Midterm Learning Objectives PDF
Document Details
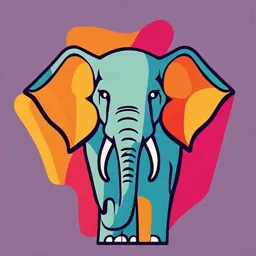
Uploaded by ZippyPelican
York University
Tags
Summary
This document presents midterm learning objectives for a biology course at York University, covering topics in basic physiology, evolution, and related concepts.
Full Transcript
MIDTERM LEARNING OBJECTIVES For the midterm you should be familiar with and understand the following topics and concepts. These are all taken directly from lectures and the discussion forums. The lecture slides are available to you on eClass. You can refer to the textbook (relevant chapters ind...
MIDTERM LEARNING OBJECTIVES For the midterm you should be familiar with and understand the following topics and concepts. These are all taken directly from lectures and the discussion forums. The lecture slides are available to you on eClass. You can refer to the textbook (relevant chapters indicated in the course schedule and links provided on eClass) for clarification and additional information, but you will only be tested on material from lectures and discussions. UNIT I: FOUNDATIONS OF PHYSIOLOGY I. INTRODUCTION TO PHYSIOLOGY - 6 physiological processes a. Unifying themes i. Integration 1. reductionism: Reductionism is a way of understanding complex systems by looking at their individual parts and how they function. a. If you want to understand how a car works, you might study the engine, transmission, brakes, and wheels separately. By learning how each part functions, you can gain insight into how the whole car operates. 2. Emergence: Emergence is when a group of simple parts comes together to create something new and complex that you can’t predict just by looking at the parts alone. a. ingredients like flour, sugar, eggs, and butter. Individually, these ingredients are just what they are. But when you mix them together and bake them, you get a cake. The cake has properties (like being sweet and fluffy) that you couldn’t predict just by looking at the individual ingredients. ii. Physics and chemistry set the limits 1. SA:V ratio - how efficiently cells can exchange materials based on size Materials exchange- The SA ratio is crucial because the rate at which substances (like nutrients and waste) are exchanged across cell membranes depends on a cell’s surface area, while the amount of material needing exchange relates to the volume. Smaller cells have a higher SA Rate of Exchange: As cells or organisms increase in size, their volume increases faster than their surface area, which can limit how efficiently they exchange materials. 2. Size and scale - how physical laws of physics and chemistry influence strength, and metabolic processes of animals as they grow in size Impact of Size: The discussion about how increasing size affects the strength of bones and the metabolic rates of animals relates to size and scale. Larger animals need proportionately thicker bones to support their greater mass due to the relationship between area (cross-sectional) and volume (mass). Metabolic Rate: The scaling of metabolic rates—where larger animals (like elephants) have lower metabolic rates relative to their size compared to smaller animals (like mice)—also fits into this point. This scaling illustrates how size affects physiological processes. iii. Form and function 1. Genotype; phenotype; environment a. Phenotype: This includes morphology (form), physiology (function), and behavior. The phenotype is what natural selection acts upon. b. Genotype: The genetic makeup (genotype) determines the phenotype. Over time, evolutionary changes result from interactions between genotype and environment, as organisms adapt to their surroundings. c. Natural Selection: The process of natural selection results in animals that are better adapted to their environments. This interaction highlights the relationship between genotype (what the organism inherits), phenotype (how it looks and behaves), and the environment (the conditions it lives in). iv. Evolution 1. Convergent and divergent evolution (analogous and homologous traits) - phenotypes and genotypes can be homologous or analogous a. Convergent -evolution occurs when different species develop similar traits independently, usually because they adapt to similar environments or challenges. (Analogous traits) Aquatic vertebrates like dolphins (mammals) and sharks (fish) have streamlined bodies and fins for efficient swimming. These similarities arise because both groups live in similar aquatic environments, not because they share a recent common ancestor. b. Divergent - two or more related species evolve different traits or behaviors, often because they adapt to different environments or niches. (Homologous traits) forelimbs of mammals show divergent evolution. For instance, the human arm, whale flipper, and bat wing have different functions (grasping, swimming, flying) but share a similar underlying bone structure due to inheritance from a common ancestor. v. Homeostasis -when our body keeps everything in balance, and functions properly regardless of what is going on inside or outside of our body. It helps maintain sugar and temperature. Our body uses negative feedback to alert us when something is wrong in our body for instance, when sugar levels are low or high. Our body will react to correct the imbalance and return it to the normal state. 1. Negative and positive feedback a. Negative feedback - Reduces deviation from setpoint Body Temperature: If the body temperature rises, mechanisms (like sweating) help cool it down. Blood Glucose Levels: If blood sugar levels rise, insulin is released to lower them back to normal. b. Positive feedback - Increases deviation from setpoint Childbirth: The release of oxytocin increases contractions, which in turn triggers more oxytocin release. Vomiting: The initial trigger can lead to a series of contractions that amplify the response. 2. Regulation: conformers and regulators a. Conformer - Allow internal conditions to change with external conditions. Cells must cope with these changes. Ex.Goldfish are ectothermic animals, meaning their body temperature changes with the water temperature. If you put a goldfish in warm water, its body heats up. If the water is cold, its body cools down. The goldfish does not actively regulate its temperature; it just adapts to the temperature of the water around it. b. Regulators - Maintain relatively constant internal conditions regardless of external conditions.This process requires considerable energy. Ex.Humans are regulators. Regardless of external temperature, they maintain a stable body temperature (around 98.6°F or 37°C) through processes like sweating when it's hot or shivering when it's cold. This regulation requires energy to achieve homeostasis despite environmental changes. 