EXPH 386 Miriam Leary Notes PDF
Document Details
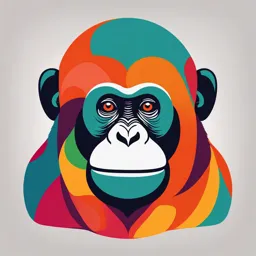
Uploaded by AmazingEiffelTower
West Virginia University
Miriam Leary
Tags
Summary
These notes cover the key functions of the cardiovascular system, including circulation, blood pressure, and venous return. They describe the heart's structure and function at various levels of detail.
Full Transcript
EXPH 386 Miriam Leary Notes Key functions of the CV system ○ Delivers oxygen and nutrients to active tissues ○ Returns deoxygenated blood to the lungs ○ Transports heat (byproduct of cellular metabolism) and hormones Circulatory System ○ Circulatory/Cardiovascular ○ Th...
EXPH 386 Miriam Leary Notes Key functions of the CV system ○ Delivers oxygen and nutrients to active tissues ○ Returns deoxygenated blood to the lungs ○ Transports heat (byproduct of cellular metabolism) and hormones Circulatory System ○ Circulatory/Cardiovascular ○ The heart is dual pump ○ 2 blood flow circuits Systemic circulation- heart and system Everywhere else in the body besides the lungs Pulmonary circulation- heart and lungs A few differences between the circuit The blood flow to the pulmonary circuit doesn’t have to go very far so the blood doesn’t have to travel as far as the blood in systemic circulation System circulation has higher pressure Arteries carry blood away from the heart but it may not always be oxygenated ○ Systemic circulation: carries oxygenated blood away from the heart to the body and returns deoxygenated blood back to the heart The heart has 2 cell types ○ Electrical: electrical pathway ○ Contracticle: mechanical cell Heart Structure and Function ○ Skeletal muscle main features Fibers: striated tubular and multi nucleated Voluntary Usually attached to skeleton ○ Cardiac muscle main features Fibers: striated branched and uninucleated Involuntary Only covering the walls of the heart Anatomy of the Heart ○ 4 chambers Right atrium Right ventricle Left atrium Left ventricle ○ 4 valves Atrioventricular valves Tricuspid (right) Bicuspid/mitral (left) Semilunar valves Pulmonary Aortic Terms and Definitions for Heart Contraction ○ Diastole: rest End Diastolic Volume: volume in the heart during rest ○ Systole: contraction End Systolic Volume: volume in the heart during contraction ○ EDV-ESV= Stroke Volume (how much blood is ejected/heart beat) ○ Isovolumetric: same volume Isovolumetric contraction: brief period of time (0.02-0.06 seconds) when all heart valves remain shut, ventricular pressure rises but volume remains unchanged Isovolumetric relaxation: brief period of time (0.02-0.06 seconds) when all heart valves remain shut, ventricular pressure drops but volume remains unchanged Venous Return ○ One way valves: helps prevent backflow and keeps blood moving toward the heart ○ Smooth muscle layer ○ Skeletal muscle pump Alternate compression and relaxation to return back to the heart Distribution of Blood at Rest ○ Venous pooling May induce fainting from insufficient cerebral blood supply So much blood stored at the bottom of the body so blood flow cannot get to the brain quick enough Varicose Veins ○ Veins fail to maintain their one way blood flow ○ Blood gathers- veins are distended and painful ○ Phlebitis- venous wall is inflamed and progressively deteriorates Vessel removal is necessary ○ Exercise cannot prevent varicose veins but minimizes complications Blood Pressure Follows a Gradient ○ The mean BP decreases from the aorta to the venae cavae ○ Decreases as the circulating blood moves away from the heart through arteries ○ BP has its greatest decrease in the small arteries and arterioles ○ BP continues to decrease as the blood moves through the capillaries and back to the heart through veins Blood Pressure ○ Systolic blood pressure ○ Diastolic blood pressure Mean Arterial Pressure ○ MAP is the perfusion pressure of the organs ○ MAP < 60 mmHg for an appreciable amount of time the end organ will get insufficient blood flow and become ischemic ○ MAP = DBP + [ ⅓(SBP-DBP)] ○ Example: BP= 120/80 80 + [⅓(120-80)] = 93 Hypertension ○ Hardened arteries and neural hyperactivity causes increased