. 120-day lifetime (changes by methaemoglobin: allows recognition by phagocytes). Methaemoglobin reductase: most methaemoglobin formed converted back in newly formed RBC. Methaemoglobinemia: Only 1-2% of haemoglobin is methaemoglobin. If it is higher than this: Methaemoglobinemia. May occur in individuals with congenital deficiency of methaemoglobin reductase. Polycythaemia (more blood cells than normal). 2. Structure of RBCS Ability to carry O2 depends on the ability of the iron atom to be hexavalent. Ferrous iron: has 6 free unpaired d orbital shells = forms 4 electrons in a plane held by covalent bonds in to nitrogen atoms porphyrin ring. This ring with its ferrous ion centre haem group. Fifth iron: binds to histidine group. Leaves 6th iron: which can form weak reversible bonds with O2. Due to steric hindrance, the O2 cannot move close enough to completely remove the electron. Still gradual methaemoglobin. 3. Forms of Haemoglobin Several forms of haemoglobin subunits (different aa sequences). All subunits: contain a haem group, but the amount of steric hindrance varies. Adult Haemoglobin: normally made up of two alpha, two beta subunits Foetal Haemoglobin: two alpha, two gammas subunits. Higher affinity for oxygen (removes it from placental blood). 2, 3 DPG: bound loosely in the centre of the haemoglobin. Enhances ability of RBCs to release O2. 4. Oxygen haemoglobin Dissociation Curve Percent Saturation: proportion of haemoglobin bound to O2 (usually between 96 and 99%, above 94). Hypoxaemia: SaO2 (arterial oxygen saturation) below 90%. Oxygen saturation ≠ tissue oxygenation (and therefore oxygen unloading). O2 unloading is described by: Oxygen Haemoglobin Dissociation Curve: describes progressive binding of O2 to haem subunits. S-shaped: flat at high pO2, steep at medium/low pO2. This is because as O2 binds: subsequent binding is easier. Flat upper part: Hb is more than 90% saturated (> 70 mm Hg) Steep middle part: Hb releases large amounts of oxygen for a small decrease in pO2 (20 – 40 mm Hg). Heat: (due to heavy metabolising): moves curve to the right, unloads more O2 Bohr Shift: heavy metabolising generates more CO2 which is acidic. This moves the curve to the right: unloads more O2. Myoglobin: a form of haemoglobin in muscle. Single subunit: greater affinity for O2 than haemoglobin. Serves as a buffer store for O2 (binds first to myoglobin before Hb). Rhabdomyolysis: when myoglobin is released from damaged muscle. May cause renal failure. 5. Haematocrit Haematocrit (percentage of RBCS: usually 45%). indicates how much O2 is carried by the blood. This is controlled by erythropoietin. Erythrocytes as transport of CO2 √ (IB Review). 12. Microcirculation and Oedema Learning Objectives Draw a labelled diagram showing the anatomy of the microcirculation. Describe the three types of capillaries, continuous, fenestrated and discontinuous, about their structure, distribution and function. Describe how lipid insoluble molecules can move across the capillary endothelium. Draw a labelled diagram showing filtration and reabsorption of fluid along an average capillary. Explain how capillary pressure, plasma colloid osmotic pressure and interstitial colloid osmotic pressure can affect fluid movements between plasma, interstitium and lymph. Know the different causes of oedema and explain the pathophysiology of each. 1. Microcirculation Includes: small arteries, first-order arterioles, terminal arterioles, capillaries and venules. Metarteriole: directly from terminal arterioles to venules). Anatomy of a capillary bed: Arteriovenous Anastomoses (Metarteriole). 2. Structure of Capillaries Composed of endothelial cells with tight junctions surrounded by basement membrane. Capillaries contain NO SMOOTH MUSCLE. Continuous Capillaries: Least permeable, most widely distributed capillary. Sealed endothelium and basal lamina. Only allow small molecules (H20, ions) to diffuse. Two types: Numerous transport vesicles: skeletal muscle Few transport vesicles: located in BBB. Fenestrated Capillaries: Continuous closed basal lamina but epithelium contains small circular pores (60-80nm). This allows for free passage of H20, salts from plasma to tissue. Found in tissues specialized for bulk exchange. Kidney, pancreases, intestines. Sinusoidal/Discontinuous Capillaries: Highest permeability, have larger fenestrations (of 30-40um) to allow RBCs, WBCs, serum and proteins to pass. Have a discontinuous basal lamina. May have gaps even in between tight junctions. Liver, spleen, bone marrow. 3. Solute Exchange in Capillaries Diffusion: Fick’s law (amount moved = area x conc. gradient, diffusion coefficient). Depends on density of capillaries (high density = high diffusion rate). Concentration gradient: net rate of diffusion of a substance through any membrane ~ difference between the two sides. Diffusion coefficient: time it takes for substance to move through material Paracellular Transport: Substances passed through an intracellular space/cleft. Often confined to water-soluble substances Pass through fenestrations (large gap) or calveoli (form vesicles). 4. Fluid Exchange Across Capillaries Determined by hydrostatic and oncotic pressure. Delivers O2, nutrients, etc… Hydrostatic Pressure: favours filtration at the arteriolar end and reabsorption at the venous end of the capillary. Arterial end = 35 mm Hg Venous end = 17 mm Hg Oncotic Pressure: large colloid oncotic pressure throughout capillary pulling substances inwards. Net Filtration Pressure: (HPc - HPif) - (OPc - OPif). Arterial end = 10 mm Hg (water out) Venous end = - 8 mm Hg (water in). 6. Starling’s Hypothesis Oncotic pressure (plasma proteins): draws fluid into capillary so that with hydrostatic pressure water leaves the capillary at arteriole end and is reabsorbed at the venous end (plasma proteins cannot leave) Any surplus fluid is deposed in the lympathic system. Fluid loss: 4000 litres of plasma passes through capillaries/day. 0.1-0.2% filtered. 7. Lymphatic System and Oedema Lymphatic System: specialised vessels made up of an endothelium with large intracellular gaps surrounded by permeable b.m. Contain one-way valves. Water originally from capillaries reabsorbed at the subclavian veins or reabsorbed into circulation at lymph nodes. Approx. 