3. Phenotypic plasticity -The ability of an organism to alter its phenotype in response to environmental conditions. a. Types: Reversible Acclimation: Changes occur under controlled (laboratory) conditions with a single environmental factor. Ex.A laboratory experiment where scientists gradually increase the temperature of water for a fish species. The fish can adjust its metabolism and behavior to cope with the warmer temperature in a controlled setting. Acclimatization: Changes occur in the natural environment with multiple factors. Ex. A person moving from sea level to a high-altitude environment (like the Rocky Mountains) where the oxygen levels are lower. Over time, their body adjusts by producing more red blood cells to enhance oxygen transport, adapting to the multiple changes in altitude, temperature, and pressure in a natural setting. Irreversible Polyphenism: Development under different conditions results in alternative phenotypes in the adult organism. ○ Example: Differences in traits based on environmental factors, like variations in IQ in children. vi. Circadian control 1. Circadian control - Physiology that follows a roughly 24-hour cycle, influenced by regular and predictable environmental changes.This means that many physiological activities, such as sleeping, eating, and hormone release, occur at predictable times each day, influenced by external environmental cues. a. Light/Dark cycle Daytime: When it's light, the body may be more alert, with processes like increased metabolism, heightened hormone production (like cortisol), and active behaviors. Night Time: When it gets dark, the body prepares for rest, releasing melatonin (a hormone that promotes sleep) and reducing alertness If someone constantly goes to bed at 10 PM and wakes up at 6 AM, their body learns to expect sleep during those hours due to the light/dark cues from their environment. b. Seasons: As seasons change, so do the lengths of day and night, which can alter physiological processes Spring/Summer: Longer days might lead to increased activity levels, reproduction, and metabolic rates. Fall/Winter: Shorter days can trigger changes such as increased sleepiness and changes in appetite. Animals like bears may hibernate in winter when days are shorter and food is scarce, adjusting their physiological processes to conserve energy. II. CELL MEMBRANES AND CELL PHYSIOLOGY a. Fluid mosaic model -The fluid mosaic model describes the structure of the plasma membrane, and the various components that make it functional. i. Phospholipids - Form the basic structure of the membrane, creating a bilayer with hydrophilic heads facing outward and hydrophobic tails facing inward. ii. Proteins -Integral and peripheral proteins are embedded within or associated with the phospholipid bilayer, contributing to membrane function. 1. Peripheral -associated with signaling and temporary interactions. 2. Integral -functioning as transport channels or receptors. iii. Carbohydrates -Attached to proteins or lipids, they play a key role in cell recognition and communication. 1. Glycolipids -Carbohydrates covalently bonded to lipids, involved in cell-cell recognition. 2. Glycoproteins - Carbohydrates covalently bonded to proteins, important for signaling and immune response. b. Crossing the membrane i. Passive - Movement of substances across a membrane without the use of energy, following the concentration gradient (from high to low concentration) 1. Diffusion -moving from areas of higher concentration to lower concentration until equilibrium is reached. Ex. Lipids, oxygen (O₂), carbon dioxide (CO₂), and small uncharged molecules (like ethanol) can pass freely across the membrane through passive diffusion. a. Osmosis -movement of water across a semi-permeable membrane, from an area of low solute concentration to an area of high solute concentration. Water moves into a cell placed in a hypotonic solution, causing the cell to swell. b. Tonicity Isotonic -A solution with the same solute concentration as inside the cell, resulting in no net movement of water. A cell in an isotonic solution remains normal in size. Hypotonic - A solution with a lower solute concentration than inside the cell, causing water to move into the cell, which may lead to swelling and may burst (lysis). cells may swell or burst Hypertonic- A solution with a higher solute concentration than inside the cell, causing water to move out of the cell, leading to cell shrinkage (crenation) 2. Facilitated diffusion (facilitated transport) -Facilitated diffusion is a type of passive transport where specific molecules move across the cell membrane with the help of transport proteins. This process does not require energy because it occurs along the concentration gradient (from high to low concentration). a. Ex.Glucose transport into cells often occurs via a protein called GLUT. Since glucose is polar and cannot easily pass through the lipid bilayer, it binds to the GLUT transporter, which then changes shape to allow glucose to enter the cell ii. Active - Active transport is the movement of molecules across a cell membrane against their concentration gradient (from low to high concentration) and requires energy, usually from ATP. 1. Primary -This process directly uses ATP to transport solutes across the membrane. a. Na⁺/K⁺ pump (Na⁺/K⁺ ATPase) actively transports 3 sodium ions (Na⁺) out of the cell and 2 potassium ions (K⁺) into the cell. This creates a concentration gradient and an electrochemical gradient, which is crucial for nerve impulses and muscle contractions. 2. Secondary - uses the energy stored in the concentration gradient established by primary active transport to move other molecules against their gradient. a. Na⁺/glucose transporter uses the gradient created by the Na⁺/K⁺ pump. As sodium ions go back into the cell down their concentration gradient, they carry glucose with them into the cell against its gradient. c. Bulk transport -This is when a cell takes in substances from outside by wrapping its membrane i. Endocytosis - Cell "takes in" substances (like food or fluids) by forming vesicles. 1. Types: a. Phagocytosis: A type of endocytosis where cells take in large particles or whole cells (e.g., by unicellular organisms or immune cells like macrophages). Known as “cell eating” b. Pinocytosis: The uptake of fluids and solutes via small vesicles.Known as “cell drinking”. It’s how cells take in small amounts of fluid and dissolved nutrients. ii. Exocytosis - Cell "pushes out" substances (like hormones or neurotransmitters) by releasing them from vesicles. 1. Neurotransmitter Release: When a nerve cell (neuron) sends a signal, it releases neurotransmitters into the space between nerve cells (the synapse) using exocytosis. The vesicles carrying the neurotransmitters move to the membrane, fuse with it, and dump their contents outside. 2. Insulin Release: In the pancreas, beta cells produce insulin (hormone). When blood sugar levels rise, these cells use exocytosis to release insulin into the bloodstream. d.Multicellular specializations i. Extracellular matrix proteins -The ECM is a network of proteins and other molecules outside cells that provides structural support and helps bind cells together. (Example: Think of the ECM as the "glue" that holds a building together. Without it, the cells (like bricks) wouldn't stay connected or function properly.) 1. Collagen: Provides strength and structure (like a framework). 2. Elastin: Allows tissues to stretch and return to their original shape (like elastic bands). 