resistance ○ 95% of cases are idiopathic (etiology unknown) Factors that influence arterial BP ○ MAP = CO * TPR where CO= HR x SV ○ Increased BP with increased: Blood volume Heart rate Stroke volume Blood viscosity Total peripheral resistance ○ TPR = diameter, length, viscosity Vessel length does not change by the time you are an adult The way TPR is changed is through the diameter of the vessel If we have a large vessel and we vasoconstrict it that severely decreases the blood flow How does altering CO change MAP? ○ With constant flow in and out of the vessel we maintain a constant MAP ○ If you increase your heart rate with exercise which increases your SV which increases your flow so your pressure will increase Flow cannot go farther faster in the vessel so the pressure therefore increases By changing the resistance of the end of the vessel that is how we maintain MAP Our body can adjust resistance on the ends of vessels to maintain MAP MAP needs to stay in our normal healthy range If cardiac output goes down, TPR must increase to maintain MAP Total Peripheral Resistance ○ The body uses blood vessel diameter to help regulate BP ○ Nerves controlling the muscle fibers can cause the vessel to contract Vasoconstriction or Vasodilation Cardiac Output = HR x SV ○ CO is the amount of blood that is ejected by one ventricle in one minute ○ At rest, CO= 5 L/min ○ To increase CO Increase HR, SV, or both ○ When arterioles are relaxed, blood flows freely. This is similar to the free flow of water in a garden hose when the spray nozzle is removed. The spray nozzle adds resistance and a pressure build up Cardiac Output and TPR ○ MAP = CO x TPR or TPR = MAP/CO ○ MAP = DBP + [⅓ (SBP-DBP)] ○ TPR = DBP + [⅓ (SBP-DBP)]/ CO ○ At rest SBP = 126 DBP = 80 CO = 5 L/min TPR = 19 mmHg/L Steady Rate Exercise ○ During the first few minutes of exercise increased blood flow (CO) during steady rate exercise rapidly increases SBP ○ Vasodilation (active muscles) decreases TPR to increase blood flow to the peripheral vasculature ○ As exercise continues, SBP gradually decreases because the arterioles (in active muscles) continue to dilate which decreases TPR ○ Muscle contraction and relaxation “milks” blood back to the heart (venous return) Graded Exercise ○ SBP increases rapidly at the start of exercise, the SBP increases linearly with exercise intensity ○ DBP remains unchanged or decreases slightly ○ Max SBP may reach 200+ mmHg despite reduced TPR This level of blood pressure most likely reflects the heart’s large CO during maximal exercise by individuals with high aerobic capacity Blood Pressure Response to Exercise ○ Resistance exercise Concentric (shortening) contractions compress peripheral vasculature causing an increase in resistance Magnitude of increase is related to the intensity of effort and the amount of muscle mass used Submax Upper Body Exercise ○ UBE exercise requires greater O2 consumption than leg exercise at any submaximal power output Lower mechanical efficiency Recruitment of stabilizing muscles Greater physiological strain than LBE Higher HR, Ve, BP, and RPE Upper Body Exercise ○ Maximal exercise VO2 values are typically 20-30% below those of lower body exercise Lower max HR and pulmonary ventilation Smaller musculature activated Cardiac Output and TPR Example ○ SBP=210 ○ DBP= 90 ○ CO= 20 L/min ○ TPR = DBP + [⅓ (SBP-DBP)]/ CO ○ TPR= 6.5 Hypotensive Recovery Response ○ After a bout of sustained light to moderate exercise, SBP temporarily decreases below pre-exercising levels for up to 12 hours in normal and hypertensive subjects ○ Mechanism Blood pools in skeletal muscle vascular beds during recovery What is the clinical significance of an exaggerated BP response? ○ Future development of hypertension ○ Increased risk for cardiovascular mortality and cardiovascular events ○ Presence of cardiac hypertrophy Increased muscle Heart's Blood Supply ○ No nutrients are taken from the blood in the heart chambers ○ Coronary circulation supplies the heart muscle 5% (250ml) of total output ○ Right and left coronary arteries start at the aorta behind the aortic valve ○ Arteries divide