2-4L of lymph is returned to the circulation daily. Failure in correctly distributing it OEDEMA. Oedema: fluid retention. Too much fluid in tissues. Systemic: occurs first in lower body (recognised by pitting). Pulmonary: air spaces in lungs. Causes of Oedema: Increased secretion/filtration of fluid into the interstitium/impaired removal. Increased capillary hydrostatic pressure (more fluid forced out): heart failure, venous construction Decreased plasma oncotic pressure (more fluid escapes/less is pulled in). Kwashiorkor: severe malnutrition. Lack of proteins in blood and tissues causes reduced oncotic pressure so water is not retained by capillaries. Increased capillary permeability (allows water to flow more freely). Lymphatic obstruction (inflammation of lymph vessels, obstruction of lymph drainage). Treatment: osmotic diuretics (increase water excretion) or loop diuretics (increase sodium excretion). 13. Pulmonary Circulation Learning Objectives Outline the responses of bronchiolar and arteriolar smooth muscle which maintain a balance between ventilation of the alveoli and blood flow (perfusion) to the alveoli in normal conditions. Define the term ventilation perfusion ratio (Va/Q) and give values for the whole lung, the base and the apex of the lung in a normal subject in the upright position, explaining why these differ. Illustrate, by means of diagrams, how the perfusion of the lung may be considered in terms of different zones. Comment briefly on the effects, on blood passing through the lungs, of a high or a low Va/Q. Briefly explain the changes in circulation at birth (this will be covered again in more detail in the human development course). Two types of circulation in the lung: Bronchial: supplies the trachea and bronchi. It arises from the aorta, part of the systemic circulation. It makes up about 2% of left ventricular output. Pulmonary: from the right ventricle, receives 100% of CO. Takes ~5 seconds for blood to pass through lungs. Has ~ 280 billion capillaries, 300 million alveoli. 1. Pulmonary Circulation Anatomic Features of Pulmonary Circulation: Pulmonary arteries are thin walled and have a larger diameter. Less smooth muscle. Lower pulmonary vascular resistance Vessels: easily compressed/distended. This allows for a low systolic pressure Lower pulmonary arterial pressure Mean Pulmonary Arterial Pressure: very low: ~ 15 mmHg. Pulmonary Vascular Resistance: changes in expiration and inspiration: Inspiration: alveoli expand and compress capillaries that lie beneath them, increasing resistance. However, the extra-alveolar capillaries are slightly pulled apart, decreasing their resistance. Expiration: EA capillaries are compressed and alveolar capillaries expand. 2. Autonomic Nerve Supply Sympathetic nerve supply to lungs via spinal nerves T2 to T6. Postganglionic fibres from paravertebral sympathetic ganglia merge with vagal fibres pulmonary plexus. Little need for neuronal control of blood distribution: little sympathetic vasoconstrictor tone. 3. Zones of the Lung Gravity: when standing up, the pressure at the lung apex is much lower than at the base (higher perfusion at bases). Zone 1: no blood flow in the apices of the upright lung (PA > Pa: alveoli compress capillaries). Healthy lung: there is no zone 1! Zone 2: blood flow is pulsatile: flow occurs during inspiration (Pa < ATM: pulls apart the extra-alveolar capillaries). Zone 3: blood flow is constant: pulmonary arterial pressure and venous pressure are always greater than alveolar pressure (PA). Radioactive xenon: measures distribution of blood flow. 4. Lung Compliance and V/Q Measure of how stretchy/distensible the lungs are. Compliance = change in volume per unit pressure change (C = dV/dP). Work of breathing: least when inflation/deflation occurs in region of highest compliance. Compliance is higher at the base than at the apex: higher ventilation. Ventilation and Perfusion Both ventilation (Va) and blood flow (Q): higher at bases. However, blood flow declines more steeply due to gravity. Ventilation Perfusion Ratio: Va/Q. The ratio is lower at the bottom of the lung (more blood flow than ventilation) and higher at the apex (more ventilation than blood flow). Explained by PaO2 and PaCO2 Apex: high paO2 and low PaCO2: good exchange as nearly all the blood coming through is saturated with O2. Base: low PaO2 and high PaCO2. Due to higher blood flow gas exchange isn’t as good, but there is more of it. Airway obstruction: VQ ratio is lower than normal = 0 if no ventilation. Blood flow obstruction: VQ ratio is higher than normal. If no blood flow: V/Q is infinite. 5. Regulation of Pulmonary Blood Flow Pulmonary hypoxia: causes local vasoconstriction. Blood is diverted away from poor ventilated areas and moved to well-ventilated regions. Adaptive system. 6. Pulmonary Circulation during Exercise Increase in CO: increase in pulmonary blood flow. However, no increase in pulmonary arterial pressure as the pulmonary arterial resistance decreases to increase the flow. Done via: Reflex Relaxation: if pulmonary vessels are stretched: smooth muscle relaxes: enlarges vessels. Increased PO2 in alveoli: occurs at the start of exercise and initiates reflex relaxation. Arterio-venous shunts: open in lungs: allow blood to go directly to pulmonary veins (safety). At Birth: the pulmonary resistance is very high (no oxygen). Most of the blood moves from the right atrium to the left atrium through the foramen ovale and from pulmonary artery into aorta via the ductus arteriosus. 