Health Sciences 1CC6 Super 7 Lists PDF
Document Details
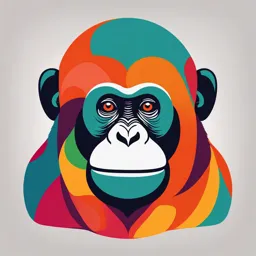
Uploaded by TransparentLemur
Brant Community Healthcare System
OCR
Tags
Summary
This document from the OCR exam board outlines topics in cell biology, including cell physiology, membrane properties, and a review of the functions of various cellular organelles. It appears to be a study guide or list of frequently asked questions for health science students.
Full Transcript
Health Sciences 1CC6 Super 7 Lists Cell Physiology 1. Briefly review the building blocks for the cell (atoms, elements, compounds, macromolecules) - Atoms combine to form -> molecules like water and protein to form -> organelles (basic components of cells) -> cells (smallest unit of living things) -...
Health Sciences 1CC6 Super 7 Lists Cell Physiology 1. Briefly review the building blocks for the cell (atoms, elements, compounds, macromolecules) - Atoms combine to form -> molecules like water and protein to form -> organelles (basic components of cells) -> cells (smallest unit of living things) -> tissues -> organs -> organ systems -> organism 2. Review structure and function of plasma membrane properties Plasma Membrane Property Structure • Bilayer forming the basic outer shell of the membrane. Contains mostly phospholipids and small amount of cholesterol • Phospholipids have a hydrophilic head that attracts Lipids water and a hydrophobic body that tail that prevents water soluble substances from crossing the membrane • Cholesterol wedges between the hydrophobic tails and stiffens the membrane • Transport communication and determine the functions that the membrane performs • Proteins can have different shapes and have different functions Proteins • Some float along the membrane and others are Integrated transmembrane or peripheral tethered to the membrane • Act as receptors, mediating transport, acting as enzymes, and attaching cells to each other and the extracellular matrix • Act as “identity molecules”, allowing cells to recognize who is who (sorting into tissues and organs); and allow immune cells to recognize bodies own cells from a pathogen Carbohydrates • Located only on the outer surface of the membrane • Can attach to proteins (glycoproteins) or lipids (glycolipids) Plasma Membrane Function Physical Barrier • Encloses the cell and separates cytoplasm from extracellular fluid Selective permeability • Determines which substances can enter or exit the cell Communication • Proteins interact with specific chemical messengers and relay messages to the cell interior Cell recognition • Cell surface carbohydrates allow cells to recognize each other 3. Differentiate cytosol vs cytoplasm and membranous vs non-membranous organelles Cytoplasm • Jelly “cell forming material”, located between Whole cell without nucleus the plasma membrane and nucleus Cytosol • Viscous, semitransparent fluid, containing Whole cell without nucleus and organelles water, proteins, salts, sugars, and other solutes Membranous • Similar membrane to the plasma membrane but is around the organelle • Enables the organelle to maintain internal environment from external Non-membranous • Ribosomes and centrioles 4. Structure and function of organelles Cell part Structure Functions Plasma Membrane Plasma Membrane • Membrane made of a • Serves as an external cell barrier double layer of • Transmembrane proteins act as phospholipids which receptors for chemical messengers cholesterol and proteins (e.g., hormones, neurotransmitters), as are embedded transport proteins, and in cell-to-cell • Proteins may extend recognition entirely through the lipid • Maintains a resting potential that is bilayer or protrude on only essential for functioning of excitable one face cells • Most externally facing proteins and some lipids have attached sugar groups Cytoplasm Cytoplasm • Cellular region between the nuclear and plasma membranes • Consists of fluid cytosol containing dissolved solutes, organelles, and inclusions Mitochondria • • Ribosomes • • Rough endoplasmic reticulum • • Smooth endoplasmic reticulum • • Golgi apparatus Peroxisomes • • Rodlike, double membrane structures Inner membrane folded into projections called cristae Dense particles consisting of two subunits, each composed of ribosomal RNA and protein Free or attached to rough endoplasmic reticulum Membranous system enclosing a cavity, the cistern, and coiling through the cytoplasm Externally studded with ribosomes • • Site of ATP synthesis Powerhouse of the cell • The sites of protein synthesis • Sugar groups are attached to proteins within the cisterns Proteins are bound in vesicles for transport to the Golgi apparatus and other sites External face synthesizes phospholipids Membranous system of sacs and tubules Free of ribosomes • Site of lipid and steroid (cholesterol) synthesis, lipid metabolism, drug detoxification and Ca2+ storage A stack of flattened membranes and associated vesicles close to the nucleus • Packages, modifies, and segregates proteins for secretion from the cell, inclusion in lysosomes, and incorporation into the plasma membrane Modifies carbohydrates on proteins The enzymes detoxify a number of toxic substances The most important enzyme, catalase, breaks down hydrogen peroxide Membranous sacs containing catalase and oxidase enzymes • • • • • Lysosomes • Membranous sacs containing acid hydrolases • Sites of intracellular digestion Microtubules • Cylindrical structures made of tubulin proteins • • Support the cell and give it shape Involved in intracellular and cellular movements Form centrioles and cilia and flagella, if present The stable cytoskeletal elements; resist mechanical forces acting on the cell • Intermediate filaments • Protein fibers; composition varies • Microfilaments Centrioles • • Fine filaments composted of the protein actin Paired cylindrical bodies, each composed of nine triplets of microtubules • • • • • Involved in muscle contraction and other types of intracellular movements Help form the cells cytoskeleton As part of the centrosome, organize the microtubule network During mitosis, form the spindle and asters As basal bodies, form the bases of cilia and flagella Storage for nutrients, wastes, and cell products Inclusions • Varied; includes stored nutrients such as lipid droplets and glycogen granules, protein crystals, pigment granules • Cellular Extensions Cilia • Short, cell-surface projections Each cilium contains nine pairs of microtubules surrounding a central pair Like a cilium, but longer Only example in humans is a sperm tail Tubular extensions of the plasma membrane Contain a bundle of actin filaments • Coordinated movement creates a unidirectional current that propels substances across cell surfaces • Propels the cell • Increase surface area for absorption Largest organelle Surrounded by the nuclear envelope Contains fluid nucleoplasm, nucleoli, and chromatin Double-membrane structure pierced by pores Outer membrane continuous with the endoplasmic reticulum • • Control center of the cell Responsible for transmitting genetic information and providing the instructions for protein synthesis • Separates the nucleoplasm from the cytoplasm and regulates passage of substances to and from the nucleus Dense spherical (nonmembrane-bounded) bodies, composed of ribosomal RNA and proteins • Site of ribosome subunit manufacture • Flagellum • • Microvilli • • Nucleus Nucleus • • • Nuclear