into a dense capillary networks that feeds the myocardium ○ Coronary sinus and anterior cardiac veins collect blood and empty directly into the right atrium Myocardial Oxygen Utilization ○ At rest the myocardium extracts 70-80% of the oxygen from the blood flowing in the coronary vessels Most other tissues only use 25% ○ In vigorous exercise coronary blood flow increases 4-6 times above resting level Impaired coronary blood supply ○ Coronary muscle is purely aerobic ○ If blood flow is interrupted chest pain results (angina pectoris) ○ Exercise increases energy requirements Stress test us used as a diagnostic tool for myocardial blood flow (ischemia) ○ A blood clot within these vessels results in myocardial infarction Damage ranges from mild to severe ○ Ischemia: restriction in blood supply to tissues ○ Hypoxia: a region of the body is deprived of adequate O2 supply ○ Infarction: tissue death caused by a local lack of oxygen Rate Pressure Product ○ Provides a non-invasive estimation of myocardial workload (myocardial oxygen uptake) ○ RPP= SBP x HR ○ RPP= linear relationship with exercise intensity ○ Chronic exposure to higher myocardial workload can lead to cardiac hypertrophy Cardiovascular Regulation and Integration ○ Cardiac muscle has an ability to maintain its own rhythm (i.e heart rate) ○ Without extrinsic stimuli the intrinsic heart rate 100 BPM ○ Extrinsic control of the heart (i.e neurohumoral factors) can adjust heart rate from 40 bpm at rest to 220 bpm at peak exercise Autorhythmic/Automaticity ○ The heart can beat on its own without the need for exogenous commands ○ Don’t rely on a nerve to contract the heart Intrinsic Regulation of Heart Rate ○ Membrane potential from negative to positive and will start depolarization ○ Starts at the SA node but will propagate outwards ○ Sinoatrial node to the Atrioventricular node to the purkinje fibers and then to the bundle of HIS ○ As it gets to the bottom of the ventricle the signal will travel up the walls of the heart ○ AV node delays the electrical signal ○ 0.10 second delay allows atria to contract before ventricular filling ○ 0.06 seconds time till ventricles contract upon stimulation Electrocardiogram (ECG/EKG) ○ Records the electrical activity of the heart ○ P wave Atrial depolarization ○ QRS complex Ventricular depolarization and atrial repolarization simultaneously ○ T wave Ventricular repolarization ○ ECG abnormalities may indicate coronary heart disease ST segment depression can indicate MI Relationship between Pressure Changes and ECG ○ We have atrial depolarization which signals atrial contraction which has a small increase in blood volume which acts on the ventricular walls increasing pressure ever so slightly ○ Polarization passes AV node and into ventricles which causes contraction and generates maximal pressure which signals ventricular repolarization Areas of the Heart That Can Initiate a Rhythm and Intrinsic Rate ○ The slower the lower ○ Sinus node: 60-100 BPM ○ Atrial Tissue: 60-100 BPM ○ AV node: 40-60 BPM ○ Ventricular Tissue: 20-40 BPM Extrinsic Heart Rate Regulation ○ Neural influences superimpose on the inherent rhythm of the myocardium To accelerate HR in anticipation of exercise and adjust HR as exercise intensity increases or decreases ○ Range 20-200 BPM (dependent upon physical fitness) Sympathetic and Parasympathetic Control ○ Sympathetic stimulation Catecholamine (NE/Epinephrine) Results in tachycardia Increases SA node depolarization, increases HR Increases contractility ○ Parasympathetic influence Acetylcholine- vagal influence Results in bradycardia Slow SA node depolarization, slows HR Exercise and Blood Flow Parasympathetic Neural Control ○ Periphery Excitation of iris, gallbladder, coronary arteries Inhibits gut sphincters, intestines, and skin vasculature During the onset of exercise, HR increases by inhibition of PNS Sympathetic Control on Blood Flow ○ Mechanism is NE via adrenergic fibers that lie within smooth muscle of small arteries, arterioles, and precapillary sphincters ○ Inactive Tissue (vasoconstriction) Renal, splanchnic, and inactive skeletal muscle Produces systemic