15. Renal Structure and Function I Learning Objectives Outline the general organisation of the kidney, ureter, bladder and urethra. Identify the parts of the nephron and describe the role of each component in the physiologic processes involved in urine production. Describe the vasculature of the kidney, relating its unique features to the physiology of urine production and nourishment of the nephron. Identify the components of the juxtaglomerular apparatus and describe its role in regulation of blood and urine volumes and renal homeostasis. Explain the ‘clearance concept’ and how this is used to measure glomerular filtration rate (GFR). State the properties of suitable marker substances and show how clearance, and hence GFR, are calculated. State normal values for the GFR 1. Glomerulus and Bowman’s Capsule Renal arteries travel into the kidney, split to become interlobar vessels small arcuate arteries in the cortex. These arteries: terminate in a glomerulus. Glomerulus: Structure enclosed in a tissue called the bowman’s capsule. First stage of urine formation: plasma filtration, occurs here. Filtration pressure: it has an afferent and efferent arteriole which generate a filtration pressure. Afferent arteriole has a larger diameter, so there is a drop in pressure. This forces fluid through the endothelium into the capsular space = 55 mm Hg. Filtration fraction: ~20% (if more: remaining blood would be too viscous). Capillaries in glomerulus: fenestrated and are covered with a layer of podocytes. If inflamed: proteins can entire urine proteinuria. Glomerular Filtration Rate 25% of CO (1.2l/min) to kidneys. GFR: Total amount filtered: 120-125ml/min. Most of this fluid filtered is reabsorbed, so urine flow is about 1ml/min. Renal Plasma Flow: volume of blood plasma delivered (600 ml/min). 2. Proximal Convoluted Tubule Fluid passes from bowman’s capsule PCT. Here, filtered materials (such as water) can be reabsorbed into the peritubular capillaries. Materials can also be secreted by the capillaries into the BC. Excretion = filtration – reabsorption + secretion. About 2/3 of all water filtered in glomerulus: reabsorbed in proximal tubule. Basal cells in the tubule contain sodium pumps: move sodium into the IF and then into the peritubular capillary. Glucose, amino acids, ions, vitamins can be co-transported. Osmotic gradient generated by Na+/K+ pumps. Water is reabsorbed via osmosis. 3. Clearance GFR can be measured by clearance. Clearance: volume of plasma cleared of a substance per minute. If all material is cleared (filtered and secreted), clearance = RPF. Clearance = urine concentration/plasma concentration x urine flow. Inulin: gold standard for measuring GFR. Completely filtered from plasma/not reabsorbed. However, to measure inulin clearance: infuse inulin over a period of hours to reach steady concentration. Creatinine: clinically, clearance is used to measure GFR. Creatinine: occurs naturally in the body. Freely filtered by glomerulus as well as being actively secreted (this leads to overestimation by 10-20%). Renal Plasma flow: clearance can be sued to measure RPF. Involves the use of PAH (para-amino-hippuric acid). Infused until steady. 4. GFR Expanded Auto regulated: doesn’t change over wide ranges of blood pressures. As the renal blood flow is constant, and the metabolic rate of the kidney (i.e. its oxygen consumption) is constant, the pO2 in the kidney interstitium is a measure of the oxygen delivery to the kidney and thus oxygen carrying capacity of the blood. Therefore, erythropoietin releasing cells are in the kidney. 16. Renal Structure and Function II Learning Objectives By means of labelled diagrams show the changes in volume and osmolality of tubular fluid along the length of the nephron, in the presence or absence of anti-diuretic hormone (ADH). State the source, nature, mechanisms of release of ADH. Describe the stimuli for the release of ADH, explain how ADH controls urine volume and osmolality. Explain how the thick-walled, ascending limb of the loop of Henle plays a key role (in conjunction with ADH) in the production of either a dilute or concentrated urine to meet the requirements of water balance. Distinguish between the terms 'water diuresis', 'osmotic diuresis', 'diabetes insipidus' and 'diabetes mellitus'. NOTE: blood plasma has an osmolarity of about 300 mOsm. 1. Broad Control of Blood Volume Loss of blood = venoconstriction. Slight increase in heart rate and rise in PVR but blood pressure remains. Blood volume control: detects loss and reduces urine flow, increases thirst to compensate for loss. 65% of blood is in the capillaries and veins. This acts as a reservoir in case of haemorrhage. 2. Regulation of Blood Volume Regulated by sensors (neuronal and hormonal) that measure VOLUME Neuronal Volume Sensors: sensory fibres found mostly in RA as well as LA. These act as stretch receptors. As there is more venous return: they become more stretched. Signal blood return to heart/minute. Information travels via vagus to the NTS to obtain information on volume hypothalamus. Previously covered in lecture on neuronal control. Hormonal Volume Sensors: are specialized muscle cells also found in the RA and IVC. If stretched (increased preload), these cells release atrial natriuretic peptide (ANP). Decreases reabsorption of the distal tubule in the kidney: greater outflow of fluid. Sensory cells can also release brain-derived natriuretic peptide. 3. Hypothalamus (OSMOLARITY) Attached to the pituitary gland via the pituitary stalk. Is a structure composed of tiny nuclei packed together There are two important nuclei involved in water balance: the supraoptic and paraventricular nuclei. Contains osmoreceptors: measure osmotic pressure of blood through them. They send axons down the pituitary stalk to the posterior pituitary. They secrete ADH. ADH: antidiuretic hormone: peptide containing 9 aa. If a high blood solute concentration is detected: ADH is released. Increases sodium reabsorption to retain water. This also induces thirst. 4. The Loop of Henle Urine = x4 concentrated as plasma osmolarity. Made possibly by the loop of Henle, which generates concentrated fluid in the renal medulla (can be as high as 1200 mmol/l). Loop of Henle consists of ascending and descending loop: Descending Loop: receives fluid from PCT. Has many aquaporins that allow water to leave the tubule and the fluid to become more and more concentrated. Ascending Loop: active transport of sodium and chloride out of the tubule: keeps ECF of medulla highly concentrated in lower regions while returning it back to normal before it enters the collecting duct. It has a thinner and thicker part. Mechanism of Na+/Cl- Transport Out: occurs mainly in the thicker part of the ascending loop. This thicker part is impermeable to water. ROMK: potassium transporter (ATP dependent). Transport potassium out into the lumen. This creates a positive voltage (10mV) in the tubular lumen. Na-K-Cl Cotransporter (NKCC2): allows these ions to move in passively down their concentration gradients into the epithelial cell. The positive potential generated by the ROMK channels enhances this inward transport. Sodium: actively transporter out of the epithelial cell by the Na+/K+ pump (also provides K+). Chloride moves out passively. Loop Diuretics: e.g. furosemide: block the transport of sodium and chloride out of the loop of Henle by inhibiting NKCC2 channels. 