Protein Function PDF
Document Details
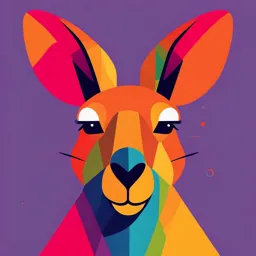
Uploaded by AwesomeSerpentine3604
Temple University
Marc A. Ilies, Ph. D.
Tags
Summary
This document explains protein function, focusing on the interactions of proteins with other molecules. It discusses ligand binding, cooperativity, and the roles of myoglobin and hemoglobin in oxygen transport. It also touches on the effects of pH and other factors.
Full Transcript
Protein Function Marc A. Ilies, Ph. D. Lehninger - Chapter 5 (p157-186) [email protected]; lab 517, office 517A (Tu, Fr 3-5) For questions, comments please use the discussion tool in Canvas or recitation session ©MAIlies...
Protein Function Marc A. Ilies, Ph. D. Lehninger - Chapter 5 (p157-186) [email protected]; lab 517, office 517A (Tu, Fr 3-5) For questions, comments please use the discussion tool in Canvas or recitation session ©MAIlies2024 1 Protein Function: Globular Proteins Proteins function by interacting with other molecules Outcome of interactions: Recognition/binding → transport/signaling/immune responses Biochemical reaction → enzymes Key topics in protein function: A molecule bound reversibly by a protein is called a ligand A ligand binds the protein at a specific site called binding site, which is complementary to the ligand in size, shape, charge, hydrophilic or hydrophobic character Reversible binding of ligands is essential – Specificity of ligands and binding sites – Ligand binding is often coupled to conformational changes, sometimes quite dramatic (Induced Fit) – In multisubunit proteins, conformational changes in one subunit can affect the others (Cooperativity) – Interactions can be regulated Illustrated by: – Hemoglobin, antibodies Reversible ligand binding: O2 binding and transport by myoglobin and hemoglobin O2 is nonpolar, weakly soluble in water cannot be carried dissolved in large amounts O2 is bound by an Fe2+ ion, chelated in a heme prosthetic group: Fe2+ (ferrous) + protoporphyrin = heme Porphyrin is a cyclic, conjugated tetrapyrrole ring (flat, planar, aromatic) Fe2+ is hexacoordinated (4 N from protoporphyrin, 1 N from 3 proximal His, 1 e- pair from O2, in an octahedral geometry: Reversible ligand binding: O2 binding and transport by myoglobin and hemoglobin Ligand positioning is essential: O2 can react with Fe2+ ion, in the presence of water, transforming it into Fe3+ (ferric) ion which cannot bind oxygen → heme prosthetic group is positioned (buried) in a hydrophobic environment created by nonpolar residues of α-helices MY O2 is accommodated in an amphiphilic cavity formed by Val, distal His, and Phe AA residues, in a bent conformation binding of O2 changes the heme color from dark red/purple to bright red (venous blood/arterial blood) Other small ligands such as CO, CN-, etc can be accommodated by this amphiphilic cavity and can bind Fe2+ ion → poisons! 4 Oxygen Binding to Myoglobin Globins: a family of proteins specialized in sensing and transport of gasses O2, NO, etc in different organisms (eukariotes, bacteria); - in humans, 4 globins: myoglobin (O2 binding, monomeric, in muscles), hemoglobin (O2 binding, tetrameric, in blood), neuroglobin (O2 binding, in neurons), cytoglobin (NO binding, in walls of blood vessels) Myoglobin is monomeric, has one O2 binding site 153 AA, MW = 16.7 KDa 78% α-helix structure, 8 α-helical segments (A-H) Proximal His (His-93), coordinates (binds) Fe2+ in heme Distal His (His-64) helps accommodate the O2 molecule Quantitative Protein – Ligand Interactions P+ L PL K a = [PL] A measure of L affinity for P It is more common and simpler to use the [P] [L] the >Ka > affinity (usually [L] >># of dissociation constant Kd which is the reciprocal binding sites so conc of free L of Ka (Kd=1/Ka). The units here are in molar K a[L] = [PL] remains constant). concentration (M). Kd is the equilibrium [P] constant for the release of ligand (L). The equations Fraction of binding sites occupied change to q= binding sites occupied [PL] K d = [P][L] = total binding sites [PL] + [P] [PL] [P][L] Substituting K a[L][P] for [PL] and re-arranging terms yields [PL] = Kd [L] [L] q= K a[L][P] = Ka[L] = q= K a[L][P] + [P] Ka[L]+1 [L] + 1 [L] + K d Ka x= y/(y+ z) is the eqation for a hyperbola, thus the graph of qvs L Kd represents affinity of P for L. looks like this The lower the Kd the higher the affinity. Kd is equivalent to the molar conc of L at which half of the available binding sites are occupied. At this point the P is said to have