Protein Function Biochemistry PDF
Document Details
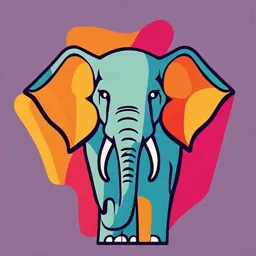
Uploaded by TopNotchHydrogen
Medical School
Tags
Summary
This document details protein function, focusing on myoglobin and hemoglobin, and their interaction with oxygen. It covers ligand binding, conformational changes, and the roles of these proteins in oxygen transport and storage. The text also touches on the structure and interactions of heme and explains how protein structure affects oxygen binding.
Full Transcript
Protein Function Fibrous proteins are structural elements of cells and tissues depends on stable, long-term quaternary interactions between identical polypeptide chains. The functions of many other proteins involve interactions with a variety of different molecules. Most of these intera...
Protein Function Fibrous proteins are structural elements of cells and tissues depends on stable, long-term quaternary interactions between identical polypeptide chains. The functions of many other proteins involve interactions with a variety of different molecules. Most of these interactions are transient, though they may be the basis of complex physiological processes such as oxygen transport and immune function. MYOglobin: MUSCLE, HEMOglobin: BLOOD Protein Function Ligand: A molecule bound reversibly by a protein. (any molecule including protein). Binding site: the site on the protein where ligand binds , which is complementary to the ligand in size, shape, charge, and hydrophobic or hydrophilic character. the interaction is specific: the protein can discriminate among the thousands of different molecules in its environment and selectively bind only one or a few. A given protein may have separate binding sites for several different ligands. Protein Function Proteins are flexible. Changes in conformation UNDERSTOOD, reflecting molecular vibrations and small movements of amino acid residues throughout the protein. A protein flexing in this way is sometimes said to “breathe.” Protein flexibility = Protein breathing Changes in conformation reflects small movements of amino acid throughout the protein. Protein Function phrma 1 The binding of a protein and ligand is often coupled to a conformational change in the protein that makes the binding site more complementary to the ligand, permitting tighter binding. The structural adaptation that occurs between protein and ligand is called induced fit. In a multisubunit protein, a conformational change in one subunit often affects the conformation of other subunits. Interactions between ligands and proteins may be regulated, usually through specific interactions with one or more additional ligands. These other ligands may cause conformational changes in the protein that affect the binding of the first ligand. Enzymes represent a special case of protein function. Enzymes bind and chemically transform other molecules— they catalyze reactions. The molecules acted upon by enzymes are called reaction substrates rather than ligands, and the ligand-binding site is called the catalytic site or active site. Enzymes ligands = substrates. Binding site = active site 1. Storage and transport of ions and molecules Myoglobin and Hemoglobin most studied, best understood protein. 1st three dimensional revealed. - O2 poorly soluble in aqueous soln. Diffusion through tissues is ineffective over distances greater than a few millimeters. - A.A. side chain not suitable to reversible binding of O2. transition Metals (Iron) suitable for this function. - Iron bound + sequestered in molecule making it less active(no ROS) incorporated in heme group (prosthetic group). i.e. Iron is often incorporated into a protein-bound prosthetic group called heme. Heme: - A complex organic ring structure porphyrin bound to ferrous Fe 2+ - porphyrin consist of 4 pyrrole rings - Coordinated nitrogen atoms ((which have an electron-donating character) prevent Fe2+ ( bind oxygen) →ferric Fe3+ ( can not) In free heme molecule→ irreversible conversion to Fe3+ In heme containing proteins, this reaction is prevented by sequestering of the heme deep within the protein structure where access to the two open coordination bonds is restricted NO and CO carbon monoxide bind with greater affinity →toxic Heme: iron has 6 coordination bonds Organic ring structure Porphyrin ring Heme group viewed from the side: 2 coordination bonds to Fe2+ flat porphoryin ring system. One bound to O2 the other to His (proximal His). 1. One of these two coordination bonds is occupied by a side-chain nitrogen of a His residue. 1. The other is the binding site for molecular oxygen (O2) Two histidine in Mb. One anchor the heme group called proximal. The other help bind oxygen called distal that reduce binding the CO. The Structure of Myoglobin Myoglobin: Simple oxygen-binding protein in all mammals primarily Structure of myoglobin: in muscle tissue. - Single polypeptide (153a.a) - 8 cylinders A-H -Nonhelical residues AB,CD … segments that interconnect -The heme pocket made up largely from E and F helices. - has histidine. His93 (the 93rd a.a residue ( the N: of the side chain of His attached to Fe, from the N-terminal end of the myoglobin polypeptide sequence), is also called His F8 (the 8th residue in alpha helix F) A reversible protein-ligand interaction pharma sec2 Myoglobin’s function depends on protein’s ability not only to bind oxygen but also to release it. Reversible binding of a protein P to a ligand L : P + L PL Ka = [PL] -------- [P] [L] Ka= affinity of L to its P. Ka= association constant = M-1 Kd = dissociation constant= 1/Ka = M Kd is equivalent to the molar concentration of ligand at which half of the available ligand-binding sites are occupied Binding equilibrium. ( increase in L but ligand binding sites limited) (Theta) = binding sites occupied/ total binding sites = [PL] --------- [PL]+[P] The more tightly a protein binds a ligand → the lower [ligand] required for the binding sites to be occupied →lower Kd. Dentist next tuesday Graphical representations of ligand binding The fraction of ligand binding sites occupied, θ plotted against [free ligand] Kd = 1/Ka The [L] at which half of the available ligand- binding sites are occupied (at θ = 0.5) corresponds to 1/Ka. Binding of Oxygen to Myoglobin P50 is the oxygen concentration that equals Kd O2 is a gas, conc. described by partial