envelope • • Nucleolus • Chromatin • Granular, threadlike material composed of DNA and histone proteins • DNA constitutes the genes 5. Review membrane transport mechanisms Passive Transport ▪ ▪ ▪ ▪ • • • • • • • Higher concentration of molecules and ions = rapid diffusion Smaller molecules = rapid diffusion Increased temperature = rapid diffusion Lipid soluble = diffusion across plasma membrane Diffusion: movement of molecules or ions from an area where they are in higher concentration to an area where they are in lower concentration (concentration gradient). The driving force is intrinsic kinetic energy; constant random and high-speed motion between molecules and ions cause collisions, ricocheting off each other. Simple diffusion – substances diffuse directly through the lipid bilayer. Usually non-polar like, oxygen, carbon dioxide, steroid hormones, and fatty acids Facilitated diffusion – certain molecules like glucose and other sugars, some amino acids, and ions are transported passively even though they can cross the lipid bilayer Saturable – there is a maximum rate of transport because there are only a limited number of proteins in the membrane. Once the cell is “saturated” or “full” of what needs to be transported, it cannot carry any more. Binds to carrier proteins in the membrane o Transmembrane protein that transports specific polar molecules or classes of molecules such as sugars and amino acids o Alternates its shape to envelop and transport substance, allowing it to bypass the nonpolar regions o High to low concentration gradient Moves through water-filled channel proteins o Transmembrane proteins that transport substances, usually ions or water, through aqueous channel from one side of the membrane to the other o Leakage channels which are always open and allow ions or water to move according to concentration gradients o Gated channels are controlled by chemical or electrical signals Osmosis – diffusion of a solvent such as water through a selectively permeable membrane. Occurs whenever the water concentration differs on either side of the membrane • • o Water moves freely and reversible through water-specific channels constructed by transmembrane proteins called aquaporins. These are particularly abundant in RBCs and kidney tubules o Water will go wherever the higher concentration of solutes are to balance the cells Osmolarity = the total concentration of all solute particles in a solution Tonicity = how the solution affects cell volume (isotonic, hypertonic, hypotonic) Active Transport • Requires ATP to move substances across the plasma membrane, against the electrochemical gradients • Primary active transport – energy comes directly from the hydrolysis of ATP (ATP – ADP + P). ATP hydrolysis causes the phosphorylation of transport proteins which leads to the change in the shape of transport proteins and pumping the bound solute across the membrane. o Examples: Na+K+ pump • Secondary active transport – move more than one substance at a time. The transport is driven indirectly by the energy stores in ionic (concentration) gradients created by operation of primary active transport system • Vesicular transport – large particles and macromolecules are transported across cell membranes using vesicles o Exocytosis – moving substances from cell interior into extracellular space o Endocytosis – moving substances from cell exterior into cell interior o Phagocytosis – cells engulf large particles such as bacteria, cell debris using pseudopods o Pinocytosis – a process by which plasma membrane surrounds a very small volume of extracellular fluid containing dissolved molecules. Membrane edges fuse, forming a fluid filled vesicle o Receptor-mediated endocytosis – extracellular substances bind to specific receptor proteins, enabling the cell to ingest and concentrate ligands in protein coated vesicles Process Energy Source Description Examples Active Transport Primary Active transport ATP • • • Secondary active transport Ion concentration gradient maintained with ATP • • Transport of substances against a concentration (or electrochemical) gradient A pump protein moves substances across the plasma membrane Directly uses energy of ATP hydrolysis Cotransport (coupled transport) of two solutes across the membrane Energy is supplied by the concentration gradient Ions (Na+, K+, H+, Ca2+, and others) Movement of polar or charged solutes, e.g., amino acids (into cell by symporters); Ca2+, H+ (out of cells via antiporters) • created by primary active transport Symporters move the transported substances in the same direction; antiporters move transported substances in the opposite directions across the membrane Vesicular Transport Endocytosis ATP • A large external particle (proteins, bacteria, dead cell debris) is surrounded by a pseudopod and becomes enclosed in a vesicle (phagosome) Occurs primarily in phagocytes (some white blood cells and macrophages) Pinocytosis (fluid-phase ATP endocytosis) • Plasma membrane sinks beneath an external fluid droplet containing small solutes Membrane edges fuse, forming a fluid-filled vesicle Occurs in most cells; important for taking in dissolved solutes by absorptive cells of the kidney and intestine Phagocytosis • Receptor-mediated endocytosis ATP • • External substance binds to Means of intake of some hormones, cholesterol, iron membrane receptors Selective endocytosis and and most macromolecules transcytosis Vesicular trafficking ATP • trafficking Vesicles pinch off from Intracellular organelles and travel to other between certain organelles, organelles to deliver their cargo e.g., endoplasmic reticulum and golgi apparatus Exocytosis ATP • Secretion or ejection of Section neurotransmitters, substances from a cell Substance is enclosed in a hormone, mucus, etc membranous vesicle, which fuses with the plasma membrane and ruptures, releasing the substance to the exterior • of 6. Types of cell junctions and signal transduction Cell Junction Type Function Tight junctions (zipper of • Interlocking junctional proteins on Ziploc) the plasma membrane that form a continuous seal around the cell, resulting it to be impermeable • This causes a prevention of molecules from passing between cells • Some can be “leaky” and may allow some ions to pass Desmosomes (binding • They are anchoring junctions that bodies) bind adjacent cells together like molecular Velcro • Helps cells from “tearing” apart • Consists of plaque, linker proteins and intermediate filament (keratin) Gap Junction • A “communicating” junction (communication) • Connexons (hollow cylinders) which are composed of transmembrane proteins form channels between cells, which allow ions and small molecules to pass from cell to cell • Each junction can be different transmembrane proteins, and each will determine what can pass through them • Location Epithelial cells lining the digestive tract keep digestive enzymes and microorganisms in the intestine from going into the bloodstream • Most abundant in tissues subjected to mechanical stress. Example skin and heart muscle • Present in excitable tissues like the heart and smooth muscle. Ion passage helps synchronize their electrical activity and contraction 7. See key concepts for CV physiology Cardiovascular Part 1: Anatomy and Physiology 1. Review heart structures and blood flow 2. Anatomy of heart wall and all about valves The heart wall is composed of 3 layers: • Epicardium – visceral