5. Collecting Duct Main action of ADH. Dilute fluid passes from ascending loop distal tubule collecting duct. If channels are open: most water is reabsorbed (low to high solute concentration). If closed it is not. Urea: actively pumped out of the collecting ducts into IF. This further increases solute concentration in renal medulla. Countercurrent Multiplier: process of pumping salts into the extracellular fluid around the loop of Henle. Countercurrent Exchange: provided by vasa recta. These prevent the wash out of blood as it follows the tubule in osmolarity. Supplies cells of the loop of Henle with oxygen without interfering. 17. Hormonal Control of Blood Pressure Learning Objectives Describe the renin-angiotensin-angiotensin system and explain its role in blood pressure regulation List common drugs which can be used to control hypertension and explain their mechanism of action Compared to the neuronal system, the hormonal system works on a slower time scale. 1. Juxtaglomerular Apparatus Sensors for blood pressure in distal convoluted tubule. Three components: Macula Densa Cells: lie in the wall of the distal tubule Detects sodium concentration. If Na + low = GFR is low. Tubuloglomerular feedback: attempt to increase the GFR by relaxing the smooth muscle around the afferent arteriole and increasing the filtration pressure. Mesangial Cells: lie between the afferent and efferent arteriole. Juxtaglomerular cells: lie around the walls of the arterioles. Persisting low Na+ concentration = causes the macula densa cells to signal the juxtaglomerular cells. This release renin into the efferent arteriole. 2. The Renin-Angiotensin-Aldosterone Pathway Renin travels to venous blood, meets angiotensin (secreted by the liver) Renin enzymically cleaves angiotensinogen angiotensin I. Angiotensin I pass in lungs cleaved by angiotensin-converting enzyme (ACE) angiotensin II. Angiotensin II acts on angiotensin II receptors on the lumen of endothelial blood cells. AT1: stimulate the release of NORADRENALINE. Cause a rise in blood pressure via the sympathetic nervous system as well as direct effect on receptors. Noradrenaline: causes further release of renin. Also: found in adrenal cortex. Secrete ALDOSTERONE. AT2: actions on CNS. Currently undergoing research. NOTE: renin can also be released via sympathetic stimulation (innervates kidney). Aldosterone: acts on receptors on the distal convoluted tubule. Increases reabsorption of sodium. 3. Hypertension Malfunctioning of the Hormonal System If the renal artery of the afferent arterioles is narrowed (atheroma formation), there is reduced blood flow into the kidney = reduces GFR. Leads to more sodium being reabsorbed, excess renin release which will raise blood pressure. Treatment of Hypertension: Control of blood pressure after haemorrhage: Water moves into blood: keeps volume, osmolarity decreased. Sympathetic NS constricts veins: reduces venous blood volume. Renin release: due to dilute blood passing through kidney. 18. Paranasal Sinuses and Larynx (Covered in Anatomy Practical) 19. Introduction to the Respiratory System Learning Objectives List the functions of the respiratory system. Name the main structural features of the lungs. Distinguish between respiratory and non-respiratory components of the lungs and show how histological features. Describe the branching pattern of the respiratory tree, commenting on the significance of the cross-sectional area Outline the causes and social impact (in terms of mortality and morbidity) of an) airways disease; and b) respiratory infection Comment on the major social, environmental and occupational factors associated with pulmonary disease. The respiratory system is not just involved in gaseous exchange but also acid-base balance, phonation, warming, humidification of gas and defense against airborne pathogens. 1. Division of the Airways The components of the respiratory tree are: Upper Respiratory Tract: oro-pharynx and larynx. Lower Respiratory Tract: consists of two parts (airways is divided 23 times). Conducting airways (first 16 divisions up to the terminal bronchioles). Volume of about 150-200ml (known as dead space: exhaled first) Gas exchange tissue/respiratory zone (last 7 divisions make: from respiratory bronchioles). Ends with alveolar sacs (alveolus) = 0.2mm. Around 480 million alveoli. Rapid increase in total cross-sectional area. This reduces the inward velocity of the air and allows or rapid gas exchange (greater surface area per Fick’s law). 2. Anatomical Features of the Respiratory System Trachae, bronchi, bronchioles: lining is mainly ciliated pseudo-stratified columnar epithelium. Trachae and bronchi contain cartilage which prevents collapse These parts also contain smooth muscle: controls the diameter. They also contain glands (secrete mucus). Respiratory Airways: a very thin epithelium that facilitates gas exchange. NOTE: The epithelium everywhere except in alveoli is covered with a layer of mucus (traps dust/bacteria). Alveoli therefore has macrophages that are present in high numbers. Mucociliary elevator: mucous moved up towards the throat where it is swallowed. Epithelium is made up of Type I alveolar cells/pneumocytes (squamous, make up 90% of wall). As well as Type II alveolar cells/pneumocytes (cuboidal, make up 10% of area). Produce surfactant: reduces the surface tension and stops alveoli from collapsing. Made up of phospholipoprotein. 