3. Hyaluronan: Keeps tissues hydrated and helps with cell movement. ii. Transcellular and paracellular pathways 1. Transcellular pathway -Movement of substances through the cells themselves Example: In gut epithelial cells, glucose is transported from the gut lumen (inside the intestines) into the bloodstream. This involves: a. Na+-glucose transporter: this transporter uses the sodium gradient (high concentration of sodium outside the cell) to bring glucose into the cell along with sodium ions. b. GLUT2 transporter: Moves glucose from inside the cell to the extracellular space that goes into the (bloodstream). 2. Paracellular pathway -Movement of substances between the cells (the spaces in between). a. In leaky epithelial tissues, such as those lining the intestines, water and small ions can easily pass through the gaps between cells. UNIT II: CHEMICAL COMMUNICATION BETWEEN CELLS III. CELL-CELL COMMUNICATION a. Direct and indirect i. Direct -Direct signaling involves communication between cells that are physically connected. a. Gap Junctions: Signaling and target cells are connected by gap junctions, allowing direct transfer of signaling molecules from one cell's cytoplasm to another's. b. Example: A wave of depolarization in nerve cells can directly affect adjacent cells. c. Contact-Dependent Signaling: The signaling molecule is attached to the membrane of the signaling cell. It binds to a receptor on the target cell without needing to be released into the environment. ii. Indirect-release of chemical messengers that travel to their target cells. a. Chemical Messenger: The signaling cell releases a molecule into the extracellular environment. b. Transport: This molecule travels through the extracellular fluid to reach the target cell. c. Receptor Binding: The messenger binds to a receptor on the target cell, activating a response. Chemical signaling iii. Autocrine-a cell sends out signals that it can also respond to, which can lead to uncontrolled growth, especially in cancer cells. (cell signals itself) iv. Paracrine -a cell releases molecules that only affect nearby cells, helping them communicate and respond to each other quickly. (cell signals nearby cells) v. Endocrine -hormones are made by special cells and released into the bloodstream to affect cells that are far away in the body. vi. Pheromones- chemicals they release into the air, to communicate with each other. These chemicals can help mark paths to food, set boundaries for their territory, warn others about dangers, and attract mates. vii. Neuronal -nerve cells send fast electrical signals along long fibers (axons) to communicate with other cells far away. b. Chemical classes of signal molecules -enable flexible, efficient, and diverse communication within the body, essential for maintaining homeostasis and responding to various stimuli. i. Peptides -Peptides are small proteins made of 2 to 200 amino acids, produced in the rough endoplasmic reticulum, stored in vesicles, and released into the body where they dissolve in fluids, bind to cell surface receptors, and quickly trigger responses in target cells. a. (e.g. oxytocin) - stimulates uterine contractions during labor , promotes milk ejection from breasts. Used for reproductive ii. Steroids -Steroid hormones, made from cholesterol in the smooth endoplasmic reticulum or mitochondria, include types like mineralocorticoids (for electrolyte balance), glucocorticoids (stress hormones), and reproductive hormones, and they are hydrophobic, meaning they can pass through cell membranes, bind to receptors inside cells, and have slower effects on gene activity a. (e.g. estrogen) -Helps control the menstrual cycle and ovulation., breast development, support bone density (reproductive hormone) iii. Biogenic amines -Biogenic amines, which include substances like acetylcholine and catecholamines, contain an amine group and can act as hormones or neurotransmitters, with most being water-soluble except for thyroid hormones, and they have a wide range of effects in the body. a. (e.g. norepinephrine) - responding to stressful situations iv. Eicosanoids - like prostaglandins, act locally (as paracrines) in processes such as inflammation and pain; a. (e.g. prostaglandins) v. Purines -adenosine and ATP, function as communicate (neuromodulators) and act between nearby cells (paracrines) a. (e.g. adenosine) vi. Gases -send signals between nearby cells. a. (e.g. nitric oxide, NO) -relax blood vessel c. Receptor types i. Intracellular -receptors are inside the cell and bind to molecules that can pass through the cell membrane (like hormones). When they bind, They help control gene expression, affecting traits like growth or metabolism. 1. Single Transcription Factor: A transcription factor is a protein that helps turn genes on or off. One transcription factor can activate a lot of different genes at once. 2. Genes and Phenotypes: Many genes work together to create specific traits or characteristics in an organism, known as a phenotype (like eye color or height). 3. Transcription Beyond Hydrophobic Ligands: While some transcription factors respond to hydrophobic (fat-soluble) molecules, there are also other ways to change gene activity that don’t involve these types of ligands. ii. Ligand-gated ion channel -open or close in response to specific signals (ligands). When they open, ions can flow in or out of the cell, causing quick changes in the cell's electrical charge. 1. Binding: A signaling molecule (ligand) attaches to a specific receptor on the cell’s surface. 2. Shape Change: This binding causes the receptor to change shape. 3. Channel Opens: The shape change opens a channel in the membrane. 4. Ion Movement: Ions (like sodium or potassium) flow through this channel into or out of the cell. 5. Membrane Potential Change: The movement of these ions changes the electrical charge inside the cell, affecting how the cell functions. iii. Receptor enzymes-When a signaling molecule binds to them, they trigger chemical reactions inside the cell, changing cell behavior, like metabolism 1. Binding: A signaling molecule (like insulin) attaches to the receptor on the cell surface. 2. Activation: This binding activates the enzyme part of the receptor. 3. Phosphorylation Cascade: The enzyme starts adding phosphate groups to specific proteins inside the cell. 4. Cell Response: This phosphorylation changes the activity of those proteins, leading to changes in the cell's behavior. iv. G-protein coupled- activates special proteins called G-proteins when a signaling molecule binds to them. This activation leads to various changes in the cell’s activities and functions. 1. Binding: A signaling molecule (ligand) attaches to a receptor on the cell's surface. 2. Activation: This binding causes the receptor to interact with G-proteins inside the cell. 3. G-Protein Activation: The G-protein splits into different parts (subunits) that move and activate other proteins in the membrane. 4. Second Messengers: These activated proteins create second messengers (like cAMP) that carry signals inside the cell. d. Cellular signaling pathways i. Water-soluble – membrane-bound receptor - This mechanism is used for rapid responses, like increasing blood sugar levels during stress by breaking down glycogen. 