reached half saturation with respect to ligand binding. heme The more tightly a P binds to an L the → oxyter lower the conc of L required for half the [L] at which half of the available ligand-binding sites sites to be occupied and the lower Kd. are occupied (at =0.5) corresponds to 1/Ka 6 Quantitative Protein – Ligand Interactions When ligand is a gas, binding is expressed in terms of partial pressures (pO2): [L] pO2 q= q= K d + [L] p50 + pO2 P50 – partial pressure of O2 at which 50% of the available sites are occupied – similar significance as KD O2 binds tightly to myoglobin, with a P50 = 0.26 kPa 7 TABLE 5-1 Protein Dissociation Constants: Some Examples and Range Protein Ligand Kd(M) a Avidin (egg white) Biotin 1 x 10–15 Insulin receptor (human) Insulin 1 x 10–10 Anti-HIV immunoglobulin (human)b gp41 (HIV-1 surface protein) 4 x 10–10 Nickel-binding protein (E. coli) Ni 2+ 1 x 10–7 Calmodulin (rat)c Ca2+ 3 x 10–6 2 x 10–5 Color bars indicate the range of dissociation constants typical of various classes of interactions in biological systems. A few interactions, such as that between the protein avidin and the enzyme cofactor biotin, fall outside the normal ranges. The avidin- biotin interaction is so tight it may be considered irreversible. Sequence-specific protein- DNA interactions reflect proteins that bind to a particular sequence of nucleotides in DNA, as opposed to general binding to any DNA site. aA reported dissociation constant is valid only for the particular solution conditions under which it was measured. Kd values for a protein-ligand interaction can be altered, sometimes by several orders of magnitude, by changes in the solution’s salt concentration, pH, or other variables. bThis immunoglobulin was isolated as part of an effort to develop a vaccine against HIV. Immunoglobulins (described later in the chapter) are highly variable, and the Kd reported here should not be considered characteristic of all immunoglobulins. cCalmodulin has four binding sites for calcium. The values shown reflect the highest- and lowest-affinity binding sites observed in one set of measurements. 8 Specificity: Lock-and-Key Model Proteins typically have high specificity: only certain ligands bind High specificity can be explained by the complementary of the binding site and the ligand. Complementary in – size, – shape, – charge, – or hydrophobic/hydrophilic character “Lock and Key” model by Emil Fisher (1894) assumes that complementary surfaces are preformed. + Specificity: Induced Fit Conformational changes may occur upon ligand binding (Daniel Koshland in 1958) – This adaptation is called the induced fit – Induced fit allows for tighter binding of the ligand – Induced fit allows for high affinity for different ligands Both the ligand and the protein can change their conformations + Protein structure affects how the ligands bind Carbon monoxide (CO) binds 20000 x tighter than O2 the free heme: Carbon monoxide (CO) binds only 40 x tighter than O2 the heme in myoglobin: Distal His = His64, does not bind directly Fe2+, but helps stabilize the O2 binding to Fe2+ O2 binding to heme in myoglobin Proximal His = His93, binds directly the Fe2+ 11 Carbon monoxide competes with O2 for metal binding CO has similar size and shape to O2; it can fit to the same binding site CO binds over 20,000 times better than O2 because the carbon in CO has a filled lone electron pair that can be donated to vacant d- orbitals on the Fe2+; CO is a much better ligand for Fe2+ than O2 Placement of heme in the myoglobin pocket decreases affinity for CO, but it still binds about 40 times better than oxygen CO is highly toxic as it competes with oxygen. It blocks the function of myoglobin, hemoglobin, and mitochondrial cytochromes that are involved in oxidative phosphorylation Hemoglobin and O2 transport Transport of O2 from lungs to tissues is ensured by hemoglobin Hemoglobin is a tetrameric protein, MW 64.5 Kda, cvasi-spherical (d ~ 5.5 nm), it contains 4 subunits, 2 α-subunits (141 AA each) and 2 β-subunits (146 AA each), structurally similar to myoglobin, each containing a prosthetic heme group: 13 https://www.youtube.com/watch?v=FaB4qbyMC1M Hemoglobin subunits interact strongly The 4 subunits interact with each other via ionic interactions between key positively charged and negatively charged AA on different subunits and via other H-bonds (not presented): 14 Hemoglobin has two main states The T state is more stable than R state when O2 is not present (T is the