layer of the serous pericardium, often infiltrated with fat, especially in older people • Myocardium – composed mainly of cardiac muscle and forms the bulk of the heart. It is the layer that contracts. The cardiac muscle cells are arranged in spiral or circular bundles. These interlacing bundles effectively link all parts of the heart together • Endocardium – “Inside the heart”. Composed of a glistening white sheet of squamous epithelium resting on a thin connective tissue layer. Located on the inner myocardial surface, it lines the heart chambers and covers the fibrous skeleton of the valves. It is continuous with the endothelial linings of the blood vessels leaving and entering the heart Heart valves: facilitate blood flow in one direction • Atrioventricular valves – prevent backflow into the atria when the ventricles contract and depend upon pressures o Tricuspid valve – “right AV valve” Has 3 flexible cusps (flaps of endocardium reinforced by connective tissue) o Mitral valve – “Left AV valve” ▪ Has 2 cusps ▪ Also, can be called the ‘bicuspid’ valve • Each AV valve flap have chordae tendineae attached, which are tiny white collagen cords. These “heart strings” anchor the cusps to the papillary muscles which protrude from the ventricular musculature. Both structures act as tethers that anchor the valve flaps in their closed position. If the cusps were not anchored, they would be blown upward (everted) into the atria • Semilunar valves – guard the bases of the large arteries issuing from the ventricles (aorta and pulmonary trunk) and prevent backflow into the associated ventricles. Each valve has 3 pocketlike cusps, each shaped like a crescent moon o Aortic ▪ Valve leading to aorta, to pump blood to rest of body o Pulmonary ▪ Valve leading to lungs ▪ 3. Anatomy and physiology of coronary circulation • The myocardium in the heart is too thick to allow diffusion via the blood within the chambers. As a result, the coronary arteries provide the nourishment. The coronary circulation is also the shortest circuit in the body. These vessels and their main branches lie in the epicardium and send branches inward to nourish myocardium. They deliver blood when the heart is relaxed (diastole) and are ineffective when the ventricles contract because they are compressed by the contracting myocardium • Major coronary arteries: the left and right coronary arteries both arise from the base of the aorta and encircle the heart in the coronary sulcus. They provide the atrial supply of the coronary circulation: o Left coronary artery - runs toward the left side of the heart and then divides into two major branches: ▪ Anterior interventricular artery (left anterior descending artery). It follows the anterior interventricular sulcus, supplies blood to the interventricular septum and anterior walls of both ventricles ▪ Circumflex artery – supplies the left atrium and posterior walls of the left ventricle o Right coronary artery – to the right side of the heart and serve the right atrium and nearly all of the right ventricle. It has two branches: ▪ Right marginal artery – supplies the myocardium of the lateral right side of the heart ▪ Posterior interventricular artery – runs to the heart apex and supplies the posterior ventricular walls. Near the apex of the heart, this artery merges (anastomoses) with the anterior interventricular artery • Coronary veins: after blood passes through the capillary beds of the myocardium, the venous blood is collected by the cardiac veins, whose paths roughly follow those of the coronary arteries. All of these veins join to form an enlarged vessel called the coronary sinus, which empties blood into the right atrium. The coronary sinus is obvious on the posterior aspect of the heart: o The sinus has three large branches: ▪ Great cardiac vein, in the anterior interventricular sulcus ▪ Middle cardiac vein, in the posterior interventricular sulcus ▪ Small cardiac vein, running along the hearts right inferior margin o Additionally, several anterior cardiac veins empty directly into the right atrium anteriorly 4. Cardiac cycle: major events • Cardiac cycle – includes all events associated with the blood flow through the heart during one complete heartbeat (atrial systole and diastole followed by the ventricular systole and diastole). The cardiac cycle is marked by succession of pressure, blood volume changes related to pressure gradient 1. Ventricular filling: mid-to-late diastole • Pressure in the heart is low, AV valves are open, and blood is passively flowing from the atria in the ventricles, and SL valves are closed • 80% of the ventricular filling occurs during this period and the remaining 20% of the blood is delivered to the ventricles when the atria contract • Following depolarization of the atria (P wave), this causes a slight rise in atrial pressure, which propels the residual blood into the ventricles • At this point the ventricles are in the last part of diastole and have the maximum amount of blood (EDV: end diastolic volume) • Then the atria relax, and the ventricles depolarize (QRS complex); atrial diastole persists through the rest of the cycle 2. Isovolumetric contraction (2a) • The ventricles begin to contract, pressure rises rapidly and sharply closing the AV valves (first heart sound) • At this point the AV valves and the SL valves are closed for a split-second period; the blood volume remains constant in the chambers while the ventricles contract • Ventricular pressure continues to rise, and finally exceed the pressure in the large arteries, which causes the SL valves to open • This stage ends with the SL valves opening 3. Ventricular ejection (2b) • Blood rushes into the aorta and pulmonary trunk 4. Isovolumetric relaxation: early diastole • Following the peak of the T wave, the ventricles relax. The ESV (end systolic volume), is no longer compressed, ventricular pressure drops rapidly and blood in the aorta and pulmonary trunk flows back toward the heart, closing the SL valves (second heart sound). Again, SL and AV valves are closed, and the volume of blood doesn’t change • The cycle begins again, where the AV valves open and begin to passively flow down the pressure gradient 5. Cardiac output: definition and determinants • Stroke volume – the volume of blood pumped out by one ventricle with each beat o Depends on the degree to which venous return stretches the heart muscle. Anything that influences heart rate or blood volume influences venous return, hence stroke volume o The difference between EDV and ESV ▪ EDV is the intrinsic factor influencing SV and is determined by how long the ventricular diastole lasts and by venous pressure (normally 120mL). An increase in either factor raises EDV ▪ ESV determined by arterial blood pressure and the force of the ventricular contraction (approx. 