3. Partial Pressures of Respiratory Gases Partial pressure = total pressure in gas mixture x fractional concentration of gas. Pressure exerted by one of the gases in a mixture of it occupied the same volume as the mixture on its own. PO2 of dry inspired air = 160 mm Hg. In alveolar gas: incorporate water vapor. This reduces the partial pressure of other gases which changes total pressure in the gas mixture. So, the partial pressure of oxygen will be = 150 mm Hg. PO2 in alveoli = 100 mm Hg PCO2 in alveoli = 40 mm Hg. These pressures vary however between systemic and pulmonary circulation. PO2 is highest leaving the lungs, PCO2 is highest entering lungs. Altitude: oxygen fraction stays constant, total pressure decreases, so PO2 decreases. 20. Gas Exchange and Lung Testing Function Learning Objectives Describe the physical and partial pressures inside the lungs during normal breathing Understand the role and functions of surfactant in the lung Describe the factors that limit gas transfer in the lung Describe the tests used to assess lung function 1. The Fick Principle To maximize gas transport in lungs we have: Large exchange area A thin diffusion membrane A low partial pressure difference A high permeability coefficient. How easily the substances dissolve in the membranes. 2. Partial Pressures Average partial pressure of O2 in alveolar gas is = 100 mm Hg. Due to the ventilation perfusion mismatch (higher ventilation than perfusion in apices): gas in the alveoli in lung apices have a higher partial pressure: closer to tracheal levels of 150 mm Hg. Apical alveolar gas = 135 mm Hg. Gas in the lung bases has a much lower partial pressure. Basal alveolar gas = 92 mm Hg. So, in general, because blood leaving the apices is fully saturated and blood leaving the bases is not (though there is more of it) the blood in the apices will have a higher partial pressure. Difference in Partial Pressures: normally, oxygen transfer is very efficient between alveoli/blood. O2 dissolves easily in the alveolar membrane and binds to hemoglobin. Oxygen in alveolar gas = 100 mm Hg, oxygen is mixed pulmonary venous blood = 95 mm Hg. The difference between this is only 5 mm Hg which makes for easy transfer. Acid-Base Regulation: O2 saturation is not affected by changing breathing rate due to high PO2. However, CO2 transport is less efficient: PCO2 in alveoli = 40 mm hg, in pulmonary venous blood = 46. Changing the breathing rate will affect the excretion: keeps blood pH at desired level. 3. Thoracic Wall and Lungs Two important forces hold the thoracic wall and the lungs in close opposition. Intrapleural Fluid: water molecules in Intrapleural fluid are attracted to each other and resist being pulled apart. Therefore, the pleural membranes tend to stick together. Negative Intrapleural Pressure: pressure gradient created by the sub-atmospheric pressure between the lung surface and the inner chest wall of the thorax (two pleura). Around ~4mm Hg less than ATM even if breathing with open glottis (lungs = ATM). IP decreases in inspiration, increases in expiration. The pleura are two layers of serous membrane: A parietal layer which covers the lungs Visceral layer which covers the inner thoracic wall. Boyle’s Law: if the volume of a gas is made to increase the pressure exerted by the gas decreases (decrease in pressure, air sucked in). 4. Inspiration and Expiration Inspiration: the increase in the size of the lungs: intra-alveolar pressure falls sucking air in. Decrease in pressure is small: only by about 1 mm Hg. Air is sucked in. Expiration: a passive process that usually involves no muscle (elastic recoil of inspiratory muscles). Recoil makes pressure rise: again, only by about 1 mm Hg above ATM. Air is moves out. Caused by: Elastic connective tissue Alveolar surface tension: attraction between water molecules at water-air interface. Produces a force that would make the alveoli collapse if not lined by surfactant. NOTE: per Laplace’s law, the smaller the alveoli, the higher the tendency to collapse P (inward collapsing pressure) = 2T (surface tension) / r (radius). Surfactant: mixture of lipid and protein secreted by T2 pneumocytes. Four different lipoproteins: B + C (classic type): reduces surface tension. A + D: coats bacteria and viruses, helps the immune system. (In newborns: fetal lungs cannot synthesize surfactant up to week 28). Premature babies can have infant respiratory distress syndrome. 5. Lung Function Testing Spirometry: assess prognosis of respiratory disease, assess severity, effect of treatment etc… Measurement of total lung capacity (TLC): measured by inspiration of a fixed volume of gas (helium). Peak flow measurement: assesses airway resistance. Useful in patients with asthma/COPD. Full inspiration short sharp blow. Average of three attempts is taken. Test of Ventilation/Perfusion: analyse with isotope scanning. Reduced ventilation/perfusion causes hypoxaemia (low O2 in blood). Measurement of gas transfer: efficiency of O2 transport across alveolar membrane Takes measurement of alveolar oxygen partial pressure (oxygen meter). Compares it to measurement of arterial oxygen partial pressure (pulse oximeter). 21. Pharmacological Treatment of CV Disease Learning Objectives Describe the role of the vascular endothelium. Briefly describe how drugs may be used for treatment of hypertension and angina. Define heart failure and describe its treatment. Describe the renin-angiotensin-aldosterone system and explain its role in blood pressure regulation 1. Hypertension Defined as having a blood pressure of 140/90 or higher. Most types of hypertension: asymptomatic, with causes often not being known (idiopathic). May be secondary to another condition (kidney diseases, excessive sympathetic drive etc…) Stage 2: clinical pressure is 160/100 mm Hg or higher Severe: clinical pressure is 180/110 mm Hg or higher. Treatment: involves adapting environment/lifestyle and the administering of pharmacotherapy. Angiotensin Converting Enzyme Inhibitors (ACE): interrupt the renin-angiotensin system. Ramipril, enalapril (the PRILS). Side effects may be K+ retention, cough. Calcium Channel Blockers: block Ca entry to vascular smooth muscle, myocardial cells. Amlodipine. Side effects: flushing, headache, oedema. Diuretics: decrease intra-vascular volume and therefore CO. Other Options: include some of the following: Alpha adrenergic blockers (prazosin) Aldosterone blockers Beta blockers (used in heart failure, MIs). Treatment Ladder: A: given if aged under 55 years C: given if aged over 55 years. A+ C: A combination is given in Step 2, A+C+ D: Thiazide-like diuretic is added in Step 3. 