PHS203: Exitable Tissues & Autonomic Systems PDF
Document Details
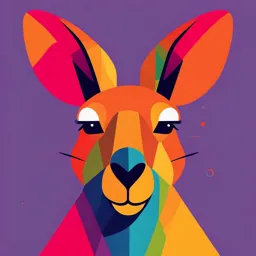
Uploaded by StimulatingLimerick481
Baze University Abuja
Tags
Summary
This document provides an introduction to excitable tissues and electrical signals in cells. Topics covered include cell membrane properties, ion movement, and action potentials. It discusses the four primary tissue types in complex animals and their response to stimuli. This document appears to be lecture notes rather than an exam paper.
Full Transcript
Monday, 25th September, 2023 PHS203: EXCITABLE TISSUES AND AUTONOMIC SYSTEMS WEEK 1-5: Introduction to excitable cells and electrical signals in cells Basic Properties and Structures of the Cell Membrane Movement of Ions across cell Membranes Types of Membrane...
Monday, 25th September, 2023 PHS203: EXCITABLE TISSUES AND AUTONOMIC SYSTEMS WEEK 1-5: Introduction to excitable cells and electrical signals in cells Basic Properties and Structures of the Cell Membrane Movement of Ions across cell Membranes Types of Membrane Transport Proteins Generation of electrical Potentials in cells and Electrochemical Equilibrium Action Potentials and their Propagation Neuromuscular Transmission Intended Learning Objective: Understand the types of tissues that are called excitable tissues (nerve and muscle) Discuss: Depolarization Repolarization Action potential Resting membrane potential (RMP) INTRODUCTION: 1) Complex animals have four primary types of tissues; Epithelial Connective Muscle Nervous 2) These tissues all respond to stimulus: chemical, electrical and mechanical. 3) However, nerve and muscle generate transmittable signals (action potential) after stimulation, this ability classified them as EXCITABLE TISSUES. 4) All cells have 2 major compartments; intracellular compartment, and extracellular compartment. This compartmentalization is formed by cell plasma membrane. 5) Cell membrane is a semi-permeable membrane (selective fiber) that allows the free passage of certain molecules and tightly regulate the passage of others. 6) The movement across the membrane depends on multiple factors such as; electrical charge molar mass Polarity of the molecules. 7) Movement of uncharged substance like oxygen, CO2, and glucose depends on conc. gradients. 8) Charged substances such as K+, Na+, Cl- can’t diffuse across the membrane due to its internal hydrophobic structures. Commented [h1]: Some channels allow positively 9) This requires a specialized, water filled ion channels; ion channels, have the following characteristics; charged molecules but some won’t allow negatively charged molecules. Finely timed opening and closing Selective based on size and charge of molecules. High selectivity Depends on solubility of molecules (water soluble, lipid soluble) FACTORS THAT MEDIATE THE MOVEMENTS OF IONS: 1) CONC. GRADIENT: diff in conc. of ions on 2 sides of membrane 2) ACTIVE TRANSPORT: 3) ELECTRICAL GRADIENT: positive ions will be attracted to negative electrical potential and Commented [h2]: Will explain action potential repelled from (+) electrical potential. Inside= 130 mmol/L Outside= 4 mmol/L 4) SODIUM-POTASSIUM PUMP K+ inherently an intracellular ion. Conc. gradient: different levels of substance in and out of cell. Higher conc lower conc. Electrical gradient: positive repel positive, positive attract negative etc Active: To understand how conc. gradient and electrical gradient influence movement of ions across the cellular membrane, let’s analyze the movements of k+ ions: Conc. gradient: the intracellular conc. of K+ greatly exceed the extracellular conc. Thus K+ will tend to exit the cell according to the conc. gradient. Electrical gradient: As (+)charged K+ ions are released, the charge of the intracellular space becomes Commented [h3]: As intracellular is negative (-65mV), relatively negative. Hence some K+ ions are attracted back towards the intracellular space, despite the electrical pull pulling K+ into cell. conc. gradient leading them in the opposite direction. Commented [h4]: Since ECF = rich in cation Results in inequality in ionic And ICF = richer in anion distribution across the membrane Unequal distribution of this ion= membrane potential MEMBRANE POTENTIAL: Before stimulation/ rest: resting membrane potential (RMP) Commented [h5]: Negative inside At equilibrium potential: rate at which ions leave by conc. gradient = rate at which ions enter via Commented [h6]: In a cell where only one type of ion can electrochemical gradient. cross the membrane, the resting membrane potential will equal the equilibrium potential for that particular ion. Nernst Equation: used to calculate the value of the equilibrium potential of a particular cell for a RMP: naturally occuring particular ion. Commented [h7]: Artificially created Assume only 1 ion in membrane At what point will the rate it is entering be the same as rate of its going out. Vm= equilibrium potential Z= valence of ion [C]0= conc. Of ion X (outside of cell)/ mol [c]i= conc. Of ion X (inside cell)/mol Assuming only K+ ions could cross the membrane and knowing common values for the ICF and ECF conc. Of K+, one can calculate the approximate resting potential of a cell. The RMP is maintained by sodium-potassium pump (Na+ K+ ATPase) Commented [h8]: Na+ is extracellular ion. Wants to move This pump actively transports K+ and Na+ ions against their electrochemical gradients (i.e. K+ moves into cell. Sodium-potassium pump oppose natural movement/natural gradient of ions so it needs energy (ATP - intracellularly and sodium moves extracellularly). ADP + energy) therefore it uses active transport. If there was to be any change in the permeability of the cell membrane to ions (via channels opening or 2 molecules for 3 molecules. closing) then the membrane potential would be altered – this will result to the generation of ACTION “For every ATP molecule that the pump uses, three sodium ions are exported and two potassium ions are POTENTIAL. imported” Measurement of RMP: use intracellular recording method: 2 electrodes: recording and reference electrode connected to voltmeter ACTION POTENTIAL: The application of threshold stimulus produces action potential; action potential is the electric activities or pulses generated/ propagated in nerves. When recorded by EC method, it produces ascending (depolarization) and descending curve (repolarization limb). Stimulus alters permeability of ions and that generates an action potential. Electrical activity that take place in muscle and neuron (a momentary reversal in electrical potential across a plasma membrane (of a neuron or muscle fiber) that occurs when a cell has been activated by a stimulus) Reverse from negative to positive. When cell is stimulated. Brief positive change in MP when cell is stimulated into action Occurs in excitable membranes All or nothing (either there is action potential or no) Critical level must be reached (threshold) before impulse is sent Positive feedback mechanism Measurement of AP Extracellular shows transition; life recording Intracellular shows at specific points; single reading Depolarization (ASCENDING): Change within cell During which cell undergoes shift in electric charge distribution Resulting in less negative charge inside cell. Negative intracellular charge positive Depolarization is the loss of normal resting polarized state of membrane shown as the rise from -70mV to positive scale of +35mV. STAGE 1: -70Mv to -55mV (initial stage) STAGE 2: -55mV to 0mV (rapid depolarization) STAGE 3: 0mV to 35mV (over shoot) Depolarization is as a result of the opening of the voltage-gated sodium channel leading to influx of sodium ions inside the cell. DEPOL PHASE: Stimulus causes adjacent Na+ ion channels to open along axon Na+ ions rush into cell (high to low conc.) Movement of Na+ ions reverses the change of the membrane (depolarization): Inside cell membrane – positive charge Outside cell membrane – negative charge If threshold is reached, adjacent Na+ ion channels to open along axon. DEPOL: Surface (used to be positive) negative Spread of surface negative charge Repolarization (DESCENDING): Repolarization is the restoration of the polarize state, as observed in the descending limbs from +35mV to -70mV making the inside negative and outside positive. It occurs in 3 stages: STAGE 1: rapid repolarization from +35 to -70mV STAGE 2: hyper polarization from -70mV to -80mV. STAGE 3: After hyper polarization from -80mV to -70mV. Ionic: It’s as a result of closure of the Na+ channels and opening of the K+ leading to efflux of K+ from the cells. Restoration of negative charge inside (resting) cell Removed stimulating electrode Restore cell to rest Spreading of surface positive charge K+ ion channels open K+ ions rush out of the cell (high to low conc.) Restores polarization of cell membrane Inside cell membrane – negative charge Outside cell membrane – positive charge Ion distribution is different than at resting potential – can’t stand another impulse yet Inside cell – low K+, high Na+ Outside cell – high K+, low Na+ LEARN THIS DIAGRAM THIS IS AN EXTRACELLULAR REPRESENTATION HYPERPOLARIZATION: Commented [h9]: homeostasis AFTERPOTENTIAL: Restore back to -70 The cell membrane of all body cells in the resting condition… Determinants of membrane potential: AP consists of: Depol phase: this is the rising phase of AP and it takes 0.2-0.5 ms. Firing level (FL): absolute magnitude of MP where AP is fired or generated, usually occurs after an initial 15mV of depol. Spike potential: sharp rise and fall in AP. Overshoot: This is the portion of the AP between zero and peak potential. Peak potential: has a value of +20mV to +40mV (in all cells) After potential: portion of AP after 70% of repol: After hyperpol: part of after potential that is more negative than RMP. After depol: part of after potential that is more positive than RMP. IONIC BASIS OF AP: When stimulated, polarity is abolished and reversed briefly. Stimulation leads to increased permeability changes for Na+, influx of Na+, depol, generation of AP once FL is reached MP moves towards equilibrium of Na+ but rather stops at peak potential (PP). Commented [h10]: The sodium gates are shut and due to Later permeable to K+ efflux, repol the peak potential, potassium channels open and potassium efflux out of cell carrying positive charges, therefore Opening of K+ channels more slower and prolonged than Na+ restoring the negativity of the intracellular space PROPAGATION OF ACTION POTENTIAL: Two directions: 1. Orthodromic (anterograde) Commented [h11]: An orthodromic impulse runs 2. Antidromic (retrograde) along an axon in its anterograde direction, away from the soma. Two types: Orthodromic denotes the propagation of an impulse alo ng a conduction system (for example, nerve fiber) in th 1) Electrotonic (local current flow) in unmyelinated e direction it normally travels. Commented [h12]: An antidromic impulse in an axon refers to conduction opposite of the normal (orthodromic) direction. That is, it refers to conduction along the axon away from the axon terminal (s) and towards the soma. rare doesn’t happen in human beings. Commented [h13]: Na+ entry depolarizes the membrane, which opens additional Na+ channels. Positive charge flows into adjacent sections of the axon by local current flow. MECHANISM OF NERVE IMPULSE CONDUCTION: In unmyelinated neurons, impulses are conducted in the form of waves of action potential. It travels along the nerve. In unmyelinated fibers, every part of the axonal membrane needs to undergo depolarization, making the propagation significantly slower. 2) Saltatory (node to node) in myelinated (faster) Commented [h14]: In myelinated nerve fibers, impulse are propagated by a special mechanism called SALTATORY CONDUCTION. Hence, impulses jumps from one node of ranvier to the other making it the fastest method of impulse conduction which is x50 of unmyelinated. (COMMON MCQ QUESTION) (IN SHORT NOTE, DRAW AND EXPLAIN) myelin enables saltatory conduction of the action potential, since only the Ranvier nodes depolarize, and myelin nodes are jumped over. REFRACTORY PERIOD: Commented [h15]: Those times when the nerve fibers cannot respond to stimulus Cannot produce a response no matter how high the Is the time after an action potential is generated, during which the excitable cell cannot produce stimulus is another action potential. Prevents backward current flow Absolute refractory period (ARP): Second stimulus does not produce a response no matter how high it is. Frog nerves and muscles (2-3ms); mammalian nerves and muscles (0.4-1ms) No stimulus of any strength can trigger an AP (this is as a result of continuous opening of sodium gates) Relative Refractive Period: Period after ARP during which only a suprathreshold stimulus can produce an AP. Commented [h16]: Subthreshold stimuli cannot cause Only stronger than normal stimulus will result to a new AP. (This is as a result of continuous an action potential. Threshold stimuli are of enough energy or potential to opening of K+ gates this opposes and incoming Na+) produce an action potential (nerve impulse). Suprathreshold stimuli also produce an action potential, but SUPERNORMAL: During the supernormal period, excitation is possible in response to an their strength is higher than the threshold stimuli. otherwise subthreshold stimulus; that same stimulus fails to elicit a response earlier or later than the supernormal period. Two factors are responsible for supernormality: the availability of fast Na+ channels The proximity of the Em to threshold potential. During the supernormal phase of excitability, the cell has recovered enough to respond to a stimulus (i.e., an adequate number of Na+ channels is available for activation). At the same time, because the Em is still reduced, it requires only a little additional depolarization to bring the fiber to threshold; thus, a smaller stimulus than is normally required elicits an action potential. SUBNORMAL: a little triggers can produce an action potentials (AP) Commented [h17]: a period of subnormal excitability is followed by a supernormal period of slightly increased excitability. Following the supernormal period, in a period called the late subnormal period, the excitability of the axon returns to baseline and the threshold current again increases COMPOUND ACTION POTENTIAL: Commented [h18]: Caused by multiple stimuli. A compound action potential (CAP) is a signal recorded from a nerve trunk made up of numerous axons1. It is the result of summation of many action potentials from the individual axons in the nerve trunk. FACTORS THAT AFFECT THE EXCITATORY OF NERVES: Temperature: cooling decreases and warm increases Pressure: mechanical pressure decreases Blood Supply: Ischemia decreases Oxygen Supply: Better oxygenation increases Commented [h19]: Genetic mutations that result in loss of Na+ channel function, Na+ channel blockade with class I antiarrhythmic drugs, and acute myocardial ischemia can cause reduced membrane excitability. Commented [h20]: Ischemia is a condition where blood flow and oxygen are reduced to a part of the body, causing damage or death to the affected tissue NERVE CELLS: Neuron: primary structural and functional unit of nerve tissue (brain, spinal cord, nerves, sensory cells) DENDRITE: Fibrous root Branch out from cell body Receive and process signals from the axons of other neurons CELL BODY: Carries genetic info. Maintains structure Provides energy AXON: Long, tail-like Joins cell body and axon hillock Some myelinated (insulated with fatty substance called myelin) some unmyelinated SYNAPTIC KNOB: Axon terminal TYPES OF NERVES: TYPE A: Largest (5-20 micr, 130 m/s) Myelinated Somatic Sensory Motor to skeletal muscles TYPE B: Medium (2-3 microns, 15m/s) TYPE C: Small (0.5-1.5 microns, 2.4 m/s) Unmyelinated.