pressure of O2 expressed in kilopascals (Kpa). O2 binds tightly to myoglobin with P50 of only 0.26KPa. Protein Structure Affects How Ligands Bind pharama sect 1 and 2 The interaction is greatly affected by protein structure and is often accompanied by conformational changes. For example, the specificity with which heme binds its various ligands is altered when the heme is a component of myoglobin. Carbon monoxide binds to free heme molecules more than 20,000 times better than does O2, but it binds only about 200 times better when the heme is bound in myoglobin. The difference may be partly explained by steric hindrance. When O2 binds to free heme, the axis of the oxygen molecule is positioned at an angle to the Fe-O bond. In contrast, when CO binds to free heme, the Fe, C, and O atoms lie in a straight line In arterial blood passing from the lungs through the heart to the peripheral tissues, hemoglobin is about 96% saturated with oxygen. In the venous blood returning to the heart, hemoglobin is only about 64% saturated. Thus; each 100 mL of blood passing through a tissue releases about one-third of the oxygen it carries Myoglobin, with its hyperbolic binding curve for oxygen is relatively insensitive to small changes in the concentration of dissolved oxygen and so functions well as an oxygen-storage protein. Hemoglobin, with its multiple subunits and O2-binding sites, is better suited to oxygen transport. Interactions between the subunits of a multimeric protein can permit a highly sensitive response to small changes in ligand concentration. Interactions among the subunits in hemoglobin cause conformational changes that alter the affinity of the protein for oxygen. The modulation of oxygen binding allows the O2-transport protein to respond to changes in oxygen demand by tissues. Protein Structure Affects How Ligands Bind The binding of O2 to the heme in myoglobin also depends on molecular motions, or “breathing,” in the protein structure. The heme molecule is deeply buried in the folded polypeptide, with no direct path for oxygen to move from the surrounding solute on to the ligand binding site. If the protein were rigid, O2 could not enter or leave the heme pocket at a measurable rate. However, rapid molecular flexing of the amino acid side chains produces transient cavities in the protein structure, and O2 evidently makes its way in and out by moving through these cavities. Structures of Myoglobin and the ß Subunit of Hemoglobin structure of myoglobin and the β subunits of hemoglobin: 27a.a. identical their structures are very similar to that of myoglobin, even though the amino acid sequences of the three polypeptides are identical at only 27 positions Most of the amino acids in hemoglobin form alpha helices, connected by short non-helical segments. (Hemoglobin has no beta strands and no disulfide bonds). Quaternary structure of hemoglobin show interaction with unlike subunits. Hemoglobin consists of 2 alpha subunits and 2 beta subunits to give a four chain structure. The quaternary structure of hemoglobin features strong interactions between unlike subunits. The α1β1 interface (and its α2β2 counterpart) involves more than 30 residues, and its interaction is sufficiently strong -Hemoglobin exists in 2 interchangeable structural states: T (tense): stable when O2 not bound (-O2), Deoxy R (relaxed): stable when O2 bound (+O2), Oxy Dominant interactions between hemoglobin subunits In this representation,α- ubunits are light and β subunits are dark. The strongest subunit interactions (highlighted) occur between unlike subunits. When oxygen binds, the α1β1 contact changes little, but there is a large change at the α1β2 contact, with several ion pairs broken The T R transition The rotation of His HC3 residues The transition from the T state (“tense=ion pairs”) to the R state (“relaxed” oxygenated) shifts the subunit pairs substantially, affecting certain ion pairs. Most noticeably, the His HC3 residues at the carboxyl termini of the subunits, which are involved in ion pairs in the T state, rotate in the R state toward the center of the molecule, where they are no longer in ion pairs. Another dramatic result of the T to R transition is a narrowing of the pocket between the beta subunits. The T→ R transition: dentist Sunday The transition from the T state (“tense=ion pairs”) to the R state (“relaxed” oxygenated) shifts the subunit pairs substantially, affecting certain ion pairs Positively charged side chains = blue. Negatively charged side chains/ partners = red. Binding to O2 →conformational change to R Shift in ion pairs mainly HC3 residue rotate toward molecule center. Narrowing the pocket between β subunits. Steric effects on the binding of ligands to the heme of myoglobin(NO QUATERNARY STRUCTURE) (a) Oxygen binds to heme with the O2 axis at an angle, a binding conformation readily accommodated by myoglobin. (b) Carbon monoxide binds to free heme with the CO axis perpendicular to the plane of the porphyrin ring. When binding to the heme in myoglobin, CO is forced to adopt a slight angle because the perpendicular arrangement is sterically blocked by His E7, the distal His. This effect weakens the binding of CO to myoglobin. (c) The bound O2 is hydrogen bonded to the distal His, His E7 (His64), further facilitating the binding of O2. Hb in RBC and oxygen binding In arterial blood passing from the lungs through TWO STATES: OXY HB and DEOXY HB the heart to the peripheral tissues, hemoglobin is about 96% saturated with oxygen. In the venous blood returning to the heart, hemoglobin is only about 64% saturated. Thus; each 100 mL of blood passing through a tissue releases about one- third of the oxygen it carries A sigmoid (cooperative) binding curve 1. A sigmoid binding curve can be viewed as a Release O2 Retain O2 hybrid curve reflecting pO2=13.3kP 4kPa Case 1 a transition from a low- affinity to a high-affinity state. Hb case 2. Cooperative binding, as manifested by a sigmoid binding curve, renders hemoglobin more sensitive to the small differences in O2 Case 2 concentration between the tissues and the lungs, allowing hemoglobin to bind oxygen in the lungs (where pO2 is high) and release it in the tissues (where pO2 is low). EFFICIENT IN BINDING, AND EFFICIENT IN UNLOADING OXYGEN Hemoglobin must bind oxygen efficiently in the lungs, where the pO2 is about 13.3 