50mL). The higher the arterial BP the higher the ESV • Cardiac output – amount of blood pumped out by each ventricle in 1 min (typically 5L/min) Determinants • Regulation of stroke volume o Preload – degree of stretch of the heart muscle and affects EDV ▪ Resting cardiac cells are normally shorter than optimal length. As a result, the stretch of the muscle exaggerates the increase in contractile force ▪ Venous return is an important factor to stretching heart muscle. Anything increasing venous return will increase EDV and impact the SV and contraction force ▪ Exercise and increased filling time increase EDV. The stimulation of the SNS and the squeezing action of the skeletal muscle compress the veins during exercise, resulting in an increase of venous return, thus preload ▪ Conversely, low venous return from severe blood loss or extremely rapid HR does not allow enough time for the heart to fill. This decreases EDV, causing the heart to beat less forcefully and lowering SV ▪ If one side of the heart has more blood it will impact the other side of the heart muscle to increase and therefore increase output o Contractility – contractile strength achieved at a given muscle length that affects ESV ▪ It is independent of muscle stretch and EDV ▪ Extrinsic factors influence heart muscle contractility ▪ Contractility rises when more Calcium enters the cytoplasm from the extracellular fluid and the sarcoplasmic reticulum ▪ Enhanced contractility = more blood ejected from the heart, greater SV, and lower ESV ▪ Increased sympathetic stimulation increases contractility, allowing an increase of calcium entry, which promotes more cross bridge binding an enhances ventricular contractility ▪ Positive inotropic agents increase contractility • Hormones such as epinephrine, thyroxine, and glucagon • Drug digitalis • High levels of extracellular calcium ▪ Negative inotropic agents decrease contractility • • Acidosis (excess H+), rising extracellular potassium levels Drugs: calcium channel blockers o Afterload – the pressure that ventricles must overcome to eject blood (back pressure that the arterial blood exerts on the aortic and pulmonary valves; about 80mmHg in aorta and 10mmHg in the pulmonary trunk). Also, it affects ESV ▪ In healthy individuals the SV remains relatively constant ▪ Hypertension reduces the ability of the ventricles to eject blood because of the increased pressure within the blood vessels. As a result, more blood remains in the heart after systole, increasing ESV and reducing SV • Regulation of heart rate – when SV declines CO is maintained by increasing HR and HR and contractility, with the effort of trying to circulate blood effectively o Homeostatic mechanisms are induced neurally, chemically, and physically ▪ Positive chronotropic factors increase HR and negative chronotropic factors decrease HR ▪ Autonomic nervous system (ANS) • The ANS (SNS and PNS) exerts the most important extrinsic controls affecting HR • Sympathetic nerve fibers to release norepinephrine at their cardiac synapses. These bind to the beta 1 receptors in the heart causing the threshold to be reached more quickly. As a result, the SA node fires more rapidly, and the heart responds by beating faster • Sympathetic stimulation also enhances contractility and decreases relaxation. By enhancing calcium movements in the contractile cells, the increase in contractility lowers the ESV, so SV does not decline as it would if only the HR was increased (when the HR beats faster there is little time for ventricular filling, so EDV is lowered) • Parasympathetic nerve fibers release acetylcholine, which hyperpolarizes the membranes of its effector cells by opening potassium channels. It slows down the HR but has little effect on contractility because innervation of the ventricles by the vagus nerves are sparse o Chemical regulation ▪ Hormones • Epinephrine released by the adrenal medulla from the stimulation of the SNS, enhances HR and contractility • Thyroxine releases from the thyroid gland increases metabolic rate and production of body heat. When large quantities are released, it works directly on the heart and causes a sustained increase in HR. It also enhances the effects of epinephrine and norepinephrine ▪ Ions • Normal function depends on having normal levels of intracellular and extracellular ions, particularly potassium and calcium o Other factors ▪ Age – fetus has a high HR (140-160bpm) and it gradually decrease over age. Average adult HR is 60-100bpm ▪ Gender – women have an average HR that is faster than men ▪ Exercise – stimulates SNS and increases systemic blood pressure and routes more blood to the muscles Body temperature – heat increase HR by enhancing the metabolic rate of the cardiac cells (example, febrile). Cold temperature decreases HR ▪ 6. Frank-Starling’s Law and clinical implications The Frank-Starling Mechanism is the ability of the heart to change its force of contraction and therefore stroke volume in response to changes in venous return. It is definitively the relationship between preload and stroke volume ▪ Preload: the degree to which the cardiac muscle stretches just before contraction ▪ Stroke volume: the volume of blood pumped out of the ventricle with each contraction The more a ventricle fills with blood during diastole = the greater the volume of blood ejected from the heart during systole contraction → the greater amount of blood entering the heart during diastole reflects a greater volume of blood that is ejected from the heart during systole. Over time the heart loses its stretch ability/contractility which in turn decreases the stroke volume A healthcare professional must understand that the Frank Sterling Law involved the stretching of sarcomeres and tension that is created. Think of the sarcomere as a rubber band that when stretched will return to its normal size but if continuously stretched over long periods of time will lose its elasticity and have a more difficult time returning to its original size and shape. In clinical scenario such as working with a patient experiencing congestive heart failure the sarcomeres are too weak and have been stretched to their maximum capacity. This causes the heart to not be able to pump out all the returned blood to the heart and causes overloading. Overtime the cycle will continue and the patient’s condition will worsen. Understanding the mechanism of action of such a condition can help healthcare professionals provide appropriate care and be able to think critically in an emergency. 7. Electrical regulation, pathways, and basic ECGs Intrinsic (noncontractile) The heart has two different myocytes: • Contractile Responsible for heart pumping activity • Noncontractile (pacemaker cells) o Spontaneously depolarize AKA automaticity (able to depolarize on its own without extrinsic nerves) Majority are contractile cells but, in some locations, there is noncontractile cells. The electrical regulation and pathways are done from the intrinsic conduction system (pacemaker cells; in picture) and by the extrinsic conduction system (SNS and PNS fibers) and the action potentials of contractile cells. o SNS Cardio-acceleratory center • Projects to sympathetic neurons in the T1-T5 level of the spinal cord • These preganglionic neurons, in turn, synapse with postganglionic neurons in the cervical and upper thoracic sympathetic trunk • From there, postganglionic fibers run through the cardiac plexus to the heart where they innervate the SA and AV nodes, heart muscle and coronary arteries • Results in an increase in both heart rate and contractility PNS Cardio-inhibitory center • Sends impulses to the parasympathetic dorsal vagus nucleus in the medulla, which in tun sends inhibitory impulses to the heart via branches of the vagus nerves • Most parasympathetic postganglionic motor neurons lie in ganglia in the heart wall and their fibers project most heavily to the SA and AV nodes • Results in slow HR • The photo depicts the action potential of the contractile cells. The major difference