Transmembrane Ion Channels PDF
Document Details
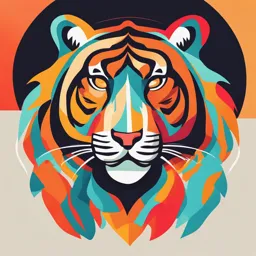
Uploaded by AmbitiousBlue
null
Tags
Summary
This document provides an overview of transmembrane ion channels, outlining their roles in neurotransmission, cardiac conduction, and muscle contraction. It also covers the mechanisms of action of associated drugs, like local anesthetics and benzodiazepines, on these channels.
Full Transcript
Transmembrane Ion Channels Many cellular functions require the passage of ions and other hydrophilic molecules across the plasma membrane Specialized transmembrane channels regulate these processes. (Regulating the flow of ions across cell membrane.) Functions of ion channels: 1. Neurotra...
Transmembrane Ion Channels Many cellular functions require the passage of ions and other hydrophilic molecules across the plasma membrane Specialized transmembrane channels regulate these processes. (Regulating the flow of ions across cell membrane.) Functions of ion channels: 1. Neurotransmission 2. Cardiac conduction 3. Muscle contraction 4. Secretion Transmembrane Ion Channels Three major mechanisms of transmembrane ion channels: Cannel Typer MOA Function Ligand-gated channels Conductance controlled by ligand binding to the Altered ion e.g. cholinergic nicotinic channel. conductance receptor Voltage-gated channels changes in voltage across the plasma membrane. Altered ion e.g. voltage-gated calcium conductance channels Second messenger- Binding of ligand to transmembrane Second messenger regulated channels receptor with G protein-coupled regulates ion cytosolic domain, leading to second conductance of channel messenger generation Transmembrane Ion Channels Two important classes of drugs altering ion channel conductance: Local anaesthetics Benzodiazepines Transmembrane Ion Channels Local anaesthetics Targeting Sodium Channels: Blocking voltage-gated sodium channels in the neuronal cell membrane. Inhibition of Action Potential: By inhibiting sodium channels, local anesthetics prevent the influx of sodium ions, which is essential for the initiation and propagation of action potentials along the nerve fiber Inhibit pain perception (nociception) by blocking pain signal transmission from the periphery to the central nervous system. Reversible Blockade: The blockade is reversible, meaning that once the anesthetic is removed or metabolized, normal nerve function can resume. Transmembrane Ion Channels Benzodiazepines Benzodiazepines bind to the GABA receptor complex in the brain. GABA is the primary inhibitory neurotransmitter in the CNS. They enhance GABA’s ability to open chloride channels, increasing the flow of chloride ions into neurons The influx of chloride ions hyperpolarizes the neuron, making it more negatively charged and less likely to fire an action potential. Resulting in CNS Depression: sedation, anxiolysis, muscle relaxation, and anticonvulsant actions. Transmembrane G Protein-Coupled Receptors G protein-coupled receptors (GPCRs): Most abundant class of receptors in the human body. Located at the extracellular surface of the plasma membrane Structure: GPCRs have seven transmembrane helices within a single polypeptide chain. Known as 7-transmembrane (metabotropicor - heptahelical) receptors. The extracellular domain usually contains the ligand-binding region. Intracellular G-protein (e.g. Gs, Gq, and Gi) >> 3 subunits (α, 𝛽, and 𝛄). Transmembrane G Protein-Coupled Receptors Mechanism of Action (MOA) of G Protein-Coupled Receptors (GPCRs) Mechanism of Action (MOA) of G Protein-Coupled Receptors (GPCRs) 1. Ligand Binding: An endogenous ligand (e.g., hormone, neurotransmitter) or exogenous drug binds to the extracellular domain of the GPCR. 2. Receptor Activation: The binding induces a conformational change in the GPCR. 3. G Protein Activation: The conformational change in the GPCR activates the associated G protein by promoting the exchange of GDP for GTP on the α subunit. Mechanism of Action (MOA) of G Protein-Coupled Receptors (GPCRs) 4. G Protein Subunit Dissociation: The GTP-bound α subunit dissociates from the βγ subunits.* 5. Effector Interaction: The dissociated α subunit and βγ subunits interact with various effector proteins (e.g., adenylyl cyclase, phospholipase C, ion channels). 