kPa, and release oxygen in the tissues, where the pO2 is about 4 kPa. Myoglobin, or any protein that binds oxygen with a hyperbolic binding curve, would be ill-suited to this function. A protein that binds O2 with high affinity would bind it efficiently in the lungs but would not release much of it in the tissues. If the protein bound oxygen with a sufficiently low affinity to release it in the tissues, it would not pick up much oxygen in the lungs. O2 dissociation curve of hemoglobin → sigmoidal O2 dissociation curve of myoglobin → hyperbolic - Hemoglobin binds O2 efficiently in lungs (13.3 kPa) release it in tissues (4 kPa). If a Protein binds O2 with high affinity in lungs would not release it in tissues. If it binds O2 with low affinity to release it in tissues, it would not pick up much O2 in lungs. - O2 binding is both allosteric and cooperative. As O2 binds to one site hemoglobin undergoes conformational change affecting other binding sites. - Conformational changes bw the T and R states mediated by subunit- subunit interaction results in cooperative binding described by sigmoid binding curve. Allosteric protein: binding of a ligand to one site affects the binding properties of another site on the same protein. allos greek = other. stereos = solid / shape. Allosteric protein = having “other shapes” / conformation induced by binding of ligand= modulator (inhibitor or activator). Conformational changes →more/less active. Homotropic= normal ligand and modulator are identical. Heterotropic= modulator other molecule than normal ligand. One protein have several modulators homotropic/heterotropic. O2 binding to hemoglobin : O2 ligand + homotropic modulator. Allosteric protein Allosteric: other shapes = conformation Modulator ( activator or inhibitor) Oxygen in Hb Homotropic Heterotropic Same Normal ligand and modulator Different ligand and modulator CO poisining and half time 2-6.5 hrs (outdoor atmosphere) or tens of minutes if oxygen is supplied. Hemoglobin carries end products of respiration H+ and CO2 that stabilize T state. CO2 + H2O ↔ H+ + HCO3- carbonic anhydrase ( abundant in RBC) CO2 insoluble in blood → formation of bubbles in blood and tissues if not converted to bicarbonate. CO2 Hydration to bicarbonate →increase [H+ ] → decrease in pH. Affinity of hemoglobin to O2 ↓ as pH ↓ in peripheral tissue. Hemoglobin transports about 40% of the total H+ and 15% to 20% of the CO2 formed in the tissues to the lung and the kidneys. (The remainder of the H+ is absorbed by the plasma's bicarbonate buffer; the remainder of the CO2 is transported as dissolved HCO3- and CO2). In lungs capillaries CO2 is excreted. in the capillaries of the lung, as CO2 is excreted and the blood pH consequently rises, the affinity of hemoglobin for oxygen increases and the protein binds more O2 decrease PH…..release O2 while increase ….bound O2 Bohr effect = effect of pH and [CO2 ] on O2 binding and release. Hb + O2 ↔ HbO2 (oxygen binds to iron in Heme). HHb + O2 ↔ HbO2 + H+ (proton binds to any of the a.a). His149 (His HC3) of B subunit when protonated ion pair- Asp94 → stabilize T state in deoxyhemoglobin Effect of pH on O2 binding to hemoglobin. pH of blood in lungs = Higher [H+] 7.6 Lower affinity to In tissues 7.2 O2 Lung/tissue O/H+ O2-saturation curve of hemoglobin is influenced by the H+ concentration. Both O2 and H+ are bound by hemoglobin, but with inverse affinity. When the oxygen concentration is high, as in the lungs, hemoglobin binds O2 and releases protons. When the oxygen concentration is low, as in the peripheral tissues, H+ is bound and O2 is released CO2 binds hemoglobin inversely to O2 Binds as a carbamate group to the N-terminal end of each globin chain → carbaminohemoglobin. H+ produced contribute to Bohr effect. Oxygen binding to hemoglobin is modulated by 2,3-bisphosphoglycerate which binds to and stabilize T state. Unlike O2, only one molecule of BPG is bound to each hemoglobin tetramer. BPG binds at a site distant from the oxygen-binding site Heterotropic allosteric modulation (effect): Reduce affinity of Hb to O2. - Physiological adaptation to the lower pO2 in high altitudes. [BPG ] ↑ , affinity to O2 ↓. - O2 delivered to tissues >> 40%. Effect of BPG on hemoglobin binding to O2 : BPG conc in normal human blood= 5mM at sea level, 8nm at high altitudes. Hemoglobin binds O2 tightly when BPG is entirely absent, At sea level hemoglobin is nearly saturated with O2 in lungs. But only 60% in other tissues. At high altitudes O2 delivery declines by one fourth. BPG binding to deoxyhemoglobin: BPG binding stabilizes deoxyhemoglobin T state. BPG -ve charges interact with +ve charges surrounding the pocket bw β subunits in T state In c the binding pocket of BPG disappears on oxygenation following transition to → R state. Mesh surface image T state R state Regulation of O2 binding to hemoglobin by BPG important in fetal development: Fetus has α2γ2 hemoglobin. This tetramer has ↓ affinity to BPG than adult hemoglobin → ↑ affinity to O2. Fetus can extract O2 from maternal blood. Normal uniform, cup shaped erythrocytes: Sickle cell anemia: - a genetic disease caused by a single a.a substitution ( Glu6 –ve charge by → Val6 no charge) in each ß chain of hemoglobin. - The change produces a hydrophobic patch on the surface of hemoglobin that causes the molecules to aggregate (sticky) into fibers bundles when hemoglobin S is deoxygenated. This homozygous condition results in serious medical complications. Erythrocytes in sickle cell anemia: Fewer + abnormal + immature + some blade shaped. Normal and sickle cell hemoglobin: A single a.a change ( Glu 6→Val 6 ) in β chains Deoxyhemoglobin S has a hydrophobic patch on its surface Causing the molecules to be sticky and aggregate into strands that align into insoluble fibers. Insoluble fibers responsible for deformed shape. Normal hemoglobin soluble Hemoglobin S insoluble when deoxygenated. Enzymes Chapter 6 Enzymes -Enzymes are mostly proteins , or catalytic RNAs. Ribozymes (ribo nucleic acid en zyme s) are RNA molecules that have role in RNA splicing in gene expression, similar to the action of protein enzymes. - Highly specialized proteins catalyze biochemical rxn’s (high degree of specificity to substrates) - function at (mild conditions, temperature, neutral pH, aqueous environment) - Activity depend on integrity of the native