1. Epinephrine binds to receptors on the liver cell membrane, activating messenger molecules that trigger enzyme activity, leading to the breakdown of glycogen and release of glucose. a. gives quick energy to react fast during stress for instance when we get scared of seeing a snake or insects 2. Ligand-gated ion channels and receptor-enzymes act through membrane-bound receptors to create quick cellular responses. ii. Water-insoluble – cytosolic/nuclear receptor -This mechanism is used for longer-term changes, such as regulating growth, development, and metabolism by altering gene expression. (Slower responses) 1. Lipid-soluble hormones (like steroid hormones) diffuse through the cell membrane, bind to receptors in the cytoplasm or nucleus, and change gene expression. a. Example: Estrogen, Think about puberty when your body starts changing. Estrogen, a hormone, enters your cells easily because it's fat-soluble.Inside the cell, estrogen binds to its receptor, which then goes into the cell's nucleus. Here, it helps turn on genes that lead to the development of breasts and wider hips. This process takes longer but results in important physical changes 2. The hormone-receptor complex moves into the nucleus to regulate target genes. e. Hormone action i. Ligand-receptor interactions -Only target cells with specific receptors respond to hormones. The response strength depends on hormone levels, receptor numbers, and how well the hormone binds to the receptor. More receptors lead to a stronger response, and receptors can be up-regulated or down-regulated based on the cell's needs. IV. INTRACELLULAR SIGNALLING a. GPCR signaling -GPCR (G-Protein-Coupled Receptor): This is a type of receptor on the cell membrane that, when activated by a signaling molecule (like a hormone), undergoes a shape change. i. Activation: GDP → GTP a. In its inactive state, a G-protein attached to the GPCR has a molecule called GDP (guanosine diphosphate) bound to it. When the GPCR is activated, it causes the G-protein to release GDP and bind to GTP (guanosine triphosphate). This switch from GDP to GTP activates the G-protein. ii. Inactivation: GTP hydrolysis and GRS proteins 1. GTP Hydrolysis: The active G-protein (now with GTP) can interact with other proteins in the cell to initiate a response. However, it will eventually hydrolyze the GTP, breaking it down to GDP. This inactivates the G-protein and stops its signaling activity. 2. GRS Proteins: GRS (GTPase-activating proteins) can help speed up the process of GTP hydrolysis, further ensuring that the signaling pathway is turned off efficiently. These proteins promote the inactivation of the G-protein, helping to maintain balance in cellular signaling. b. Adenylate cyclase i. cAMP production 1. When a signaling molecule binds to a G-protein-coupled receptor, it activates adenylate cyclase. 2. cAMP binds to the regulatory subunits of protein kinase A (PKA). 3. PKA then adds phosphate groups to other enzymes, altering their activity (activation or inhibition). ii. cAMP broken down by cAMP phosphodiesterase -stopping the signaling pathway. a. Example: after adrenaline (epinephrine) triggers a quick response in the body—like increasing heart rate or breaking down glycogen—cAMP levels rise to carry that signal. Once the immediate effect is no longer needed, cAMP phosphodiesterase acts to degrade cAMP. This ensures that the body can return to its normal state iii. e.g. adrenaline; αs -When you experience stress, adrenaline (also known as epinephrine) is released. It activates the cAMP pathway, which helps to quickly mobilize energy by promoting the breakdown of glycogen into glucose, providing immediate fuel for your body. iv. e.g. prostaglandin; αi -can also activate the cAMP pathway, influencing processes such as pain signaling and regulating blood flow, depending on the specific type of prostaglandin and its target cells. a. Example: For instance, during menstrual cramps, the body produces prostaglandins that can cause contractions in the uterus. This contraction can lead to pain and discomfort, as the prostaglandins activate the cAMP pathway in nearby cells, which sends pain signals to the brain and regulates blood flow in the area c. PKA- protein kinase A - increasing or decreasing their enzymatic activities within seconds. i. 4 cAMP bind to activate ii. Fast response - seconds to minutes 1. Phosphorylates other kinases a. Protein kinase A adds a phosphate group to various enzymes, which quickly changes how active those enzymes are - making them work faster. iii. Slow response - minutes to hours 1. Phosphorylation of CREB a. In a slow response that takes minutes to hours, the enzyme PKA adds a phosphate group to a protein called CREB in the cell nucleus. Once CREB is activated, it binds to another protein called CBP and together they attach to a specific DNA region called CRE. This process starts the production of certain genes, leading to changes in the cell's activities over time. 2. CREB → CBP → CRE → transcription a. This means that when CREB (a protein) gets activated, it connects to another protein called CBP. Together, they then attach to a specific DNA sequence called CRE. This connection helps start the process of making new proteins by triggering transcription, which is how DNA is turned into RNA. CBP (CREB-Binding Protein) is a co-activator that helps CREB enhance the transcription of target genes. When CREB binds to the CRE sequence in DNA, CBP joins in to strengthen that connection, facilitating the transcription process. CRE (cAMP Response Element) is a specific DNA sequence that CREB recognizes and binds to. This sequence is found in the promoter region of certain genes and is essential for the regulation of those genes in response to signals like cAMP iv. Serine/threonine phosphatases inactivate phosphorylated proteins 1. This process helps turn off the signaling pathways by removing phosphate groups from proteins that were previously activated. v. Pathogenic mechanisms 1. Cholera toxin a. Cholera toxin from the bacteria Vibrio cholerae changes a protein called Gαs, which normally helps turn off a signal. Because of this change, the signal stays active and causes levels of a messenger called cAMP to rise in intestinal cells. This increase in cAMP opens channels that let chloride ions (Cl⁻) flow out of the cells. As a result, a lot of chloride and water leave the cells and enter the gut, leading to severe diarrhea. V. ENDOCRINE SYSTEMS a. Endocrine cells and glands i. Developmental origins 1. Endocrine glands develop from ectodermal tissue, forming clusters of cells that connect to blood vessels during development. ii. Distribution 1. Mammals a. Includes the pituitary gland, thyroid gland, adrenal glands, and pancreas. Some endocrine cells are also found scattered in organs like the pancreas and intestines. b. Positive and negative feedback regulation i. Prolactin and oxytocin a. Prolactin: Promotes milk production in mammals. b. Oxytocin: Triggers uterine contractions during childbirth and milk release during breastfeeding. It works through positive feedback. ii. Insulin and glucagon a. insulin- Decreases blood glucose by promoting glucose uptake and fat storage b. Glucagon: Produced by pancreatic alpha cells; it raises blood glucose levels by converting glycogen to glucose and promoting fat breakdown. c. Homeostasis: Together, these hormones maintain glucose levels through negative feedback loops. 