predominant state of deoxyhemoglobin): The binding of O2 to the T state triggers a conformation change to the R state; R state is stabilized by the presence of O2 via a shift in position of helix F; interactions between subunits changed: 15 Could myoglobin transport O2? pO2 in lungs is about 13 kPa: it sure binds oxygen well pO2 in tissues is about 4 kPa: it will not release it! Myoglobin Would lowering the affinity (P50) of myoglobin to oxygen help? For effective transport affinity of protein for O2 must vary with local pO2 Due to its structure, hemoglobin is able to change its affinity for O2 at lungs and at tissues: R state In the lungs Hb is > 90% saturated while in the tissues it is about 60% saturated note that Hb does not T state “discharge” completely its O2 load (almost never reaches the T state except under extreme hypoxic conditions)! How can affinity to oxygen change dynamically? Must be a protein with multiple binding sites Binding sites must be able to interact with each other This phenomenon is called cooperativity – positive cooperativity first binding event increases affinity at remaining sites recognized by sigmoidal binding curves T Cooperative Binding Models R T Sequential Model- Koshland MWC –Monod, Wyman, Changeux Intermediates Symmetry or concerted Model (All or None) R pH Effect on O2 Binding to Hemoglobin Actively metabolizing tissues generate H+, lowering the pH of the blood near the tissues relative to the lungs Hb Affinity for oxygen depends on the pH – H+ binds to Hb and stabilizes the T state Protonates His146 (His HC3) which then forms a salt bridge with Asp94 (Asp FG1) Leads to the release of O2 (in the tissues) The pH difference between lungs (pH = 7.6) and metabolic tissues (pH 7.2) increases efficiency of the O2 transport (the Bohr effect): In lungs (pH = 7.6, PO2 = 13 kPa, PCO2 = 4 kPa): HbH+ + O2 → HbO2 + H+ H+ + HCO3- (from plasma) CO2 (exhaled) + H2O In tisues (pH = 7.2, PO2 = 4 kPa, PCO2 = 6 kPa): CO2 (from tissues) + H2O H+ + HCO3- (in plasma) Affinity of Hb for O2 HbO2 + H+ → HbH+ + O2 decreases as pH decreases! CO2 hydration/dehydration catalyzed by carbonic anhydrase, also present in erythrocytes, together with hemoglobin Hemoglobin binds CO2 and contributes to pH effect Hemoglobin can react with CO2 and get carbamylated (at high PCO2 pressure, in tissues); release of protons during carbamylation contributes to the release of O2 with stabilization of T state: In tissues (pH = 7.2, PO2 = 4 kPa, PCO2 = 6 kPa): CO2 + Hb → HbCO2- + H+ HbO2 + H+ → HbH+ + O2 In lungs (pH = 7.6, PO2 = 13 kPa, PCO2 = 4 kPa): HbCO2- + H+ + O2 → HbO2 + CO2 Carbamylation of Hb works in tandem with Bohr effect to transport O2 and CO2 into/from the tissues 20 2,3-BPG allows for O2 release in the tissues and adaptation to changes in altitude 2,3-bisphosphoglycerate (BPG) is present in large amounts in erythrocytes; it binds at a distant site from O2 and decreases the affinity of Hb for O2: Required for adaptation of individuals to high altitude, where pO2 is lower (BPG is synthesized when individual is moved to high altitude) BPG This is a typical case of allosteric modulation of activity (allos = other (greek) + stereos = solid shape (greek) induced via binding of a small MW molecule called allosteric modulator 2,3-BPG binding stabilizes the T state of hemoglobin - a single molecule of 2,3-BPG binds to a positively charged cavity formed by the β-chains of the tetramer of deoxyhemoglobin (T state) and stabilizes it: Positively charged areas available for BPG binding DeOxyHb (T state) stabilized by 2,3-BPG binding OxyHb (R state) : Binding area for BPG not available 22 Fetal hemoglobin has a different structure, which makes it resistant to 2,3-BPG action Fetal hemoglobin HbF, has 2 regular alpha chain but 2 gamma chains instead of 2 beta ones HbF has a higher affinity for oxygen than HbA because it binds BPG weakly (chains have less + charges). The higher affinity for oxygen by HbF is a way for the fetus to extract oxygen from maternal blood supply through placenta θ HbA HbF HbA +BPG + BPG 23 pO2 Sickle cell anemia is a molecular disease of hemoglobin Sickle cell anemia occurs in individuals who inherit the allele for sickle cell hemoglobin from both parents → fewer erythrocytes, with many long, thin sickle-shaped erythrocytes Caused by a mutated version of hemoglobin – hemoglobin S (HbS); when deoxygenated HbS aggregates into polymers, fibers, which cause the sickle cell aspect of erythrocytes Caused by single AA