2. Heart Failure An increased venous return usually leads to stronger contractions of the heart (Starling’s law). This adaptation fails in heart failure (may be due to ischemic heart disease/valve defects etc…). Heart failure can be worsened by compensatory mechanisms. Treatment: modify physiological compensatory mechanisms that worsen it if primary cause not corrected: Aims to improve stroke volume Reduce pre-load as well as after-load Improve tissue perfusion/oxygenation. Treatment again involves making adaptations to lifestyle (control fluid intake, minimize salt intake). Drugs: Diuretics ACEs (corrects the renin-angiotensin system) Positive ionotropes (e.g. digoxin). Digoxin: selectively binds to and inhibits myocyte Na pump (Na/K ATPase). This causes an increase in calcium which causes improvised contractility. Direct action on conduction at AV (decreases automaticity). Has a narrow therapeutic effect. 3. Angina Pain arising from ischemic heart disease of myocardial muscle (due to plaque formation). In other works, the myocardial muscles demand for oxygen exceeds the supply Treatment: works to increase the supply or reduce the demand. Nitrates: e.g. glyceryl trinitrate (short-acting). It has a dilatory effect on vessels (including coronary vessels, reduces preload. Side effects: head ache, drop in blood pressure. Beta Adrenoceptor Antagonists: reduces work of myocardium and hence oxygen demand. Calcium Channel Blockers 4. High Cholesterol Excess of some lipoproteins (LDL) associated with CVS disease. It has multiple causes. Treatment: involves and adaption to the diet as well as administering of drugs. Statins: one of the most prescribed drugs in the UK. Inhibits HMG Co-A. Ezetimibe: blocks cholesterol absorption from the small intestine. PCSK9 Inhibitors: monoclonal antibodies. Target and inactivate PCSK9 in the liver. Causes reduced levels of LDL. 22. Structure and Function of Blood Learning Objectives What is blood made of? What is plasma? What are the blood cells? How are they produced? What is anaemia? How do we detect haematological problems? 1. Plasma Forms 20% of extracellular fluid. Consists of: Water (90%): acts as a solvent, lubricant, cushion (buffer), heat dissipater. Glucose/Salts/Other Dissolved Chemicals (2%) Proteins (8%): Albumin (60%) Globulin (36%) Fibrinogen (4%). Plasma Ions: inorganic ions (potassium, sodium, calcium, chloride, bicarbonate, phosphate). These ions need to be balanced to maintain blood pressure and fuel muscle, heart contractions. Plasma Proteins and Carriers: plasma is involved directly in producing an immune response. Complement proteins: permeability, chemotaxis, enhance ability of antibodies. Cytokines: released from lymphocytes. Antibodies: protect against infection. Various other roles: blood clotting, waste disposal, hormones, drugs, osmotic effects etc… 2. Production of Blood Cells Known as the process of hematopoiesis Bone marrow. Bone marrow stem cells (hematopoietic cells): undergo differentiation into myeloid stem cells: Erythrocyte Megakaryocyte: platelets Myeloblast: eosinophil, basophil, neutrophil. 3. Anaemia Always an underlying cause: one of the most common blood disorders (25% of people). Defined as: <130 g/l of hemoglobin (males) <115g/l for females. Symptoms: tiredness, fain, shortness of breath, rapid heart beat (palpations). Signs: pale, bounding pulse, cardiac failure etc… The types of anaemia are divided based on the mean cell volume (MCV). Iron Deficiency Anaemia: iron is a key component of Hb. Leads to the production of small, microcytic cells instead. Can be caused by: Pregnancy, infection, menstrual loss, bleeding ulcers etc… 4. Platelets Small circulating cells without a nucleus. Fragment of megakaryocytes from bone marrow. Production of one million per second, 10-day lifespan. Hemostasis: platelets form a plug: clotting protein produces a form thrombus. Thrombocytopenia: low platelet levels breeding and bruising. Hemophilia: low clotting actors. 5. White Blood Cells Granulocytes Neutrophils: twice as big as RBCs, 2-4 lobes. Most common WBC, half-life of 6-8 hours. 1 million/second. Neutrophilia: infection, inflammation, tissue damage, haemorrhage. Neutropenia: overwhelming sepsis, HIV, bone marrow failure. Eosinophils: bilobed nucleus, bright orange granules. Increase in parasitic worm diseases, skin disorders, asthma. Basophils: 2-3 lobed nucleus (often can’t see them due to blue histamine levels). Monocytes: large kidney shaped nucleus. Similar function to myeloid cells: turn into macrophages. These present foreign substances to lymphocytes. Lymphocytes: small and dense with little cytoplasm, contains few granules. 6. Detecting Haematological Problems Red Blood Cells: the absolute number and the mean cell volume. Hemoglobin: mean cell hemoglobin: reduced if the cells are small. Full Blood Count: measures the cell/plasma components (hematocrit)/packed cell volume (PCV). Platelet count White cell count. Blood Films: spread on glass. Used to examine morphology of the cells. 25. Respiratory Physiology I: Lung Mechanics Learning Objectives Understand which lung volumes can be measured directly on a spirometer and those that cannot. Understand measurement of airflow (FEV1/FVC) Describe the role of the respiratory muscles in (a) quiet and (b) forced/vigorous breathing. Describe what is meant by the term “dead space” of the lungs (anatomical and physiological) Define and calculate alveolar ventilation 1. Lung Volumes Spirometer: closed space: movement recorded as changes in lung volume. Tidal Volume: volume breathed out = volume breathed in. At rest (breathing quietly: each breath is ~ 0.5L). Inspiratory Reserve Volume: the air that can be forcibly inhaled after inspiration of normal tidal volume (3,100mL). Expiratory Reserve Volume: Additional air that can be forcible exhaled after expiration of a tidal volume (250ml). ~ 1,200 mL Inspiratory Capacity (IC): Volume of gas that can be taken into lungs in full inhalation. Can be calculated