Excitable tissues and ANS_013408-1.pptx
Document Details
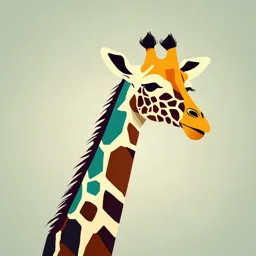
Uploaded by HeroicVision5762
Kaduna State University
Full Transcript
NERVE AND MUSCLE PHYSIOLOGY & AUTONOMIC NERVOUS SYSTEM DR DANBOYI TIMOTHY DEPARTMENT OF HUMAN PHYSIOLOGY FACULTY OF BASIC MEDICAL SCIENCES COLLEGE OF MEDICINE KADUNA STATE UNIVERSITY COURSE OUTLINE Orga...
NERVE AND MUSCLE PHYSIOLOGY & AUTONOMIC NERVOUS SYSTEM DR DANBOYI TIMOTHY DEPARTMENT OF HUMAN PHYSIOLOGY FACULTY OF BASIC MEDICAL SCIENCES COLLEGE OF MEDICINE KADUNA STATE UNIVERSITY COURSE OUTLINE Organization of the Nervous System and Physiologic Anatomy of a Neuron Nerve Impulse: Action Potential and Classification of Nerve fibers Synapses: Transmission Pathways Physiology of Skeletal muscles Physiology of Smooth muscleS Physiology of Cardiac muscles Neuromuscular Transmission Physiologic Anatomy of Autonomic Nervous System (ANS) Sympathetic and Parasympathetic Systems of the ANS Effects of ANS impulses on effector organs Drugs acting on the ANS INTRODUCTION Primary function of the nervous system is to maintain homeostasis through the control of body systems. Some aspects of the neural system involve motor commands to skeletal, cardiac and smooth muscles of the target organs. The motor commands issued by the nervous system are commonly generated in response to sensory inputs. Sensors of the nervous system detect changes in the body’s internal and external environment and transmit the sensory information to the brain for storage and use in generating a response. INTRODUCTION Contd Complex interactions & activities of the neurons as manifested in our day-to-day activities Intact Nervous System (NS) = intact life Interplay btw the different parts of the brain, the nerves, receptors and outside environment About 1m bits of info are received each minutes by the NS & processed or integrated to determine the appropriate response to be made by the body Most activities in the NS are initiated by stimuli exciting the sensory receptors The stimuli can either cause a prompt response from the brain or stored for future exposure GENERAL FUNCTIONS OF THE NERVOUS SYSTEM The CNS is equivalent to the CPU of a computer Exerts its functions at 3 levels: 1. The spinal cord level: walking movt and various reflexes 2. The subcortical levels: subconscious activities e.g BP control, control of respiration, equilibrium and emotions, sleep and behaviour 3. The cortical level: large memory store house which fxn always in association with the subcortical areas – Regulates the fxns of the lower centres – Essential for most of the thought processes ORGANIZATION OF THE NERVOUS SYSTEM - Origin In the developing embryo, the Nervous system (NS) develops from ectoderm, that forms the neural plate. The neural plate differentiates into neural tube and a neural crest. The neural tube then differentiates into the Central Nervous System (CNS), which consists of the Brain and the Spinal cord. The neural crest gives rise to most of the Peripheral Nervous System (PNS), which consists of 12 pairs of cranial nerves and 31 pairs of spinal nerves. ORGANIZATION OF THE NERVOUS SYSTEM – Cont’d The Central Nervous System The brain develops at the cranial end of the embryonic neural tube. By the end of the first month of development, three (3) Primary vesicles are formed: Forebrain (Prosencephalon), the Midbrain (Mesencephalon), and the Hindbrain (Rhombencephalon). A week later, the forebrain gives rise to the Telencephalon and the Diencephalon, and the hindbrain gives rise to the Metencephalon and the Myelencephalon resulting in a total of five (5) secondary vesicles. ORGANIZATION OF THE NERVOUS SYSTEM – Cont’d In adults: Telencephalon develops into cerebral hemispheres. Diencephalon gives rise to the Thalamus and the Hypothalamus and other structures. Mesencephalon becomes the Mylencephalon becomes Midbrain. Medulla oblongata. The medulla oblongata, pons and Metencephalon gives rise to the the midbrain form the adult Pons and the Cerebellum. Brain stem. ORGANISATION OF THE CNS PHYSIOLOGIC ANATOMY OF A NEURON Neurons are the functional units of the nervous system that have four distinct regions: Soma: this is the cell body of a neuron and its metabolic center. It contains the components necessary to synthesize and package proteins used in other parts of the cell to maintain a variety of cellular functions. These include cytoplasm, nucleus and cell organelles. It also contains granules called Nissl granules/bodies that are regarded as the ER of the neuron. Cell bodies are usually located mainly in the CNS but some are found in the autonomic and dorsal root ganglia. PHYSIOLOGIC ANATOMY OF A NEURON – Cont’d Dendrites: are usually short, branchlike structures that extend out from the cell body. The surfaces of the dendrites and soma are where most neurons receive information from other nerve cells. Axon: is a long fiberlike structure that extends from the soma arising from the axon hillock (which together with its adjacent small part forms the initial segment) to make contact with other nerve cells, or effectors, such as muscle fibers. PHYSIOLOGIC ANATOMY OF A NEURON – Cont’d Initial Segment/Axon Hillock: is where action potentials are generated and transmitted down the axon as nerve impulses. Myelinated nerve fibers are electrically insulated from extracellular fluid by segments of myelin. Unmyelinated nerve fibers lack the myelin insulation. Near it distal end, the axon branches many times, forming axon terminals, which make contact with and transmit information to other nerve cells at junctions