between the pacemaker and contractile cell action potentials is in the depolarization phase. In the pacemaker, depolarization is the influx of calcium that influences the action potential and reverses the membrane potential. In the contractile action potential, it is the sodium influx that produces these changes • Another difference is that the contractile action potential has a plateau phase which allows: o The contraction to be sustained to efficiently eject blood from the heart o Ensure a long absolute refractory period, to avoid tetanic contractions and to ensure the heart can fill again before the next beat Cardiovascular Part 2: Circulation and Muscle Contraction 1. Structure and function of the sarcomere: include troponin, tropomyosin, actin, myosin Microscopic Anatomy • Cardiac muscle is striated and contracts by the sliding filament mechanism • Cardiac cells are short, fat, branched, and interconnected • Each cardiac myocyte contains one or at most two large, pale, centrally located nuclei • The intercellular spaces are filled with a loose connective tissue matric (the endomysium) containing numerous capillaries o Delicate matrix connected to the fibrous cardiac skeleton, which acts both as a tendon and as an insertion, giving the cardiac cells something to pull or exert their force against • Plasma membranes of adjacent cardiac cells interlock like the ribs of two sheets of corrugated cardboard at dark staining junctions called intercalated discs o Contain anchoring desmosomes, and gap junctions ▪ Desmosomes prevent adjacent cells from separating during contraction ▪ Gap junctions allow ions to pass from cell to cell, transmitting current across the entire heart • Large mitochondria account for 25-35% of the volume of cardiac cells which makes them highly resistant to fatigue • Most of the remaining volume is occupied by myofibrils composed of fairly typical sarcomeres o The sarcomeres have Z discs, A bands, and I bands that reflect the arrangement of the thick (myosin) and thin (actin) filaments comprising them o The myofibrils of cardiac muscle cells vary greatly in diameter and branch extensively, accommodating the abundant mitochondria between them ▪ Produces a binding pattern less dramatic than that seen in skeletal muscle • The system for delivering calcium is less elaborate in cardiac muscle cells • The cardiac sarcoplasmic reticulum is simpler and lacks the large terminal cisterns seen in skeletal muscle o As a result, cardiac muscle fibers do not have triads o The T tubules are wider and fewer than in skeletal muscle, they enter the cells oncer per sarcomere at the Z discs ▪ T tubules are invaginations of the sarcolemma (invaginate twicer per sarcomere, at the A band-I band junctions in skeletal muscles) Myofibrils • A single muscle fiber contains hundreds to thousands of rods like myofibrils that run parallel to its length o Made up of a chain of sarcomeres linked end to end ▪ Sarcomeres contain even smaller rod-like structures called myofilaments Myofilaments • Small structures, myofilaments or filaments, are arranged in an orderly fashion that give the sarcomere its banding pattern o Are the muscle equivalents of the actin-containing microfilaments and myosin motor proteins ▪ Actin and myosin play a role in motility and shape change in virtually every cell in the body. This property reaches its highest development in the contractile muscle fibers. There are two types of contractile myofilaments in a sarcomere o The central thick filaments containing myosin (red) extend the entire length of the A band, they are connected in the middle of the sarcomere at the M line o The more latter thin filaments contain actin (blue) extend across the I band and partway into the A band. The Z disc, a protein sheet, anchors the thin filaments • Muscle contraction depends on the myosin- and actin-containing myofilaments • Thick filaments – composed primarily of the protein myosin • Thin filaments – composed chiefly of the protein actin o Actin has kidney shaped polypeptide subunits, called globular actin or G actin o Each G actin has a myosin-binding site (active site) to which the myosin heads attach during contraction o Two intertwined actin filaments, form the backbone of each thin filament • Thin filaments also contain several regulatory proteins; o Polypeptide strands of tropomyosin, a rod-shaped protein, spiral about the actin core and help stiffen and stabilize it. Arranged end to end along the actin filaments, and in a relaxed muscle fiber, they block myosin-binding sites on actin so that myosin heads on the thick filaments cannot bind with the thin filaments o Troponin, the other major protein in thin filaments, is a globular protein with three polypeptide subunits. One attaches to actin, another binds to tropomyosin and helps position it on actin and the third subunit binds calcium ions ▪ Both troponin and tropomyosin help control the myosin-actin interactions involved in contraction Sliding Filament Model of Contraction • Contraction refers only to the activation of myosin’s cross bridges, which are force-generating sites • Shortening only occurs if the cross bridges generate enough tension on the thin filaments to exceed the forces that oppose shortening • Contraction ends when the cross bridges become inactive, the tension declines, and the muscle fiber relaxes • In the relaxed muscle fiber, the thin and thick filaments overlap only at the ends of the A band • The sliding filament model of contraction states that during contraction, the thin filaments slide past the thick ones so that the actin and myosin filaments overlap to a greater degree • Never the thick nor the thin filaments change length during contraction • When nervous system stimulates muscle fibers, the myosin heads on the thick filaments latch onto myosin-binding sites on actin in the thin filaments, and the sliding starts • These cross-bridge attachments form and break several times during a contraction, acting like tiny ratches to generate tension and propel the thin filaments toward the center of the sarcomere o As this event occurs, simultaneously in sarcomeres throughout the cell, the muscle cell shortens 2. Cardiac muscle contraction • Contraction is preceded by a depolarization in the form of an action potential (AP) o Transmission of the depolarization wave down the T tubules causes the sarcoplasmic reticulum (SR) to release Ca2+ into the sarcoplasm o Excitation-contraction coupling occurs as Ca2+ provides the signal (via troponin binding) for cross bridge activation ▪ This sequence of events couples the depolarization wave to the sliding of the myofilaments in both skeletal and cardiac muscle cells • Some cardiac muscle cells are self-excitable • The heart contains two kinds of myocytes o Almost all are contractile cardiac muscle cells, responsible for the heart’s pumping activity o Certain locations in the heart contain special noncontractile cells, called pacemaker cells, that spontaneously depolarize ▪ This property is called automaticity or auto-rhythmicity o Because heart cells are electrically joined together by gap junctions, these cells can initiate not only their own depolarization, but also that of the rest of the heart. No neural input is required • The heart contracts as a unit o Because gap junctions tie cardiac muscle cells together, this allows the wave of depolarization to travel from cell to cell