protein structure. Denaturation / dissociation into subunits/ hydrolysis into a.a → lost activity → activity dependent on structure ( primary, secondary, tertiary and quaternary. - Mwt = 12,000 to million - Measurement of enzyme activities in blood plasma and tissue samples→ diagnosing Some enzymes require no chemical groups for activity other than their amino acid residues. Some require nonprotein 1) cofactors (metal ion) 2) coenzymes (organic) -Some require both. -Coenzyme / cofactor tightly and covalently bound= prosthetic group. -Enzyme + cofactor and or coenzyme = holoenzyme -Enzyme alone = apoprotein / apoenzyme Cofactors for enzymes Coenzymes Coenzymes act as transient carriers of specific functional groups. Most are derived from vitamins, organic nutrients required in small amounts in the diet. some enzyme proteins are modified covalently by phosphorylation, glycosylation, and other processes. Many of these alterations are involved in the regulation of enzyme activity any of a class of yellow water-soluble nitrogenous pigments derived from isoalloxazine and occurring in the form of nucleotides as coenzymes of flavoproteins; especially :RIBOFLAVIN any of a class of yellow water-soluble nitrogenous pigments derived from isoalloxazine and occurring in the form of nucleotides as coenzymes of flavoproteins; especially :RIBOFLAVIN Enzyme names: pharma sec 1 1) Addition of suffix “ ase” to a name describing substrate / function Urease → urea hydrolysis DNA polymerase → polymerization of DNA 2) Named after the broad function before specific function known: Pepsin = greek “ pepsis” = digestion Lysozyme = lysis of bacterial cell wall 3) Some have 2 / more names. Solution: System for naming and classification :6 classes, subclasses based on rxn catalyzed. ATP + glucose → ADP + glucose -6 p (ATP:glucose phosphotransferase) Other name hexokinase Class (transferase), subclass (phosphotransferase), phosphotransferase with hydroxyl as acceptor, glucose as phosphoryl group acceptor. Enzymes are classified according to the type of reaction they catalyze 6 classes based on rxn catalyzed. Enzyme Classification As an example, the formal systematic name of the enzyme catalyzing the reaction: ATP + D-glucose ADP + D-glucose 6-phosphate *** ATPglucose phosphotransferase: it catalyzes the transfer of a phosphoryl group from ATP to glucose. All enzymes have formal EC numbers and names. Its Enzyme Commission number (E.C. number) is 2.7.1.1. The first number (2) denotes the class name (transferase); The second number (7), the subclass (phosphotransferase); The third number (1), a phosphotransferase with a hydroxyl group as acceptor; and the fourth number (1), D-glucose as the phosphoryl group acceptor. How Enzymes Work?pharma 2 S P S P EE E E E E+S ES EP E+P E: enzyme / S: substrate / P: product ES and EP are transient complexes of the enzyme with the substrate and with the product Substrate binding to enzyme active site: Chymotrypsin with S (red). Active site: pocket of the E with a.a residues with groups that bind S Substrate: molecule/ ligand bound to active site and acted on by the E. Active site is lined with amino acid residues with substituent groups that bind the substrate and catalyze its chemical transformation Enzyme Catalysis What is the function of the catalyst? to increase the rate of a reaction. The role of enzymes is to accelerate the inter conversion of S (Substrate) and P (product). Not effect on the both concentrations. Characteristics: 1. do not affect reaction equilibria. 2. The enzyme is not used up in the process, and the equilibrium point is unaffected. 3. The reaction reaches equilibrium much faster when the appropriate enzyme is present, because the rate of the reaction is increased. Decrease the time that needed for particular reaction Energy barrier between S and P: required for Alignment of reacting groups, formation of transient unstable charges, bond rearrangements For the reaction to occur: must overcome this barrier and therefore must be raised to a higher Parially breakdown, partially formed energy level. The highest possible free energy energy hill At the top of the energy hill is a point called the transition state: where the bond is starting to form and the geometry is changing. Activation energy (bond breakage, bond formation, and charge A-B.C development, ) have proceeded to the precise point at which decay to either substrate or product is equally likely. A+B-C (The difference between the energy levels of the ground state and the transition state is the activation energy, G‡) The rate of a reaction reflects the activation energy Higher activation energy → slower rxn In cells, enzymes and other catalysts lower the activation energy of a rxn →enhance rxn rate. accelerate interconversion of S and P - E not used up in the rxn Equilibrium constant Keq = K = [P] / [S] ∆G`° = large and –ve → favorable rxn. the equilibrium constant is directly related to the overall standard free energy change for the reaction (Table 6–4). A large negative value for G reflects a favorable reaction equilibrium—but as already noted, this does not mean the reaction will proceed at a rapid rate Reaction equilibria are linked to the standard free-energy change for the reaction ∆G° Reaction rates are linked to the activation energy, ∆G‡ Exergonic reaction will be spontaneous, negative ∆G° Endergonic reaction will not be spontaneous and will need energy to do, positive ∆G° https://www.youtube.com/watch?v=tPCOEUo6J8s Sucrose C12H22O11 + O2 → 12CO2 + 11 H2O ∆G`° large and –ve In vitro ( in container) : no rxn In vivo : rxn + useful form of energy ATP E overcomes the E barrier , important for control in metabolism. Reaction intermediate: S→ ES→EP→P Rxn with several steps: Step/s with highest activation energy = highest energy point in the diagram of S→P interconversion = rate limiting step. E: enzyme / S: substrate / P: product ES and EP are transient complexes of the enzyme with the substrate and with the product The function of a catalyst is to increase the rate of a reaction Any reaction may have several steps, involving the formation and decay of transient chemical species called reaction intermediates. - Enzymes are highly effective catalyst enhancing rxn rates by a factor of 105 -1017 Rate is described by determining how quickly the reactant are consumed or the