as: IVR + TV = ~ 3,700 mL Vital Capacity (VC): maximum amount of air person can expel from lungs after maximum inhalation. IRV + TV + ERV = ~ 4, 800 mL Residual Volume: volume of air remaining in the lungs after maximal exhalation (1,200 mL) Functional Residual Capacity: volume of air in lungs, end of passive expiration ERV + RV, is ~ 2400 mL Minute Ventilation: refers to the amount of air, a person breathes per minute. Vitalograph: measures the air flow rather than the air volume. Peak expiratory: maximum rate at which air is expelled from the lungs (litres/min). Forced expiratory volume: how much air a person can exhale in one second. Forced vital capacity: volume of air that can be maximally exhaled. FEV1/FVC: if ratio is <70-80%, can indicate a defect. Restrictive: harder to fully expand airways due to damage to lung tissue. FEV1 is reduced in proportion to loss of lung volume (FVC) so no change in ratio. These two values are reduced by about 20- 30% the normal value. Obstructive narrowing of the airways. FEV1 is lowered due to obstruction while FVC does not decrease much. So, the ratio is decreased. 2. Ventilation (Quiet vs Forced). Quiet Respiration: Inspiration is the only active part. Done by two main muscles: Diaphragm: main muscle in quiet respiration. Innervated by the phrenic nerves. Descends into the chest and draws air in. Compromises 75% of the energy expenditure during quiet breathing. External Intercostals: most active during inspiration. Carry out two types of movement: Pump-handle movements: anterior end of each rib elevated Bucket handle: diameter of chest increases by rib on either side. Forced Respiration: Accessory muscles: assist in elevating the rib cage If used at rest often a sign of respiratory distress Abdominal muscles: Squeeze contents of abdomen against diaphragm, force it up into the chest expelling the air. Internal intercostal muscles: pull rib downwards, decreasing thoracic volume. Most important muscles in expiration and forced respiration. 3. Anatomic Dead Space Alveolar ventilation = portion of total ventilation that reaches alveoli. Anatomical Dead Space: system of tubes connecting alveoli to the atmosphere (pharynx, trachea etc… up to the terminal bronchioles). Approx. 150ml of any breath. Usable portion of TV is therefore 350ml. (Alveolar ventilation per minute is 4200 mL if respiratory rate is 12 breaths/min). Alveolar dead space: dead space due to age/disease (e.g. in alveoli with insufficient blood supply). In healthy individuals, very small (5ml). Physiological Dead Space: anatomic dead space + alveolar dead space. 27. Respiratory Physiology II: Lung Mechanics Learning Objectives Outline the relationships between the lungs, pleura and chest wall. Define the term intrapleural pressure Describe how intrapleural pressure changes during normal breathing and during pneumothorax. Explain how lung volumes are determined by the balance between the elastic properties of (1) lung and (2) chest wall. Draw the pressure-volume curve for the lung and state how it is used as a measurement of compliance. State the role of surface tension (and surfactant) in lung compliance Name diseases that affect compliance of the lung and chest wall Understand the principles of airway resistance, the main sites of airway resistance and factors that affect it. Describe what determines the work of breathing. 1. Elastic Recoil Properties Lung volumes are determined by the balance between elastic properties of the lung and chest wall. Chest wall recoils outwards, lungs inwards which generate intrapleural pressure. Intrapleural Pressure: lungs and chest wall are held together by the cohesive forces of the intrapleural fluid. As the chest wall expands: the lung is obligated to follow. Pneumothorax: intrapleural pressure becomes equal to/exceeds atm. Pressure surrounding the lungs will increase and lungs may collapse. Elastic Fibres of Lungs: elastic fibres form bulk of c.t. present in walls of alveoli. 2. Lung Compliance Elasticity: how easily the lungs can be stretched = compliance. Can be expressed as the slope of the curve in the volume-pressure curve. Normal range: lung is very compliant. Lower pressures: lung is stiffer/harder to inflate, so compliance is less. Greater pressure is needed to inflate the lung than deflate it. Hysteresis: lung volume at any pressure during inhalation are always lower than expiration because you need to try harder to inflate the lungs than to deflate them (lungs are stiffer: less compliance). Also, explains why it is easier to inflate a lung filled with fluid (less pressure required: only elastic forces are working whereas air filled also require surface tension. Reduced Compliance: increase of fibrous tissue in the lung (pulmonary fibrosis), collapse of the lung. Increased compliance: age and emphysema (makes the lungs stretchier): less pressure, easier to inflate. Emphysema: destruction of elastic fibres and collagen: lung is more easily distended. NOTE: a structural change in the thorax (e.g. kyphoscoliosis) can affect compliance. 3. Surface Tension Also, generates elastic properties of lung. Cohesive forced between liquid molecules. The water molecules on the boundary: especially strong attraction to each other (so water is always trying to contract droplets). Same principle in alveoli: trying to collapse (forcing air out into bronchi). Surface tension elastic force 4. Airway Resistance Resistance to the flow of gas within the airways of the lung. Asthma (bronchoconstriction) causes increase of airway resistance. Laminar Flow: movement is orderly and streamlined. Turbulent Flow: movement in chaotic. Sites of Airway Resistance: half of resistance comes from the upper respiratory tract. Increased if cold (through nose), decreased if breathing through mouth. The other half comes from the lower respiratory tract. Although the diameter of tubes decrease, there are more of them so resistance remains the same. Control of airways resistance is done by small bronchi and bronchioles. These contain little/no cartilage but are innervated by smooth muscle that can constrict. 