across the heart ▪ Either all fibers in the heart contract as a unit or the heart doesn’t contract at all • Contraction of all cardiac myocytes ensures effective pumping by the heart • The influx of Ca2+ from extracellular fluid triggers Ca2+ release from the SR o In cardiac muscle, depolarization opens special Ca2+ channels in the plasma membrane ▪ These slow Ca2+ channels allow entry of 10-20% of the Ca2+ needed for contraction ▪ Once inside, this influx of Ca2+ triggers Ca2+-sensitive channels in the SR to release bursts of Ca2+ (‘calcium sparks’) that account for the other 80-90% needed for contraction • Tetanic contractions cannot occur in cardiac muscles o Absolute refractory period is the period during an APR when another AP cannot be triggered ▪ If the heart were to contract titanically, it would be unable to relax and fill, and would be useless ▪ The absolute refractory period in the heart is nearly as long as the contraction itself, which prevents tetanic contractions • The heart relies almost exclusively on aerobic respiration o Cardiac muscle has more mitochondria than skeletal muscle does, reflecting its greater dependence on oxygen for its energy metabolism o Cardiac muscle cannot effectively operate for long without oxygen o Cardiac muscle is more adaptable than skeletal and can readily switch metabolic pathways to use whatever nutrients are available, including lactic acid generated by skeletal muscle activity 3. Arteries and veins: structural and functional differences Circulatory Pathways • Blood vessels are dynamic structures that pulsate, constrict, relax, and even proliferate • The blood vessels form a closed delivery system that begins and ends at the heart Blood Vessel Structure and Function • Three major types of blood vessels are arteries, capillaries, and veins • As the heart contracts, it forces blood into the large arteries, leaving the ventricles • Blood then moves into successively smaller arteries, finally reaching the smallest branches, the arterioles, which feed into the capillary beds of body organs and tissues • Blood drains from capillaries into venules, the smallest veins, and then into larger and larger veins that • • • • merge to form the large veins that ultimately empty into the heart Arteries – carry blood away from the heart o Said to branch, diverge, fork, as they form smaller and smaller divisions Veins – carry blood toward the heart o Said to join, merge, converge, into successively larger vessels approaching the heart In the systemic circulation, arteries always carry oxygenated blood and veins always carry deoxygenated blood. The opposite is true in two special locations: o In pulmonary circulation, the arteries, still defined as vessels leading away from the heart, carry deoxygenated blood to the lungs, and the veins carry oxygenated blood from the lungs to the heart o In the special umbilical vessels of a fetus, the roles of the veins and arteries also differ with respect to oxygenation Of all the blood vessels, only capillaries have intimate contact with tissue cells and directly serve cellular needs o Exchanges between blood and tissue cells occur primarily through the gossamer-thin capillary walls Layers of Blood Vessels • The walls of all blood vessels, except the very smallest, have three distinct layers, or tunics (‘coverings’), that surround a central blood-containing space, the vessel lumen (cavity inside a tube, • • blood vessel or hollow organ) The innermost tunic is the tunica intima o Direct contact with the blood in the lumen o Contains the endothelium, the simple squamous epithelium that lines the lumen of all vessels ▪ Endothelium is continuous with the endocardial lining of the heart, and its flat cells fit closely together, forming a slick surface that minimizes friction as blood moves through the lumen • In vessels >1mm in diameter, a subendothelial layer, consisting of a basement membrane and loose connective tissue, supports the endothelium The middle tunic, tunica media, is mostly circularly arrange smooth muscle cells and sheets of elastin o Activity of the smooth muscle is regulated by sympathetic vasomotor nerve fibers of the autonomic nervous system and a whole battery of chemicals o Depending on the body’s needs, regulation causes either vasoconstriction or vasodilation o Activities of the tunica media are critical in regulating circulatory dynamics because small changes in • vessel diameter greatly influence blood flow and blood pressure o Generally, the tunica media is the bulkiest layer in arteries which bear the chief responsibility for maintaining BP and circulation The outermost layer, the tunica externa (also called tunica adventitia), is composed largely of loosely woven collagen fibers that protect and reinforce the vessel, and anchor it to surrounding structures o Infiltrated with nerve fibers, lymphatic vessels, and, in larger veins, a network of elastic fibers o In larger vessels, the tunica externa contains a system of tiny blood vessels, the vasa vasorum (‘vessels of vessels’) that nourish the more external tissues of the blood vessel wall o Innermost (luminal portion of the vessel obtains nutrients directly from blood in the lumen The three vessel types vary in length, diameter, wall thickness and tissue makeup • Arteries are pressure reservoirs, distributing vessels, or resistance vessels • In terms of relative size and function, arteries can be divided into three groups – elastic arteries, muscular arteries, and arterioles Elastic Arteries • Elastic arteries are the thick-walled arteries near the heart – the aorta and its major branches • Are the largest in diameter, ranging from 2.5-1cm and the most elastic o Large lumens make them low-resistance pathways that conduct blood from the heart to medium-sized arteries, elastic arteries are sometimes called conducting arteries • Contain more elastin than any other vessel type (present in all 3 tunics, tunica media contains the most) o The elastin constructs concentric ‘holey’ sheets of elastic connective tissue sandwiched between layers of smooth muscle cells • Are pressure reservoirs, expanding and recoiling as the heart ejects blood o Blood flows fairly continuously rather than starting and stopping with the pulsating rhythm of the heartbeat ▪ If the blood vessels become hard and unyielding, as in atherosclerosis, blood flows more intermittently • Without the pressure smoothing effect of the elastic arteries, the walls of the arteries throughout the body experience higher pressures o Arteries eventually weaken and may balloon out (aneurysm) or even burst Muscular Arteries • Distally the elastic arteries give way to the muscular arteries, which deliver blood to specific body organs (and so are sometimes called distributing arteries) • Account for most of the named arteries studied in anatomy • Internal diameter ranges from that of a little finger to that of a pencil lead • Muscular arteries have the thickest tunica media of all vessels o Contains relatively more smooth muscle and less elastic tissue than elastic arteries ▪ More active in vasoconstriction and less capable of stretching Arterioles • The smallest of the arteries, have a lumen diameter ranging from 0.3mm to 10nanometer • Larger arterioles have all three tunics, but their tunica media is chiefly smooth muscle with a few scattered elastic fibers • Smaller arterioles, which lead into the capillary beds, are little