product is formed. Rate equation Rate / velocity V = k[S] , k= rate constant, unit : reciprocal time: s-1 reflects probability of rxn in a given temp, pH, etc… Order of reaction describes how the rate of reaction depend on conc. The rate of any reaction is determined by the concentration of the reactant (or reactants) and by a rate constant (constant not depend on concentration). S → P , 1st order rxn If a first-order reaction has a rate constant k of 0.04 (mean that 4% of the available S will be converted to P in 1 s) A reaction with a rate constant of 2,000 s1 will be over in a small fraction of a second V = k[S] S1 + S2 → P , 2nd order rxn If a reaction rate depends on the concentration of two different compounds, or if the reaction is between two molecules of the same compound, the reaction is second order and k is a second-order rate constant, with units of M1s1. V = k[S1] [S2] , k = M-1s-1 Now we turn from what enzymes do to how they do it? Catalytic power and specificity of enzymes: 1) Transient covalent interactions bw S and catalytic site of E mainly functional groups of some a.a, metal ion or coenzyme. Rearrangement of bonds/ transfer of a functional group to E Covalent interactions lower the activation energy by providing an alternative, lower-energy reaction path 2) Non covalent interactions bw E and S ( hydrophobic / ionic / H-bonds) Formation of weak interaction in the ES complex is accompanied by release of a small amount of free energy that provides a degree of stability to the interaction Each bond formation associated with energy release→ stabilize transition state. Free energy released in forming bonds + interaction bw E and S = binding energy = ∆GB Binding energy is a major source of free energy used by enzymes to lower the activation energies of reactions How does an enzyme use binding energy to lower the activation energy for a reaction? Enzyme specificity : pharma sec 1 Monday 27.11 1) Key and Lock model : ( misleading ) An enzyme completely complementary to its substrate would be a very poor enzyme. Emil Fischer → E structurally complementary to S Fit in like key in lock Does not explain how product released or the rate Enzyme is dynamic not rigid 2) Induced fit : Active site not 100% complementary to S but to transition states. An imaginary enzyme (stickase) designed to catalyze breakage of a metal stick ES complex is more stable and has less free energy in the ground state than substrate alone. The result is an increase in the activation energy. No change in E or substrate shape enzyme must be complementary to the reaction transition state More acceptable model. Involve parts of the stick far away from bent site stabilize + Changes in shape away from water. Explain why E are large? Weak Interactions between Enzyme and Substrate Are Optimized in the Transition State pharma sec 2 Saturday Role of binding energy is catalysis: activation energy for a rxn lowered by binding energy bw E and S weak noncovalent interactions in transition state. The summation of the unfavorable (positive) activation energy G‡ and the favorable (negative) binding energy GB results in a lower net activation energy *: acquire an amount of energy equivalent to the amount by which activation energy is lowered. Binding energy explains the need for large enzymes, and account for specificity. An enzyme must provide functional groups for ionic, hydrogen-bond, and other interactions, and also must precisely position these groups so that binding energy is optimized in the transition state Once a substrate is bound to an enzyme, functional groups aid in the cleavage and formation of bonds by a variety of mechanisms including: 1. acid-base catalysis 2. covalent catalysis 3. metal ion catalysis. Several mechanisms of catalysis: Acid- base catalysis: (general not specific –proton transfer involve molecules other than water) In E active site several a.a serve as proton donors / acceptors (most common catalysis type). Proton transfer provide rate enhancement of 102 – 105 Covalent catalysis: Transient covalent bond formed bw S and E A-B →(H2O)→ A+B (hydrolysis of the bond) Enzyme with nucleophile X: electron-rich atom A-B + X: → A-X + B →(H2O)→ A + X: +B functional groups of some enzyme cofactors can serve as nucleophiles in the formation of covalent bonds with substrates Metal ion catalysis: weak bonding interactions between metal and substrate Ionic interactions bw E-bound metal + S→ 1) Orient S /or stabilize transition state. 2) Metals mediate redox rxns by reversible changes in metals oxidation state. 1/3 E require one or more metal ions for catalysis. Most E employ more than one catalysis rxn Covalent and general acid-base catalysis: 1st step in rxn is acylation step. (cleavage of peptide bond) Hydroxyl group of Ser195 is the nucleophile. (formation of a covalent linkage) Catalytic triad in enzyme here hydroxyl group of Ser195 is the nucleophile His 57 Covalent linkage bw E and part of S the base is the side chain of His57 H+ electron- deficient atom (an electrophile). Break peptide bond OH in ser is not strong nucleophile so the H will be removed by His N atom, that were oriented in the correct position by the attraction with Asp. i.e stabilize the histidine so it can carry the protonation, and convert Ser to more nuleophile by converting OH to alcoxide that attack the carbon of the carbonyl group. So break down of peptide bond. https://www.youtube.com/watch?v=OY1WsqlcUdo Enzyme kinetics Dentist Sunday 2023 Most enzymes have certain kinetic properties in common Rate of rxn and the effect of [S] Initial rate = initial velocity = V° Effect of [S] on the initial velocity of an E – catalyzed rxn: sec 1 Michaelis constant = Km = [S] where Vo is half maximal. The substrate concentration at which V0 is half Vmax Hyperbolic (all E except regulatory) Plateau like V Saturation kinetics At low [S] → Vo ↑ linearly steady state, [ES] constant over time with [S]. V0 increases by smaller and At higher [S]→ Vo ↑ by smaller smaller amounts in response to increases in [S] amounts. Pre-steady state, E free, [ES] builds up micro seconds E saturated with S [S] but effect on V is smaller. The [S] that results in a reaction rate = 1/2 Vmax is the Michaelis constant Km which is characteristic for each enzyme acting on a given substrate. The Michaelis-Menten equation Vo = Vmax [S] Km +[S] Relates Vo to [S] and Vmax through the constant Km. Michaelis- Menten kinetics is also called steady-state kinetics. From Equation we can get information………….. !Hyperbolic curve Michaelis-Menten Equation The important of measuring the V0 because at the beginning of a reaction the changes in [S] are negligible, so [S] can be treated as a constant. The rate equation for a one-substrate enzyme-catalyzed reaction. It is a statement of the quantitative relationship between the initial velocity V0, the maximum velocity Vmax, and the initial substrate concentration [S], all related through the Michaelis constant Km. Km has units of concentration. The reaction quickly achieves a steady state in which [ES] (and the concentrations of any other intermediates) remains approximately constant over time. V0 Substrate concentration (mmol/min) (mM) ————————————— 2 1 12 5 25 11 35 50 44 200 48 500 V0= K2 [ES] V0 (Formation of [ES]) =K1[E][S] = K1[ET-ES][S]...