2. Diabetes - This means that in a condition like diabetes, the body either doesn't make enough insulin or the insulin it makes doesn't work properly. Insulin is a hormone that helps move sugar from the blood into the cells to be used for energy. When there's not enough insulin or it's not effective, sugar stays in the blood, causing high blood sugar levels. This can lead to various health problems if not managed. This leads to high blood sugar levels. a. Type 1-The body's immune system mistakenly attacks and destroys the cells in the pancreas that make insulin (called beta cells). Since the body can't produce insulin, people with Type 1 need to take insulin from outside (like through injections) to manage their blood sugar levels. b. Type 2- The body either doesn’t produce enough insulin or the insulin doesn’t work well because the cells don’t respond to it properly (often due to changes in the receptors). People with Type 2 may manage their diabetes with lifestyle changes (like diet and exercise), medication, or sometimes insulin if needed. c. Major mammalian endocrine glands i. Pituitary – master endocrine gland - produces numerous hormones 1. Anterior pituitary- produces hormones (TSH -Thyroid-stimulating hormone, ACTH - Adrenocorticotropic hormone) 2. posterior pituitary - releases hormones like oxytocin and ADH - antidiuretic hormone ii. Thyroid – metabolism, growth and development a. Regulates metabolism, growth, and development by producing thyroid hormones. iii. Adrenal hormones and stress response 1. Short term stress response- When you face something stressful, like being chased by a lion, your nervous system triggers the release of hormones like epinephrine (adrenaline) and norepinephrine (noradrenaline). These hormones prepare your body for quick action, like running away or fighting. This is often called the "fight-or-flight" response. 2. Long-term stress response: In situations where stress lasts longer (like during exam periods), another hormone called adrenocorticotropic hormone (ACTH) is released. This hormone signals the adrenal cortex to release corticosteroids, which help manage stress over a longer time by regulating things like metabolism and immune response. iv. Pineal gland and biorhythms a. Melatonin-Melatonin is a hormone made by the pineal gland in the brain. It comes from the amino acid tryptophan, which is turned into serotonin first. Melatonin helps regulate biological rhythms, which include our daily sleep-wake cycle (circadian rhythms) and processes related to reproduction. The release of melatonin is controlled by a part of the brain called the suprachiasmatic nucleus (SCN). This area senses light and dark in our environment. Melatonin production increases when it gets dark, helping us feel sleepy, and decreases when it’s light, helping us wake up. v. Hormones and behaviour a. Hormones can influence behavior by affecting how organisms respond to stimuli. Low Estrogen Levels: When female rats have low levels of estrogen, they don’t respond to touches from male rats. This means they aren’t ready to mate. High Estrogen Levels: As the eggs mature, estrogen levels go up. When this happens, if a male touches the female’s back, she responds by adopting a specific posture called lordosis. This position is crucial for successful mating. Circadian Rhythm: Hormone levels in the body follow a daily cycle (circadian rhythm). This means that hormone levels rise and fall at certain times, influencing behaviors like mating and readiness to respond to stimuli. UNIT III: ELECTRICALL COMMUNICATION VI. NERVOUS SYSTEMS a. Evolution and comparative animal nervous systems i. Vertebrates 1. CNS and PNS a. Central Nervous system composed of the brain and spinal cord spinal cord processes the reflex action ventricles -fluid-filled spaces in the brain that help produce and contain CSF. CSF is a special fluid that fills these ventricles and surrounds the brain and spinal cord. functions of CSF: Mechanical protection: It acts like a cushion, helping to absorb shocks and keep the brain floating, which protects it from injury. Chemical protection: CSF helps maintain a healthy environment for brain cells by providing the right balance of chemicals (like ions) and supports the blood-brain barrier (BBB). Circulation: It allows for a small exchange of nutrients and waste products between the blood and nervous tissue, helping keep everything running smoothly. White matter vs Gray matter White Matter: This part of the brain and spinal cord is made up of myelinated axons, which are long nerve fibers covered in a fatty substance called myelin. This myelin gives it a white appearance. White matter helps signals travel quickly between different parts of the nervous system.(process information) Ex. muscle control→ sensory perception (seeing, hearing) , decision making and emotions Gray Matter: This part contains neuronal cell bodies (the main part of nerve cells), dendrites (branch-like structures that receive signals), axon terminals (where signals are sent out), unmyelinated axons (nerve fibers that aren't covered in myelin), and neuroglia (supporting cells). Gray matter looks grayish and is where most of the processing and signal integration happens.(sends information) connects different parts of white matter to help them communicate Meninges - protective layers that cover the brain and spinal cord. has 3 layers - protects brain from infections 1.Pia mater: This is the innermost layer, sitting right on top of the brain and spinal cord. It's thin and delicate and has blood vessels that supply nutrients. 2.Arachnoid matter: This is the middle layer. It has a web-like structure that looks like spider webs. Between the pia mater and the arachnoid mater is a space called the subarachnoid space, which is filled with fluid that cushions the brain. 3.Dura mater: This is the outermost layer. It's thick and tough, providing the strongest protection. It connects to the outer layer of nerves, called the epineurium. Blood Brain barrier (BBB) - BBB helps protect the brain by controlling what can enter and ensuring it gets what it needs to function well. Tight junctions: These are strong seals between the cells in the blood vessels, preventing most proteins from getting into the brain. Slow passage for ions: Ions (charged particles) can cross, but very slowly. Easy passage for some things: Oxygen, carbon dioxide, and certain hormones can pass through without trouble. Glucose transport: Glucose, which the brain uses for energy, gets in quickly through special helpers. Other substances: Things like alcohol, nicotine, and caffeine can also get through easily. Cerebellum - Evaluates and coordinates movement, balance, and may play a role in cognitive processes. brainstem - Connects the brain to the spinal cord and controls vital functions like breathing and heart rate. Telencephalon-This part includes the cerebral cortex, which is responsible for higher-level functions like thinking, decision-making, and processing sensory information Diencephalon-Processes sensory and motor information; includes the thalamus, hypothalamus, and epithalamus. Mesencephalon -This is the upper part of the brainstem that connects the pons and cerebellum with the front part of the