more than a single layer of smooth muscle cells spiraling around the endothelial lining • Minute-to-minute blood flow into the capillary beds is determined by arteriolar diameter, which varies in response to changing neural, hormonal, and local chemical influences o Changing diameter changes resistance to blood flow, and so arterioles are called resistance vessels ▪ When arterioles constrict, the tissue served are largely bypassed ▪ When arterioles dilate, blood flow into the local capillaries increases dramatically Venules • Capillaries unite to form venules, which range from 8-100micrometers in diameter • The smallest venules, the postcapillary venules, consist entirely of endothelium around which pericytes congregate o Postcapillary venules are extremely porous (more like capillaries than veins in this way), and fluid and WBCs to the postcapillary venule endothelium, followed by their migration through the wall into the inflamed tissue • Large venules have a thin tunica media (1-2 layers of smooth muscle cells) and a thin tunica externa as well Veins • Veins carry blood from the capillary beds towards the heart • The diameter of successive venous vessels increases, and their walls gradually thicken as they progress from venules to larger and larger veins • Venules join to form veins • Usually have 3 distinct tunics, but their walls are always thinner and their lumens larger than those of corresponding arteries • Relatively little smooth muscle or elastin in the tunica media, which is poorly developed and tends to be thin even in the largest veins • Tunica externa is the heaviest wall layer o Thick longitudinal bundles of collagen fibers and elastic networks, often several times thicker than the tunica media ▪ In the largest veins (venae cavae) that return blood directly to the heart have longitudinal bands of smooth muscle which make the tunica externa even thicker • With large lumens and thin walls, veins can accommodate a fairly large blood volume • Veins are called capacitance vessels and blood reservoirs because they can hold up to 65% of the bodys blood supply at any time • Walls of veins which can be much thinner than arterial walls without danger of bursting because the BP in veins is low o Low-pressure condition demands several structural adaptations to ensure that veins return blood to the heart at the same rate it was pumped into the circulation ▪ E.g., large-diameter lumens, which offer relatively little resistance to blood flow Venous Valves • Prevent blood from flowing backward in veins, and represent another adaptation to compensate for low venous pressure • Formed from folds of the tunica intima and resemble the semilunar valves of the heart • Most abundant in the veins of the limbs, where gravity opposes the upward flow of blood • Usually absent in veins of the thoracic and abdominal cavities Homeostatic Imbalance • Varicose veins are veins that are tortuous and dilated because of incompetent (leaky) valves o More than 15% of adults suffer (usually lower limbs) o Several factors contribute, including heredity and conditions that hinder venous return, such as prolonged standing in one position, obesity, or pregnancy ▪ Exert downward pressure on vessel of the groin, restricting return of blood to the heart • ▪ Blood pools in the lower limbs, and with time, the valves weaken, and the venous walls stretch Elevated venous pressure can also cause • E.g., straining to deliver a baby, or having a BM raises intraabdominal pressure, preventing blood from draining from anal veins o Results in varicosities called hemorrhoids Venous Sinuses • Venous sinuses are highly specialised, flattered veins with extremely thin walls composed only of one endothelium • Examples include coronary sinus of the heart and the Dural venous sinuses of the brain Anastomoses • Blood vessels form special interconnections called vascular anastomoses • Most organs receive blood from more than one arterial branch, and arteries supplying the same territory often merge, forming arterial anastomoses o Provide alternative pathways, called collateral channels, for blood to reach a given body region ▪ If one branch is cut or blocked by a clot, the collateral channel can often provide sufficient blood to the area • Arterial anastomoses occur around joints, where active movement may hinder blood flow through one channel o Also common in abdominal organs, the heart, the brain • Arteries that supply the retina, kidneys, and spleen either do not anastomose or have a poorly developed collateral circulation o If their blood flow is interrupted, cells supplied by such vessels die • The metarteriole – throughfare channel shunts of some capillary beds that connect arterioles and venules are examples of arteriovenous anastomoses • Veins connect much more freely than arteries, and venous anastomoses are common o Occluded vein rarely blocks blood flow or leads to tissue death 4. Factors affecting circulation Blood flows from high to low pressure against resistance • Blood flow, blood pressure, and resistance – examine how these factors relate to physiology of blood circulation Definition of Terms • Blood flow – the volume of blood flowing through a vessel, an organ, or the entire circulation in a given period (mL/min) o If we consider the entire vascular system, blood flow is equivalent to cardiac output (CO) ▪ Relatively constant under resting conditions ▪ At any given moment, blood flow through individual body organs may vary widely according to their immediate needs • Blood pressure (BP) – the force per unit area exerted on a vessel wall by the contained blood, is expressed in mmHg o Unless otherwise stated, the term blood pressure means systemic arterial blood pressure in the largest arteries near the heart o The hydrostatic pressure gradient – the differences in BP within the vascular system – provides the driving force that keeps blood moving, always from an area of higher pressure to an area of lower pressure, through the body • Resistance – opposition of flow and is a measure of the amount of friction blood encounters as it passes through the vessels o Most is encountered in the peripheral (systemic) circulation, well away from the heart, we generally use the term total peripheral resistance (TPR) o There are 3 important sources of resistance: blood viscosity, vessel length and vessel diameter Blood Viscosity • Internal resistance to flow that exists in all fluids is viscosity and is related to the thickness or “stickiness” of a fluid • The greater the viscosity, the less easily molecules slide past one another and the more difficult it is to get and keep the fluid moving o Blood is much more viscous than water, because it contains formed element and plasma proteins, it flows more slowly under the same conditions • Blood viscosity is fairly constant o Conditions such as polycythemia (^RBCs) can increase blood viscosity and so resistance increases o If the RBC count is low, as in some anemias, blood is less viscous and TPR declines Total Blood Vessel Length • Relationship between total blood vessel length and resistance is straightforward: the longer the vessel, the greater the resistance o E.g., infants blood vessels length as they grow into adulthood, and so both TPR and BP increase Blood Vessel Diameter • The smaller the diameter, the greater the resistance • Blood viscosity and vessel length are normally unchanging in the short