5. Bronchial Smooth Muscle Tone Autonomic Control: Parasympathetic: postganglionic fibres release Ach which stimulate muscarinic receptors on the smooth muscle causing them to contract. Sympathetic: by circulating catecholamines (adrenaline) activates beta 2 receptors. No direct input from sympathetic nerves themselves. Other Factors that Contribute to Tone: Non-adrenergic non-cholinergic systems (NANC): includes bronchodilators (and possibly constrictors) Mediator release (histamine): mast cells, neutrophils, eosinophils: important in asthma. Irritant/cough receptors (fast and slow adapting). NOTE: work is = pressure x change in volume. 26. Acid Base and Control of Respiration 1. Central Chemoreceptors Central chemoreceptors on the ventral surface of the medulla. Contain specialized neurons: sense pH of the CSF. Use CSF as an indicator of blood acidity = CO2 (from blood) moves freely across the blood brain barrier into the CSF, where it forms carbonic acid protons and bicarbonate. Chemoreceptors are Affected by: Increased acidity of CSF: chemoreceptors stimulate neurons in respiratory control centers to increase ventilation. NOTE: Normal pH of CSF = 7.32 (lower because no plasma proteins filtered through choroid plexus so no pH buffering). This means a change in pH in CSF for a certain PCO2 is greater than in the blood (allowing for very sensitive response). 2. Lung Based Respiratory Stretch Receptors Pulmonary Stretch Receptors = found in bronchioles and small bronchi. Hering-Breuer Inflation Reflex: signals end of inspiration through afferents in the vagus nerve to respiratory center in medulla/pons to start expiration Inhibit respiration when lung is fully inflated (prevents damage to lung tissue). Irritant Receptors = found in airway epithelial cells (trachae and large bronchi). Stimulated by smoke, cold air etc… Active cough reflexes to objects too large to be carried by mucous. J Receptors = react to pulmonary oedema, pneumonia and heart failure. Ventilation is increased when J receptors are activated. NOTE: increase/decrease in breathing is due to hypercapnia (increase CO2) and not hypoxia (only if PO2 is less than 60 mm Hg, but before that very little response whereas immediate response to rise in CO2). 3. Peripheral Chemoreceptors (Hypoxia) Located in or around carotid body (most vascularized structure in the human body). Afferents mainly response to PaO2. Signals from these chemoreceptors mainly transmitted through glossopharyngeal. Carotid body is made up of: Glomus type I (chief) cells = release neurotransmitters to stimulate CN IX and CN X Depolarization of glomus membrane if decrease in PO2 or pH. Hypoxia = inhibition of potassium channels. Only active of O2 drops below 60 mm Hg. Glomus type II (sustenacular) cells = resemble glia and act as supporting cells. NOTE: peripheral receptors are opposite to central: respond to mainly to O2 and a little bit to CO2. Medullary Respiratory Centers Reticular formation of medulla beneath floor of fourth ventricle. Project down reticulospinal tract to activate diaphragm Inspiration and Expiration NTS receives input from pH, pulmonary stretch, cough and J receptors. It projects to the dorsal respiratory group which fire during inspiration. These contain an intrinsic rhythm modulated by two other regions Apneustic center (makes inspiration last longer) Pneumotaxic center (inhibits apneustic center). Cheyne-Stokes Breathing = damage to sensory input = gradual increase in depth/rate of breathing, followed by gradual decrease called apnoea. Can be seen in strokes, heart failure. 28. Pharmacology of Airways Asthma = episodes where airflow is limited (can be spontaneous or in response to stimuli). Key characteristic = reversibility Airflow becomes limited because of spams in bronchial muscle, oedema and mucous plugging. 1. Normal vs Asthma Normally, an irritant stimulus produces a low-level response (mild bronchoconstriction). In asthma, low levels of stimulus triggers and exaggerated response. Hyper-sensitivity = normal response to very low levels of stimulus Hyper-reactivity = exaggerated response to a stimulus. 2. Asthmatic Immune Response Normal = antigens produce low level T helper lymphocyte response. Asthma= has a specific mechanism: Antigens cross link with IgE on mast cells, cause release of histamine and various cytokines (IL-3, IL-4). Immature CD4+ to differentiate into TH2 lymphocytes and release more cytokines that recruit inflammatory cell (e.g. leukotrienes, basophils). B cells become IgE producing plasma cells = further stimulate response. Result = inflammatory response, bronchoconstriction, oedema, mucus production. Drugs that may trigger asthma = Beta lblockers (blocks Beta 2 receptor which mediates bronchodilation). Cyclooxygenase (COX) blockers = increase arachidonic acid which causes leukotriene production. 3. Asthma Treatment Works with a treatment ladder that aims to relieve symptoms, modify physical pathway, prevent attacks etc… Step 1 = inhaled short acting beta 2 agonists Step 2 = add inhaled steroid Step 3 = add inhaled long-lasting beta 2 agonist (LABA) Step 4 = further increase steroid, add fourth drug (e.g. leukotriene receptor antagonist) Step 5 = use daily steroid tablet. Bronchodilators Beta agonists = mimic sympathetic nervous system. Short acting (salbutamol) Long acting (salmeterol). Anti-cholinergic/muscarinic = block parasympathetic impact of bronchoconstriction. Phosphodiesterase inhibitors (PDE) = prolong cAMP. Protein Kinase A activation by cAMP phosphorylation intracellular proteins bronchodilation. Anti-Inflammatory Corticosteroids Suppress multiple inflammatory genes activated in asthmatic airways. Anti-inflammatory, immuno-suppressant. Inhaled dominantly, used orally in severe attacks. Alter transcription of pro-inflammatory genes (ILs). Control asthma, do not cure it. Leukotriene Antagonists Add-on treatment, active orally. 4. Delivery of Inhaled Drugs Inhalers: (can be used with spacer) Metered dose inhaler (MDI): Propellant (HFA) pushes drug particles at high velocity into airway. Spacer improves aerosol form, HFA: environmental pollutants. Dry Powder Inhaler (DPI): uses inspiration. No propellant. Requires high inspiratory flow rate. Nebulizers: air, O2-driven. Technique is important (90% of patients: do not use effectively)