brain. Thalamus - It sits in the middle of the brain, on either side of a space called the third ventricle. The two sides of the thalamus are connected by a bridge called the interthalamic adhesion. acts like a traffic hub for sensory information. Before this information reaches the cerebral cortex (the part of the brain responsible for processing thoughts, actions, and sensations), it gets filtered and processed by the thalamus. Sensory relay station - It handles most senses—like touch, taste, sight, and hearing—but not smell (which goes directly to a different part of the brain). The thalamus also plays a role in movement by connecting to other areas of the brain, such as the cerebellum (which helps coordinate movement) and the basal nuclei (which are involved in controlling movement). hypothalamus - a small but important part of the brain located just below the thalamus. wraps around the third ventricle. The hypothalamus connects to the pituitary gland (often called the "master gland") through a thin stalk called the infundibulum. This connection is crucial for hormone regulation. The hypothalamus is like a control center that helps maintain homeostasis, which means keeping the body stable and balanced. It monitors: Temperature: Helps keep your body temperature just right. Hunger: Signals when you’re hungry or full. Thirst: Tells you when you need to drink. hormone production - produces several hormones that control functions like growth, metabolism, stress response emotions- It also integrates feelings and drives, influencing emotions like happiness, anger, and stress. epithalamus - It sits at the back of the brain, capping the posterior part of the third ventricle, which is a fluid-filled space in the brain. pineal gland - produces and releases melatonin, a hormone that helps regulate sleep. limbic system - play a key role in emotions and memory Emotions: It helps regulate emotions like anger and fear. Memory: It's crucial for forming new memories and recalling past experiences. Olfaction: It also plays a role in the sense of smell. Key parts: Amygdala: Involved in processing emotions, especially fear and anger. Hippocampus: Important for learning and forming new memories. Mammillary bodies: Help with memory recall. Fornix: A bundle of fibers that connects different parts of the limbic system. White matter of cerebrum - Association fibers (green): These fibers connect areas within the same half of the brain (hemisphere). They help different parts work together, like coordinating functions within one side. Commissural fibers (red): These fibers connect the left and right hemispheres of the brain, allowing them to share information. The largest of these is called the corpus callosum. Projection fibers (blue): These fibers connect the cerebrum to lower parts of the brain and the spinal cord. They send signals to and from the cerebrum, helping with overall communication throughout the body. Corpus callosum - The corpus callosum is a thick band of nerve fibers in the brain that connects the left and right sides. Think of it as a bridge that allows the two halves of the brain to communicate with each other. It has about 300 million axons (nerve fibers), which help share information quickly between the left and right hemispheres. This connection is important for coordinating activities that involve both sides of the body and for integrating functions like language, movement, and sensory information. Cerebral cortex- outermost layer of the brain, made of gray matter and often called the neocortex It has many folds (called gyri) and grooves (called sulci). These folds help fit a lot of neurons into a small space, allowing for more complex brain functions. Different areas of the cortex are responsible for specific tasks, like sensing touch, controlling movement, or processing information. Scientists have created a map (Brodmann’s map) to show which parts of the cortex handle different functions. This helps us understand how the brain is organized and how it works. primary motor cortex -located in the precentral gyrus of the frontal lobe This area contains large motor neurons that help control movement. It sends signals to the spinal cord, which then relays these signals to the muscles on the opposite side of the body (the right side of this area controls movements on the left side of the body, and vice versa). There’s a map called the motor homunculus that shows which parts of the motor cortex control different muscles. Some areas, like the hands and face, have more representation because they need more precise control, even though they take up less space compared to larger muscles like those in the legs. primary somatosensory cortex- found in the postcentral gyrus of the parietal lobe. It receives information about touch and sensations from the body, like pressure, temperature, and pain. It processes sensations from the opposite side of the body (the right side of this area gets signals from the left side of the body, and vice versa). There’s a map called the sensory homunculus that shows which parts of this area correspond to sensations from different body parts. This map helps us understand how sensitive different areas of our body are. primary visual cortex - It receives visual information from what we see.It processes images from the opposite visual field, meaning the right side of this area processes what you see on your left side, and the left side processes what you see on your right side. This helps us understand and interpret the visual world around us. primary auditory cortex- It receives sound information from both ears, allowing us to hear.It has a tonotopic organization, which means that different areas of this cortex respond to different pitches or tones of sound, like a piano where each key produces a different note. This helps us recognize various sounds, like music or speech. association cortices - help us combine information to understand and respond to our environment better. Movement planning and rehearsal: They help us plan and practice movements before we actually do them. Pattern recognition: They allow us to recognize patterns, like faces or objects, by connecting different pieces of information. Comprehension: They help us understand language and situations. The prefrontal cortex is a special part of the association cortices responsible for higher functions, like personality, complex thinking, decision-making, and planning for the future. broca’s area - It's located in the motor association cortex, usually in the left hemisphere (the side most people use for language). Broca's area helps control the muscles involved in speaking, including those for breathing and vocalization. This means it plays a key role in how we form words and speak normally. b. Peripheral nervous system - The PNS is made up of nerves that connect the brain and spinal cord to the rest of the body.it carries messages to and from the body Types of nerves: Cranial nerves There are 12 pairs of cranial nerves that come directly from the brainstem. Example: The optic nerve (for vision) is a cranial nerve. sends information from eyes to your brain vision, smell, taste, hearing, and facial movements Spinal Nerves There are 31 pairs of spinal nerves that emerge from