(2) V0 (breakdown of [ES]) =K-1[ES] + K2[ES]……(3) In steady state [ES] is constant: (2) = (3): K1[ET-ES][S] = K-1[ES] + K2[ES] K1[ET][S]- K1[ES][S] = K-1[ES] + K2[ES] K1[ET][S]= K1[ES][S]+[ES][K-1+K2] =[ES] [K1[S]+[K-1+K2]] [ES] = K1[ET][S] [ET][S] [ET][S] K1[S]+[K-1+K2] [S]+[K-1+K2]/K1 [S]+Km V0 = K2[ET][S] V0 = Vmax [S] [S]+Km Km + [S] Interpreting Vmax and KM V0 = Vmax [S] Vmax = Vmax [S] KM + [S] 2 KM + [S] 1 = [S] 2 KM + [S] Solving for KM, we get: KM + [S] = 2[S], OR KM = [S], when V0 =1 Vmax 2 Michaelis - Menten equation / rate equation: Dependence of initial velocity Vo on [S] The equation Vo = Vmax [S] Km +[S] Vo = 1/2 Vmax Vmax = Vmax [S] 2 Km + [S] Km= [S] Equation and Km provide little information about chemical nature/ steps of the rxn. Importance of Km in enzyme reactions The Michaelis constant (Km) is a means of characterizing an enzyme's affinity for a substrate. The Km in an enzymatic reaction is the substrate concentration at which the reaction rate is half its maximum speed. A low Km value means: the enzyme has a high affinity for the substrate (as a "little" substrate is enough to run the reaction at half its max speed). This is only true for reactions where substrate is limiting and the enzyme is NOT allosteric. Km indicator of the affinity of E to S. Vary from E to another and from different S for the same E E with low [S] in cell lower Km than E with abundant S (the lower Km the less substrate needed to form ES complex Michaelis-Menten Equation dentist-Tuesday Lineweaver–Burk Plot V0 = Vmax [S] KM + [S] Double reciprocal plot or Lineweaver-Burk plot: (more convenient) The Michaelis-Menten equation Vo = Vmax [S] Km+[S] 1 = Km+[S] Vo Vmax [S] Important in analyzing E inhibition. Advantage: precisely determining Vmax Kcat = most of the enzyme is in the EP form at saturation, and Vmax = k3[Et]. describes the limiting rate of E – catalyzed rxn at saturation. kcat = Vmax/[Et]. The concentration of enzyme is necessary. Kcat = turnover number = tell us the maximum number of S molecules → P in a unit time on a single active site of E molecule when E saturated with S EX:A single active site converts 40,000,000 substrates to product per sec Taking the reciprocal of kcat(1/kcat: 1/40.000,000) give the time that is needed for one substrate to convert to product. Km: describes the amount of S needed for the E to obtain half of its maximum rate of reaction. km can be separated into two parts: 1) kd The first step of catalysis kinetic is the binding between S and E ( rate determine step in the reaction). the better enzyme bind to substrate, the smaller kd = smaller kM 2) kcat The second step of catalysis kinetic is the forming of P. The larger kcat is, the more favorable the reaction towards P Kcat and Km for evaluation of kinetic efficiency of enzymes Kcat = specificity constant Km kcat/KM = rate constant that measures catalytic efficiency. helpful in determining whether the rate is limited by the creation of product or the amount of substrate in the environment. if the rate of efficiency is: HIGH (kcat is much larger than KM) and the enzyme complex converts a greater proportion of the substrate it binds into product. (substrate binds more firmly (strong) to the enzyme, a consequence of relatively low KM ) or a large turnover rate kcat. LOW (kcat is much smaller than KM ) and the complex converts a lesser proportion of the substrate it binds into product Enzymatic rxn with 2 or more S : ATP + glucose →hexokinase→ glucose-6-p + ADP -Analyzed by M-M equation and M-M kinetics with Km for each S. -Involve transfer of a functional group/ atom from one S to another. Bi-substrate Reaction 1)Sequential binding of S1 and S2 before catalysts: A. Random substrate binding: either S1 or S2 can bind first, then the other binds. B. Ordered substrate binding: S1 must bind before S2. 2)Ping Pong reaction: first S1 give P1, then P1 released before S2 bind, then S2 give P2. Common mechanisms for rxn with more than one S: 1) E and both S form a ternary complex. In random binding substrates can bind in either order. In ordered binding S1 must bind before S2 can bind productively. 2) Ping-pong or double-displacement mechanism: E-S complex forms a P1 leaves the complex. Altered E forms a 2nd complex with another S, and P2 leaves, regenerating E S1 transfer a functional group to E to form covalently modified E’ which is transferred to S2. Steady-state kinetic analysis of bisubstrate rxns: In double-reciprocal plots, S1 conc is varied while the S2 conc is held constant. Intersecting lines indicate formation of ternary complex. Parallel lines indicate a ping-pong /double displacement pathway. ternary complex ping-pong Enzyme inhibition: sec 1 and 2 next saturday inhibitors: agents that interfere with catalysis slowing / halting enzymatic rxns. 1) Pharmaceutical agents: e.g aspirin (acetylsalicylate) inhibits prostaglandins synthesis (pain processes). 