vasoconstriction of inactive muscle ○ Active Tissue (vasodilation) Active muscle Decreases SNS activity Neural Response During Exercise ○ Anticipatory response Decrease parasympathetic Increase sympathetic ○ Exercise Parasympathetic inhibition (early) Sympathetic stimulation (more intense) ○ What happens? Increased HR and contractility Increase in arterial blood pressure Vasodilation of active skeletal muscles Vasoconstriction of skin gut spleen etc. Vasoconstriction increases venous return Functional Sympatholysis ○ Sympathetic neural activity on blood vessels = vasoconstriction ○ So how do we get increased blood flow to active tissues? Local changes in muscle metabolites and other substances reduce vascular responsiveness to alpha adrenergic receptor activation Peripheral Input Control of CV response ○ Initial signal to “drive” cardiovascular system comes from higher brain centers ○ Fine-tuned by feedback from Chemoreceptor Sensitive to blood, O2, CO2, and pH levels Baroreceptors Sensitive to changes in arterial blood pressure Muscle mechanoreceptors (heart, skin, joints) Sensitive to force and speed of muscular movement Metaboreflex muscle chemoreceptors Sensitive to muscle metabolites (K+ and lactic acid) Exercise pressor reflex All of these detect changes in chemistry or pressure BP response to standing ○ As you stand up gravity induced drop in BP from gravity pulling the blood down detected by baroreceptors in the carotid and aorta ○ Baroreceptors sense changes in the walls ○ This is going straight to the brain and the brain will response by activating the sympathetic nervous system to increase HR and contractility to increase CO so you will get more blood flow which increases blood volume which will then increase your blood vessels ○ Blood vessels are constricted with alpha 1 receptors Baroreceptors ○ Aortic arch ○ Common carotid artery branching into internal and external ○ Let's say BP gets too high Stimulate the baroreceptors which will sense to high BP and send the signal to the brain which will go to the cardioacceleratory center to inhibit it and then stimulate the cardioinhibitory system To lower BP we need to lower CO Done by blocking acceleration and stimulating inhibition HR is reduced CO will then be decreases Vasomotor center inhibited so vessels don't constrict Arterial vasodilation occurs in hopes of reducing BP Homeostasis restored Chemoreceptors ○ Oxygen, CO2 ○ Normal O2 and CO2 levels ○ Let’s say homeostasis is disturbed Decrease in O2 and pH with an increase in CO2 Chemoreceptors stimulated and signal our reflex response Cardioinhibitory center inhibited Cardioacceleratory center stimulate These lead to an increased CO Respiratory center is stimulated so we increase our respiratory rate to increase our breathing Finally increased O2 and pH with a decrease in CO2 levels in the blood Homeostasis finally restored Blood flow regulation ○ Flow = pressure x resistance ○ Determining factors for TPR to CO Physical characteristics of the blood (viscosity) Size of individual vessels Length of conducting tube Diameter of blood vessel Poiseuille’s Law ○ Quantify blood flow resistance ○ Flow = pressure gradient x vessel radius^4/ vessel length x viscosity ○ Vessel diameter = most important ○ Vessel length and blood viscosity = less impact as they don’t usually change and stay constant ○ Small alterations at the level of the vessel can cause great changes in blood flow Exercise and Blood Flow ○ Nerves and local metabolites act to dilate arterioles in active muscle beds ○ Visceral vasoconstriction and muscle pump bring blood to central circulation ○ Example: Kidneys Rest= 1100 ml per minute (20% of CO) Exercise= 250 ml per minute (1% of CO) Active Muscle ○ Dormant capillaries (1 out of 40 open) at rest ○ Three functions of opening dormant caps Increase to muscle blood flow Large blood volume with only minimal increase in blood flow velocity Increase the effective surface area for gas and nutrient exchange between blood and muscle fibers Local factors- autoregulatory mechanisms Decrease pH, increase PCO2, increase ADP, increase Ca ++, increase temp