the spinal cord. Example: The sciatic nerve, which runs down the leg, is a spinal nerve. Sciatic Nerve: This is a spinal nerve that runs down from the lower back through the legs. If you experience pain in your leg (often called sciatica), it's because the sciatic nerve is sending pain signals from your leg back to your brain. Sensory Information: They carry signals from the body to the brain. For example, if you touch something hot, sensory nerves in your skin send a message to your spinal cord and then to your brain, telling it that the object is hot. Motor Commands: They send signals from the brain to the muscles. For instance, if you decide to lift your arm, the brain sends a signal through the spinal nerves to the muscles in your arm, telling them to move. 2. ANS - Autonomic nervous system - controls involuntary functions in the body. It works automatically without us needing to think about it. Components: sympathetic Fight or Flight Response: Activates during stressful situations. It prepares the body for action by increasing heart rate, dilating pupils, and slowing digestion. Ex. Autonomic reflex: gets activated during stress or exercise parasympathetic Rest and Digest: Works when the body is relaxed. It slows the heart rate, promotes digestion, and conserves energy. Ex.After a big meal, your body enters a "rest and digest" state. The parasympathetic nervous system slows your heart rate, increases digestive enzyme production, and helps your body absorb nutrients effectively. This allows you to relax and focus on digesting your food. b. Sensory - This part carries signals from the body to the brain, helping us sense things like touch, pain, and temperature. When you touch something hot, sensory nerves send a signal to your brain saying "ouch!" c. ANS – Sympathetic and Parasympathetic Sympathetic: -Prepares your body for action during stressful situations, often called the "Fight or Flight" response. Uses norepinephrine; associated with the "Fight or Flight" response to help speed things up Parasympathetic: -Helps your body relax and recover, known as the "Rest and Digest" response. Uses acetylcholine; associated with the "Rest and Digest" response, to help calm things down Stress response Adrenal Glands: Produce hormones involved in stress responses, including: 1.Adrenal Medulla: Releases epinephrine (adrenaline) and norepinephrine (noradrenaline) for short-term stress responses. ○ immediate boost of energy and alertness ○ Example: If you need to run from danger, these hormones help you react quickly by increasing your heart rate and blood flow to your muscles. 2.Adrenal Cortex: Releases glucocorticoids and mineralocorticoids, involved in long-term stress responses. ○ regulating metabolism and blood pressure. ○ Example:If you're stressed for a long time (like during exams), the adrenal cortex helps keep your energy levels steady and manages other body functions. d. Motor -This part sends signals from the brain to muscles and glands, allowing us to move or respond. Voluntary - Controls movements we choose to make, like moving our arms or legs. Example: Deciding to pick up a cup. Involuntary - Controls automatic functions we don’t think about, like our heart beating or food moving through the digestive system. Your heart beats faster when you exercise. b. Overview of nervous system function i. Cells of the nervous system 1. Neurons - specialized cells that send and receive signals in nervous system (messengers) a. Example: Think of neurons as the messengers of the nervous system. For instance, when you touch something hot, sensory neurons send a signal to your brain, prompting you to pull your hand away. 2. Glial cells - support and protect neurons and maintain the environment for proper function. CNS Glial cells: a. Astrocytes: Provide structural support, form a barrier between blood vessels and neurons, supply oxygen and nutrients. makes sure neurons have what they need b. Oligodendrocytes: long parts of neurons that send signals. Myelin helps speed up signal transmission. c. Microglial Cells: They act like the immune cells of the brain, cleaning up waste and fighting infections d. Ependymal Cells: produce cerebrospinal fluid (CSF), which cushions the brain and spinal cord. PNS Glial cells Schwann Cells: which help signals travel quickly. Satellite Cells: Provide structural and nutritional support in ganglia. ii. Functional properties of neurons 1. Broca’s Aphasia: -affects how a person speaks a. Trouble forming complete sentences. They might only say one word at a time or struggle to put words together. For example, instead of saying, "I want to go outside," they might just say, "Go." Due to damage in the brain regions responsible for speech production (typically in the left hemisphere). b. Despite these difficulties, they can read and understand spoken language, indicating that their comprehension abilities remain intact. c. Often results from a stroke affecting the areas of the brain that control language, specifically Broca's area. VII. ELECTRICAL SIGNALING BY NEURONS: ACTION POTENTIALS a. Resting membrane potential - This is the normal electrical charge of a neuron when it’s not sending a signal, usually around -70 mV. Example: Think of it like a battery that’s fully charged and ready to work, but not in use yet. i. Equilibrium potential -Each type of ion (like sodium or potassium) has a specific charge level where it’s balanced inside and outside the cell. Example: Imagine balancing a seesaw. When both sides are equal, it’s stable. That’s how each ion feels at its equilibrium. ii. Nernst and Goldman equations - These are mathematical formulas used to calculate the ideal charge level for each ion and the overall charge of the neuron. 1. Think of them as recipes that tell you how to mix ingredients (ions) to get the perfect dish (membrane potential). b. Graded potentials - This refers to how graded potentials can weaken as they spread along the neuron. i. Depolarization -When the neuron’s charge becomes more positive, often because sodium ions rush in. 1. It’s like filling a balloon with air. As you blow into it, it expands (becomes more positive). ii. Hyperpolarization -When the neuron’s charge becomes more negative, usually due to potassium ions moving out. 1. Imagine a balloon losing air. It shrinks and becomes less inflated (more negative). iii. Passive (cable) properties of neurons - This refers to how graded potentials can weaken as they spread along the neuron. c. Action potentials -Rapid changes in a neuron’s charge that happen when it reaches a certain threshold, allowing the neuron to send a signal. i. Voltage-gated ion channels -Special doorways in the neuron’s membrane that open when the charge changes, allowing specific ions to enter or leave 1. Imagine a gated community where the gate opens only when a special password (the right charge) is said. ii. Action potential generation - The process of creating an action potential involves opening sodium channels first, then potassium channels. 