2) Discovery and define of metabolic pathway. Inhibition is reversible / irreversible Three types of reversible inhibition: 1) Competitive inhibitors: Inhibitor resembles S and bind E → EI complex but no catalysis / P αKm↑= “apparent”/ observed in I presence. ◼ Slope is larger (multiplied by a) Intercept does not change (Vmax is the same) KM is larger (multiplied by a) Solution: Add more S [S]>>[I] Competitive Inhibitor ◼ Most common ◼ Inhibitor competes with natural substrate for binding to active site ◼ Inhibitor similar in structure to natural substrate and binds active site of enzyme (reducing effective enzyme conc) ◼ Binds more strongly ◼ May or may not react ◼ If reacts, does so very slowly ◼ Gives info about active site through comparison of structures Reversible Inhibition (competitive) Vmax +Inh 1/v -Inh +inh vo -Inh 1/2 Vmax 1/Vmax Km [Substrate] 1/[S] -1/Km α α Inhibitor competes with substrates for binding to active site Inhibitor is similar in structure to substrate binds more strongly reacts more slowly Increasing [I] increases [EI] and reduces [E] that is available for substrate binding Need to constantly keep [I] high for effective inhibition (cannot be metabolized away in body) ◼ Slope is larger (multiplied by a) ◼ Intercept does not change (Vmax is the same) ◼ KM is larger (multiplied by a) Use of competitive inhibitor in Medical therapy: Case: methanol poisoning usually from contaminated alcohol beverages Methanol in liver → alcohol dehydrogenase (EC 1.1.1.1) → formaldehyde → formic acid→ metabolic acidosis Symptoms: Vomiting, abdominal pain, photophobia, tissue damage Ingestion of 10ml →blindness , 30ml → fatal (2 tablespoons deadly to a child). Treatment: Antidote مضاد سميto reverse the effect of the poison intravenous infusion of ethanol = competitive inhibitor to alcohol dehydrogenase. The dehydrogenase enzymes facilitate the interconversion between alcohols and aldehydes or ketones with the. of nicotinamide adenine dinucleotide (NAD+ to NADH) reduction CH3OH + NAD+ CHO + NADH +H+ ethanol CH3CH2OH + NAD+ → CH3CHO + NADH + H+ methanol Uncompetitive inhibitors: Sunday dentist Bind at a separate site, but bind only to the ES complex ( after S) α Km ↓ and Vmax ↓ Cyanide combines with the prosthetic group of cytochromo oxidase and inhibits the election transport chain 1. Binds only to ES complex but not free enzyme 2. Binds at location other than active site 3. Does not look like substrate. Binding of inhibitor distorts active site thus preventing substrate binding and catalysis 4. Cannot be competed away by increasing conc of substrate (Vmax is affected by [I]) Increasing [I] lowers Vmax and lowers Km. ◼ Increasing [I] ◼ Lowers Vmax (y-intercept Uncompetitive Inhibitor increases) ◼ Lowers KM (x-intercept decreases) ◼ Ratio of KM/Vmax is the same (slope) Vmax -Inh Vmax (app)_ +inh vo 1/2 Vmax 1/2 Vmax ןKm Km [Substrate] Mixed inhibitors: Also bind to a site separate than the active site, but may bind to either E or ES. Inhibitor binds E or ES ◼ Increasing [I] ◼ Lowers Vmax (y-intercept increases) ◼ Raises KM (x-intercept increases) ◼ Ratio of KM/Vmax is not the same (slope changes) If the inhibitor may bind to the enzyme whether or not the substrate has already been bound, but has a higher affinity for binding the enzyme in one state or the other, it is called a mixed inhibitor. Mixed-type inhibitors bind to both E and ES, but their affinities for these two forms of the enzyme are different. Reversible Inhibition (non-competitive) A inhibitor binds the enzyme but not in its active site. Rate of catalysis is affected. Non-competitive inhibition is a type of enzyme inhibition where the inhibitor reduces the activity of the enzyme and binds equally well to the enzyme whether or not it has already bound the substrate. Non-competitive inhibitors have identical affinities for E and ES. i.e: non-competitive inhibition can occur with or without the substrate +Inh present. Vmax 1/v -Inh Vmax 1/Vmax -Inh (app)_ +inh (app) vo 1/2 Vmax 1/2 Vmax 1/Vmax (app) -1/Km 1/[S] Km Km [Substrate] (app) Vmax is decreased proportional to inhibitor conc It not afffect km 1/v +Inh -Inh -1/Km 1/[S] Effects of reversible inhibitors on Km and Vmax Inhibition Binding Km Vmax Km/Vma x Competitive E Uncompetitive ES Mixed ES, E Noncompetitive E Irreversible inhibition: dentist Binds irreversibly to E active site by forming a covalent bond/ very stable non covalent interaction → destroy a functional group. diisopropylflourophosphte (DIFP): irreversibly inhibits chymotrypsin Ser195 is the key active site residue in chymotrypsin. Suicide inactivators / mechanism-based inactivators: pharma sec-1 monday Relatively unreactive until binding to E active site. Perform first few chemical steps of rxn→ not transformed to normal product. Inactivator is converted to a very reactive compound that combines irreversibly with the enzyme Penicillin which inhibits transpeptidase from building bacterial cell walls. Exemestane, a drug used in the treatment of breast cancer, is an inhibitor of the aromatase enzyme. Important in DRUG DESIGN: Specific for a single E unreactive until within E active site. Advantage: few side effects The pH activity profiles of two enzymes: Optimum pH is close to the pH of the environment in which the enzyme is normally found. Optimum pH→ maximum activity. Gastric juice pH = 1 - 2 pH of hepatocyte cytosol = 7.2 Regulatory Enzymes: - One / more E in a metabolic pathway have great effect on the rate of the overall rxn. - Activity of allosteric E ( increase /decrease) regulated by reversible binding of a specific modulator to a regulatory site other than active site. Effect of modulator = positive / negative. Modulators maybe the S itself (Homotropic modulator) / other metabolites. Kinetic behavior of allosteric E reflects cooperative interactions among E subunits ( similar to O2 – hemoglobin). Regulatory Enzymes: Allosteric E regulated by reversible noncovalent binding of modulators / effectors. Other E regulated by reversible covalent modification. - All are (Multisubunit proteins). Structural allosteric have 1 or more allosteric sites each with a site specific to modulator. Larger and more complex e.g. asparate transcarbomylase. Regulatory enzyme aspartate transcarbamoylase (nucleotide synthesis): 12 polypeptide chains 2 catalytic clusters. Each with 3 polypeptide catalytic chains (blue + purple). 