term, and so the influence of these factors can be considered constant • Blood vessel diameter changes frequently and significantly alter TPR Principle of Fluid Flow • Fluid close to the wall of a tube or channel is slowed by friction as it passes along the wall, whereas fluid in the center of the tube flows more freely and faster • In a tube of a given size, the relative speed and position of fluid in the different regions of the tube's cross section remain constant, a phenomenon called laminar flow or streamlining o The smaller the tube, the greater the friction, because relatively more of the fluid contacts the tube wall, where its movement is impeded o Large arteries close to the heart, which do not change dramatically in diameter, contribute little to TPR ▪ Small-diameter arterioles, which can enlarge or constrict in response to neural and chemical controls, are the major determinants of TPR o When blood encounters either an abrupt change in vessel diameter or rough or protruding areas of the tube wall (such as fatty plaques), the smooth laminar blood flow is replaced by turbulent flow, that is, irregular fluid motion where blood form the different laminae (different layers of the tube's cross section) mixes ▪ Turbulence dramatically increases resistance Relationship between Flow, Pressure, and Resistance • Blood flow (F) is directly proportional to the difference in blood pressure (∆𝑃) between two points in circulation blood pressure or hydrostatic pressure gradient o When ∆𝑃 increases, blood flow increases, and when ∆𝑃 decreases, blood flow decreases • Blood flow is inversely proportional to the TPR in the systemic circulation o If TPR increases, blood flow decreases o • 𝐹= ∆𝑃 𝑇𝑃𝑅 Of these two factors influencing blood flow, TPR is far more important than ∆𝑃 in influencing local blood flow because TPR can easily be changed by altering blood vessel diameter Intrinsic and Extrinsic Controls Determine Blood Flow Through Tissues • • Blood flow through body tissues, or tissue perfusion, is involved in: o Delivering oxygen and nutrients to tissue cells, removing CO2 and wastes o Gas exchange o Absorbing nutrients in the GI tract o Forming urine The rate of blood flow to each tissue and organ is almost exactly the right amount to provide proper function o Achieved by intrinsic controls (autoregulation) acting automatically on the smooth muscle of arterioles that feed any given tissue o Extrinsic controls act on arteriolar smooth muscle to maintain BP ▪ Acts via the SNS and hormones of the nervous and endocrine systems • Reduce blood flow to regions that need it the least, maintaining a constant MAP and allowing intrinsic mechanisms to direct blood flow to where it is most needed Autoregulation: Intrinsic (local) Regulation of Blood Flow • • • Changes in blood flow through individual organs are controlled intrinsically by modifying the diameter of local arterioles feeding the capillaries – organs regulate their own blood flow by changing resistance of their arteries Control mechanisms can be classified as metabolic (chemical) or myogenic (physical) o Both determine the final autoregulatory response of a tissue ▪ E.g., reactive hyperemia refers to the dramatically increased blood flow into a tissue that occurs after the blood supply to the area has been temporarily blocked Intrinsic and extrinsic control of arteriolar smooth muscle in systemic circulation Metabolic Controls • 5. Structure and function of micro circulation including fluid exchange at capillary level 6. Role of lymphatic vessels in micro circulation 7. Control of blood pressure: all mechanisms including local and systemic Respiratory Part 1: Anatomy and Physiology 1. Review function and lung anatomy: focus on pleura, lobes, major airways, alveoli 2. Anatomical and histology all differences between types of airways 3. Lung ventilation: how does it happen? Think pressures and Gas laws 4. Lung volumes: definitions and what they mean 5. Factors regulating lung recoil: compliance, elasticity, and surface tension 6. Surface tension 101 and role of surfactant 7. Lung ventilation: how is it regulated? Role of the neurological system? Respiratory Part 2: Cell Respiration’s 1. Anatomy of fetal circulation including before and after birth 2. Differences and similarities between fetal and adult hemoglobin 3. Physiology of alveolar gas exchange (pressure) and alveolar wall structure, include changes at high altitude (e.g., Mt Logan) and deep sea diving 4. Ventilation/perfusion ratio: what is it? What does it mean? 5. Transport of oxygen/carbon dioxide in blood: role of hemoglobin, pressures, and bicarbonate 6. Oxyhemoglobin dissociation curve: what is it? What does it mean? Why is it important? 7. Pulmonary circulation and regulation of blood flow Renal Part 1: Filtration 1. Functions of the urinary system 2. Anatomy of the kidney including locations and blood supply 3. Ultra-structure of the glomerulus 4. Fluid compartments, homeostasis and composition 5. Role of osmolarity, osmolality and toxicity equilibrium 6. Role of fluid intake and output in fluid balance, electrolyte composition, neural control 7. Thirst mechanism including anti-diuretic hormone (ADH) Renal Part 2: Fluid and Electrolytes, Acid/Base Balance 1. Glomerular filtration rate: how much, how does it happen, what are the determinants of who gets filtered and who doesn’t 2. Regulation mechanisms of GFR: myotonic, auto regulation, neural, hormonal 3. Tubules 1: if you were a water molecule, how would you be handled by the tubules include osmotic forces, counter current exchanger, ADH 4. Tubules 2: if you were a sodium ion, how would you be handled by the tubules include active transport, counter current multiplier, role of aldosterone in Na/K antiport 5. Tubules 3: if you were a glucose molecule how would you be handled including transport maximum implications 6. Endocrine function of the kidneys - the role of renin and aldosterone, how their release is regulated 7. Acid-base balance: why is our pH 7.4, what is a buffer and how do the lungs and kidneys control pH Nervous System Part 1: Overview 1. Organization of the nervous system including peripheral nervous system both motor and sensory The human nervous system is organized into two major divisions, the central nervous system (CNS) and peripheral nervous system (PNS) CNS • PNS • • Brain and spinal cord occupy the dorsal body cavity, this is the integrating and control center of the nervous system. It’s job is to interpret sensory input and dictate motor output based on reflexes, current conditions, and past experiences Made up of cranial nerves, carry impulses to and from the Pravin, the spinal nerves that carry impulses to and from the spinal cord and ganglia The PNS breaks down into two subdivisions: o Sensory (afferent) division - is made up of somatic sensory fibres and visceral sense or fibres. Somatic sensory fibres convert impulses from the skin, skeletal muscles, and joints. Visceral sensory fibres transmit impulses from the organs within the ventral body cavity o Motor (efferent) division - transmits impulses from the CNS to effector organs, which are muscles and glands. These impulses activate muscles and glands to contract and secrete ▪ Breaks down into two parts: • Somatic Nervous System (SNS) - is composed of somatic motor nerve fibres that conduct impulses from the CNS to skeletal muscles. AKA the voluntary nervous system because it