1. It’s like a roller coaster; once you go over the first drop (threshold), you’re committed to the ride (action potential). iii. Propagation of action potentials -Action potentials (APs) can only move in one direction along the neuron. (Resting state, threshold , depolarization, action potential peak, repolarization, hyperpolarization, resting state , propagation) resting state -The axon is at rest, with a negative charge inside compared to the outside (due to sodium and potassium ions). The membrane potential is around -70 mV. K+ leak channels chronically open, therefore K+ flow out leaving inner face of membrane slightly electronegative. Threshold Trigger: When a stimulus (like a neurotransmitter) is strong enough, it causes sodium channels to open, allowing sodium ions to rush into the neuron. This makes the inside of the axon more positive, reaching a threshold. Depolarization: Once the threshold is reached, more sodium channels open, and the inside of the axon becomes positively charged. This is called depolarization. Action Potential Peak: The action potential peaks when the inside of the axon is about +30 mV. At this point, sodium channels close and potassium channels open. Repolarization: Potassium ions flow out of the axon, causing the inside to become negative again. This is called repolarization. Hyperpolarization: The outflow of potassium may cause the membrane potential to drop below the resting level for a brief moment (hyperpolarization). Return to Resting State: Eventually, the sodium-potassium pump helps restore the original ion balance, bringing the axon back to its resting state. Propagation: The influx of sodium ions during depolarization causes nearby sodium channels to open, creating a chain reaction. This allows the action potential to move along the axon, segment by segment, without losing strength. Refractory periods- After an action potential, there’s a short time when the neuron can’t send another signal (absolute refractory) or it’s harder to send one (relative refractory). 2. Hodgkin Cycle: This is a process that helps keep regenerating the AP as it moves along the axon. It works well for sodium (Na+) channels but not for potassium (K+) channels, meaning sodium channels help continue the signal more effectively. 3. Myelination- Some neurons are covered with a fatty layer called myelin, which helps signals travel faster. a. Myelin is a fatty layer made by cells called Schwann cells in the peripheral nervous system (PNS) or oligodendrocytes in the central nervous system (CNS). This layer wraps around the nerve's long part, called the axon. b. Saltatory Conduction Nodes of Ranvier: Myelin doesn’t cover the entire axon. There are gaps in the myelin called Nodes of Ranvier where the axon membrane is exposed. There are special channels that allow electrical signals to jump from one node to the next. This jumping is called saltatory conduction. Characteristics of action potentials: 1. All-or-None: This means that once the threshold is reached, the action potential either occurs fully or not at all. When a neuron gets a strong enough signal, it fires an action potential fully. It’s like a light switch: it’s either completely on or off. If the signal isn’t strong enough, nothing happens. 2. Active: Action potentials require energy because they involve the movement of ions (like sodium and potassium) across the neuron's membrane. This process is active, relying on ion channels and pumps to create changes in voltage.Action potentials need energy to work. This is because they involve moving ions (like sodium and potassium) in and out of the neuron. This movement changes the electrical charge inside the neuron and requires energy. 3. Regenerative: Once an action potential starts, it triggers more action potentials in neighboring segments of the axon. This self-propagation ensures that the signal moves quickly along the axon, without losing strength. 4. Unidirectional: Action potentials travel in one direction, away from the cell body toward the axon terminals. This is because after an action potential occurs, the segment of the axon that just fired enters a refractory period where it cannot fire again immediately. This prevents the signal from traveling backward (called refractory period) Discussion post 2 1. What is a spinal reflex? Give a brief description of the components of a reflex pathway. A spinal reflex is a quick, automatic skeletal muscle reaction that happens without the brain directly triggering it. A somatic receptor, sensory neuron, an interneuron, an motor neuron and an effector muscle are some of the parts of the reflex arc. Quick reactions to sensations are made by the reflex arc, which guarantees that action is initiated before the brain has had a chance to fully digest the information. 2. What is the difference between an autonomic and a somatic reflex? Give a brief description and an example of each (no need to draw a pathway). Autonomic reflex: This regulates spontaneous functions like heart rate. The body automatically raises the heart rate during exercise because it senses an increase in physical activity and wants to provide the muscles with more oxygen-rich blood. Somatic reflex: This kind of control happens actively and uses skeletal muscles. For instance, immediate withdrawal of your hand when you come into contact with something hot. Also, somatic reflex is Patellar reflex: For example, the quadriceps muscle relaxes and the lower leg pushes out when the patellar tendon is tapped, setting off a reflex arc. 3. The primitive reflexes observed in newborns often have an evolutionary adaptive value. What might be the adaptive value of the rooting reflex? What about the palmar reflex? Rooting reflex: Helps babies in identifying and attaching onto the breast for feeding, this response is adaptive and ensures that they get the nutrition they need to survive. The baby turns their head and opens their mouth to try to suck when their cheek or side of their mouth is rubbed. Palmar reflex: A newborn will grab a finger when it is placed in their palm. When a baby is most at risk in their first days of life, this response might help them attach to a caregiver for warmth and comfort, which is essential for their survival. Additionally, by encouraging connection between the baby and their caregivers, this holding activity promotes nurturing relationships. 4. Why might a physician test a reflex like the patellar stretch reflex and what information does it provide? To evaluate the health of the neurological system, particularly the pathways linked to the knee-jerk response, a doctor will test the patellar stretch reflex. To make sure everything works as it should, this reflex test examines the spinal cord, motor neurons, and the connections between them. It could put insight into suspected neurological disorders or problems with spinal cord function. 1. What are the main similarities and differences between these two pathways? 1. The main difference between the two pathways is the location within the central nervous system that the information processing occurs. In the pupillary reflex arc, it occurs in the midbrain. Meanwhile, in the knee-jerk reflex arc, it occurs in the spinal cord. A similarity between the two pathways is both efferent neurons deliver the information to skeletal muscles. In the knee-jerk reflex arc, the muscle is the extrafusal muscle fibers of the quadriceps. In the pupillary reflex arc, the muscle is the ciliary muscle of the iris.