3 regulatory clusters. Each with 2 polypeptide regulatory chains (red + yellow). Regulatory clusters form the points of a triangle surrounding catalytic subunits. Subunit interactions in an allosteric E, interactions with inhibitors and activators: S binding site and M sites on different subunits. Catalytic regulatory Binding of M to R →induce conformational change in C →active and capable of binding S with higher affinity. When M dissociates. Enzyme→ inactive/ less active. In most multienzyme systems, the first enzyme of the sequence is a regulatory enzyme Feedback inhibition = - The regulatory E in a metabolic pathway is inhibited by the end product of the pathway. - Build up of end product slows entire pathway Heterotropic allosteric inhibition = Noncovalent + reversible. Pathway inhibited allostericaly by L-isoleucine. But not by any of the 4 intermediate. Kinetics of allosteric enzymes: Differ than M-M kinetics. Sigmoid saturation curve not hyperbolic. Reflects cooperative interaction bw subunits. reaction catalyzed by an allosteric enzyme Small changes in [S] Large effect on activity K0.5 not Km The sigmoid curve of a homotropic enzyme Sigmoid curve for allosteric enzymes: An activator cause the curve to become → more hyperbolic. K0.5 decrease, no change in Vmax and large change in activity. An inhibitor cause the curve to become → more sigmoid activator Resulting in an increased reaction velocity at a fixed inhibitor substrate concentration (V0 is higher for any value of [S] Ex: competitive inhibitor A less common type of modulation, in which Vmax is altered and K0.5 is nearly constant Covalent modifications (reversible) of regulatory enzymes by a functional group CARBOHYDRATES Ch-7 1) Energy: CHO oxidation central energy–yielding pathway. 2) Structural and protective elements: insoluble polymers in cells walls of bacteria, connective tissue of animals, plants (cellulose) 3) participate in biological transport, Adhesion and cell-cell recognition, modulation of the immune system 4) intermediates in the biosynthesis of other basic biochemical entities (fats and proteins) 5) Determine fate/ intracellular location of hybrid molecules / signal Glycoconjugates = complex CHO covalently attached to lipids/ proteins Sugars (saccharides) are compounds containing an aldehyde or ketone group and two or more hydroxyl groups.may contain sulfur, nitrogen, phosphorous e.g. in deoxysugars, aminosugars. (CH2O)n , Some exceptions exist; Ex, deoxyribose,sugar in DNA, (formula C5H10O4) Three major classes: 1)Monosaccharides / simple sugars: (-ose) single polyhydroxy aldehyde / ketone unit. most abundant = D-glucose > 4C → cyclic structure 2) Oligosaccharides: (-ose) chain of mono joined by glycosidic bond most abundant disaccharides e.g. sucrose= glucose+fructose In cells oligo >3 mono don’t occur free but joined to lipid/protein 3) Polysaccharides: >20 till hundreds/ thousands mono. linear chain (cellulose) or branched (glycogen) Monosaccharides: (simple sugars) medical Colorless, crystalline solids, soluble in water, sweet taste. ❑ Monosaccharides are classified according to three different characteristics: 1. the placement of its carbonyl group 2. the number of carbon atoms it contains, 3. its chiral handedness. Are either aldehydes or ketones with two or more hydroxyl groups. Trioses, tetroses, pentoses, hexoses, heptoses Many C atoms are chiral centers→ stereoisomers. Two families of Monosaccharides: Aldoses: if carbonyl group at an end of the C chain. Ketoses: if carbonyl group at any other position of the C chain Simplest Monosaccharides: Glycerol is the parent Sugar are numbered beginning at the end of the chain nearest the carbonyl group Hexoses: Most common mono in nature Pentoses: Components of nucleotides and nucleic acids. D-Glucose 1.an aldohexose with the formula (CH2O)6. 2. The red atoms highlight the aldehyde group, and the blue atoms highlight the asymmetric center furthest from the aldehyde. By convention: Two isomers D- and L- OH- group on the reference C on the right = D-isomer. OH- group on the reference C on the left = L-isomer. All mono except DHAP has one/more asymmetric chiral centers. Simplest = glyceraldehyde One chiral center (middle C) →2 different isomers / enantiomers → Mirror images. Sterioisomers Divided into 2 groups (D , L) that differ in configuration about chiral center most distant from the carbonyl carbon = REFERENCE Carbon. Molecule with n chiral centers have 2n sterioisomers. Glyceraldehyde 21 = 2 Aldohexose = 24 = 16 16 possible aldohexoses, eight are D forms and eight are L. Most of the hexoses of living organisms are D isomers.. Ketoses: Four- and five- carbon ketoses has the suffix – “ul” D-ribulose is the ketopentose --------→ aldopentose D-ribose Ketohexoses named differently: Aldoses: Carbon numbering : begin at the end of the chain nearest to carbonyl group C = chiral center straight-chain molecule Some sugars exist in nature as L- isomers e.g L-arabinose and components of glycoconjugates. Epimers : Two sugars that differ only in configuration of one chiral center. Epimers of D- glucose: Hemiacetal & hemiketal formation. An aldehyde can react with an alcohol → hemiacetal. A ketone can react with an alcohol → hemiketal. Nucleophilic new chiral center at the carbonyl carbon attack by OH Substitution of another alcohol (another sugar)→Glycosidic bond Hemiacetals and hemiketals, hèmi means half, are compounds that are derived from aldehydes and ketones respectively. These compounds are formed by formal addition of an alcohol to the carbonyl group. When the alcohol group is replaced by a second alkoxy group, an acetal or a ketal, respectively, is formed Mono >4C in aqueous soln → Glucose: Exists in Ex: Glucose can exist in both a straight-chain and ring form solution as an intramolecular The formation of these ring structures is the result of a hemiacetal general reaction between alcohols and aldehydes or ketones to form derivatives called hemiacetals or hemiketals Oxygen of the hydroxyl of Anomers: reference C5 attack Carbonyl Isomeric forms of mono differ carbon (aldehyde /ketone) only in configuration at hemiketal/ hemiacetal C six-membered ring called pyranoses New bond Mutarotation: Hemiacetal linkage Interconversion bw 2 anomers (α and β) of hemiacetal/ hemiketal Trans to CH2OH Cis to CH2OH Alpha below Beta above C1 hemiacetal (or carbonyl) carbon = anomeric carbon α / β Two stereoisomers Ketohexoses also occur in alpha and beta anomeric forms. In these compounds the hydroxyl group at C-5 (or C-6) reacts with the keto group at C-2, forming a furanose ring containing a hemiketal linkage 5 O O 4 1 4 1 3 2 3 2 Pyran Furan ❑ How often is D glucose found in a cyclic form? one-third 1/3(37%) alpha-D-glucose, two-thirds 2/3 (63%) beta-D-glucose, and very small amounts (