mutation (Glu → Val) on the β-chain, which changes the regional hydrophobicity, allowing aggregation Elongated sickle erythrocytes disrupt blood flow into brain capillaries, causing anoxia, painful headaches, anemia and 24 death in childhood Sickle cell mutation of HbS allows resistance to malaria There is a natural selection that occurs with the gene for sickle hemoglobin in areas endemic for P. falciparum malaria: Sickle cell anemia is a homozygous recessive disorder. People with two genes encoding normal hemoglobin A have a significant chance of dying of acute malarial infection in childhood. In contrast, people with two genes for sickle hemoglobin are likely to succumb to sickle cell disease at an early age. However People with sickle cell trait who possess one gene for normal hemoglobin and one gene for sickle hemoglobin are more likely to survive their initial acute malarial attacks than are people with two genes for normal hemoglobin. Also, they suffer none of the morbidity and mortality of sickle cell disease. Therefore, the people with sickle cell trait (carriers) are more likely to reach reproductive age and pass their genes on to the next generation. 25 Antibody/antigen interaction and the immune response antibodies (immunoglobulins, Ig) are components of humoral “fluid” immune system that targets, recognize and bind extracellular pathogens (bacteria, viruses, or foreign proteins, other macromolecule = antigens), eliciting an immune response and keeping “memory” of past infections antibodies are proteins, secreted by B-lymphocytes; about 20% of blood proteins several types: IgA, IgD, IgE, IgG (most abundant in blood), IgM IgG has an Y shape, with two heavy chains (grey) and two light chains (blue) linked through disulfide bonds (quaternary structure), in 2 antigen-binding fragments Fab and one basal fragment, Fc: Structure of antibodies: Immunoglobulin G Two heavy chains and two light chains composed of constant domains and variable domains Light chains: one constant and one variable domain Heavy chains: three constant and one variable domain Variable domains of each chain make up the antigen-binding site (two per antibody) and are hypervariable, which confers antigen specificity. Antigens binding to antibodies causes induced fit , with significant structural changes to the antibody: Mechanism of action of antibodies Antibodies bind to fragments displayed on the surface of invading cells, viruses, etc (antigens) very strongly (KD ~ 10-10 M) Fc regions of antibodies tagging the pathogen are recognized by receptors on surface of macrophages (large phagocytic cells) and trigger phagocytosis and subsequent destruction of foreign fragment: Antibody specificity is exploited in the use of antibodies as analytical reagents to quantify different biological macromolecules Enzyme-linked immunosorbent assay ELISA allows rapid screen and quantification of an antigen in a complex (e.g. biological) sample: (can be automated for high throughput screening) Immunoblot assay (Western blot), allows the identification of a protein in a complex sample: Goals and Objectives Upon completion of this lecture at minimum you should be able to answer the following: ► How protein function and what is the outcome of their interactions with other biochemical entities; ► What is a ligand, a binding site, induced fit, cooperativity in binding ► How oxygen molecule is bound in heme, what advantages are brought by proper positioning of the ligands in myoglobin and hemoglobin ► How can one quantitate the protein-ligand interactions, what is Ka, Kd, θ, and how are these parameters related in the binding of oxygen by myoglobin and hemoglobin ► Which are the ligand binding models in proteins, how structure affects ligand binding for myoglobin and hemoglobin (with examples) ► What is the structure of hemoglobin, structural particularities that makes it different from myoglobin, states of hemoglobin and cooperative binding of oxygen (with models), what is the pH effect (Bohr effect) on oxygen binding by Hb, carbamylation of Hb, what is 2,3BPG, where is important and how it interacts with Hb, what are the properties of fetal hemoglobin, what cause sickle cell anemia, what is its impact on human health and resistance to malaria ► What are antibodies, what types of antibodies we distinguish, what is the structure of a typical IgG antibody, what is their mechanism of action in vivo and where one can use the specificity of antibodies in biomedical screening 30