6. Second Messenger Production: Effector proteins generate second messengers: ((One major role of the G proteins is to activate the production of second messengers.)): ✓ Adenylyl Cyclase: Converts ATP to cyclic AMP (cAMP) (second messenger) ✓ Guanylyl Cyclase: Converts GTP to cyclic GMP (cGMP). (second messenger) ✓ Phospholipase C: Cleaves PIP2 into IP3 and DAG. (second messenger) Mechanism of Action (MOA) of G Protein-Coupled Receptors (GPCRs) 7. Intracellular Signalling:Second messengers activate downstream signalling pathways: Second Results Activation Results messenger cAMP Activates protein kinase A (PKA). ▪ Phosphorylates target proteins, altering their activity. ▪ Regulates metabolic pathways like glycogen breakdown. ▪ Promotes smooth muscle relaxation. cGMP Activates protein kinase G (PKG). ▪ Promotes smooth muscle relaxation. ▪ Inhibits platelet aggregation. ▪ Facilitates vasodilation in blood vessels IP3 Releases Ca2+ from intracellular stores. increasing cytosolic Ca2+ concentration and triggering downstream events. DAG Activates protein kinase C (PKC).. Regulates cell growth and differentiation. Smooth muscle contraction The Major G Protein Families G Protein Isoforms: Numerous G protein isoforms exist, each with unique effects on targets. Isoforms are grouped into five major families: Gs, Gi, Go, Gq, and G12/13. Differential functioning of these G proteins is important for drug selectivity. Important class in the G protein-coupled receptor family Beta-Adrenergic Receptors: Stimulated by catecholamines A significant class within the G protein- (epinephrine and norepinephrine) binding coupled receptor family. to the receptor's extracellular domain. Includes β1, β2, and β3 receptors. Epinephrine binding induces a ✓ β1 receptors control heart rate. conformational change, activating G ✓ β2 receptors relax smooth muscle. proteins and increasing intracellular cAMP ✓ β3 receptors mobilize energy from levels, leading to downstream cellular fat cells. effects. Transmembrane Receptors with Linked Enzymatic Domains Function: These receptors convert extracellular ligand-binding interactions into intracellular actions by activating a linked enzymatic domain. The enzymatic domain can be part of:1. the receptor itself or a 2. cytosolic protein recruited upon receptor activation. Transmembrane Receptors with Linked Enzymatic Domains Structure: These receptors are single-membrane- spanning proteins, unlike the seven- membrane-spanning structure of GPCR They often form dimers or multi subunit complexes to transduce signals. Play roles in a diverse set of physiologic processes, including: 1. Cell metabolism 2. Growth 3. Differentiation Transmembrane Receptors with Linked Enzymatic Domains Mechanism: Receptors with linked enzymatic domains often modify proteins by adding or removing phosphate groups from specific amino acid residues. Classification: Five major classes based on their cytoplasmic mechanisms of action: 1. Receptor Tyrosine Kinases 2. Receptor Tyrosine Phosphatases 3. Tyrosine Kinase-Associated Receptors 4. Receptor Serine/Threonine Kinases 5. Receptor Guanylyl Cyclases Receptor Tyrosine Kinases (RTKs) The largest group of transmembrane receptors with enzymatic cytosolic domains. Transduce signals from many hormones and growth factors by phosphorylating tyrosine residues on the cytoplasmic tail of the receptor. Receptor Tyrosine Kinases (RTKs) MOA: 1. Binding of a specific ligand (such as a growth factor) to the extracellular domain of RTKs induces receptor dimerization (pairing of two receptor molecules). 2. Autophosphorylation: The dimerized receptors undergo autophosphorylation on specific tyrosine residues in their intracellular domains. 3. Activation of Signaling Pathways: The phosphorylated tyrosines serve as docking sites for various intracellular signaling proteins, leading to the activation of downstream signaling pathways like the MAPK, PI3K/Akt, and PLCγ pathways. 4. Regulation of Cellular Processes: including cell growth, differentiation, survival, and metabolism. 5. Role in Cancer: Dysregulation or mutation of RTKs is often associated with cancer and other diseases, making them important targets for therapeutic intervention. Receptor Tyrosine Kinases (RTKs) Insulin Receptor Structure: This receptor consists of: Two extracellular α subunits covalently linked to Two membrane-spanning β subunits Receptor Tyrosine Kinases (RTKs) Insulin Receptor Mechanism: 1. Insulin binds to the extracellular alpha subunits of the insulin receptor. 2. Upon insulin binding, the receptor undergoes dimerization and Type 2 diabetes autophosphorylation on specific tyrosine residues located on the mellitus may, in some cases, be associated intracellular beta subunits. with defects in 3. The phosphorylated tyrosine residues then act to recruit other post-insulin receptor cytosolic proteins, known as insulin receptor substrate (IRS) proteins. signaling 4. These pathways result in cellular responses such as increased glucose uptake ,glycogen synthesis, and overall regulation of glucose metabolism.