called synapses. A nerve fiber consists of several bundles of nerves that are bound together by glial tissue called the endoneurium. The bundles are bound by another glial tissue called the perineurium and the whole nerve fiber is enclosed or surrounded by a membrane called the epineurium. PHYSIOLOGIC ANATOMY OF A NEURON – Cont’d CLASSIFICATION OF NEURONS Each neuron has a cell body, dendritic processes and axon Neurons are classified according to their Functions (sensory/motor) Length of axons (Golgi type I/II) No. of poles (uni-,bi- or multipolar) Origin (cranial/spinal) Distribution (somatic/autonomic) Structure (myelinated/unmyelinated) Relation to ganglion (pre-/postganglionic) Secretion of neurotransmitter (cholinergic/adrenergic) Diameter & conduction velocity (Erlanger-Gasser) February 22, 2025 16 February 22, 2025 18 Erlanger-Gasser classification of nerve fibres Fibre type Function Diameter (um) Conduction velocity (m/s) Aα Proprioception, 12-20 70-120 somatic motor Aβ Touch, pressure 5-12 30-70 Aϒ Motor to muscle 3-6 15-30 spindle Aᵹ Pain, cold, touch 2-5 12-30 B Preganglionic ˂3 3-15 autonomic C dorsal Pain, tempt, some 0.4-1.2 0.5-2 root mechanoreception, reflex response C Postganglionic 0.3-1.3 0.7-2.3 sympathe sympathetic tic February 22, 2025 20 Alzheimer's Disease It is a progressive degenerating disease of the brain, characterized by dementia and it is always fatal. It results from a disruption of the cytoskeleton of neurons in the cerebral cortex, a region of the brain crucial for cognitive function. It results into memory loss that progresses to a point where the patient does not recognize family members Over time even the personality changes and in the final stages, other cognitive functions fail so that the patients cannot communicate with anybody. February 22, 2025 22 Alzheimer's Disease Con’t Diagnosis of Alzheimer’s is usually made through the patients declining performance on mental status examinations. The only definitive diagnosis comes after death, when brain tissue can be examined for neuronal degeneration, extracellular plaques made of β-amyloid protein and intracellular tangles of tau, which is a protein that is associated with microtubules. Although the presence of plaques and tangles is diagnostic, the underlying cause of Alzheimer’s is unclear. Currently there is no proven prevention or treatment, although drugs that are Ach agonist or acetylcholinesterase inhibitor slow the progression of the disease. February 22, 2025 23 Terms to Know Voltage (V): It is the measure of potential energy between two points generated by a charge separation Potential or Potential difference: It is the voltage difference between two points Membrane Potential: It is the voltage difference between the inside and outside of a cell Resting membrane potential: It is the steady transmembrane potential of a cell that is not producing an electrical signal. In neurons it is about -70mV. February 22, 2025 24 Terms to Know The terms depolarize, repolarize and hyperpolarize are used to describe the direction of changes in the membrane potential. Changes in membrane potential act as electrical signals. The RMP is “polarized”, which simply means that the outside and inside of a cell have a different net charge. The membrane is depolarized when its potential becomes less –ve (closer to zero) than the resting level February 22, 2025 25 Terms to Know Overshoot refers to a reversal of the membrane potential polarity, that is when the inside of a cell becomes positive relative to the outside. When a membrane potential that has been depolarized is returning toward the resting value, it is repolarizing. The membrane is hyperpolarized when the potential is more –ve than the resting level. February 22, 2025 26 Resting Membrane Potential All cells under resting conditions have a potential difference across their plasma membranes, with the inside of the cell negatively charged with respect to the outside. The RMP exists because of a tiny excess of – ve ions inside the cell and an excess of +ve ions outside. 80% caused by selective permeability of the cell membrane to K+. The K+ diffuses out of the cell according to concentration gradient 20% is caused by the Na+/K+ pump Dentistry 07 27 Electrical Signals in Neurons All living cells have a RMP due to the presence of ion pumps and leak channels in the cell membrane. This difference in charge can be measured as potential energy- measured in millivolts. In addition, however, some cells have another group of ion channels that can be gated (opened or closed) under certain conditions. Such channels give a cell the ability to produce electrical signals that can transmit information between different regions of the membrane. This property is known as Excitability and such membranes are called excitable membranes February 22, 2025 28 Electrical Signals in Neurons Cells of this type includes all neurons and muscle cells, as well as some endocrine, immune and reproductive cells. The electrical signals occur in two forms: graded potentials (GP) and action potentials (AP). GP are important in signaling over short distances, whereas AP are long-distance signals that are particularly important in neuronal and muscle cell membranes. February 22, 2025 29 Factors affecting the RMP Two Factors influence the membrane potential 1. Concentration of ions across the membrane. Normally, Na+, Cl⁻, and Ca²+ are more concentrated in the extracellular fluid, while Kᶧ is more concentrated in the intracellular fluid. 2. Membrane permeability to those ions. The resting cell membrane is much more permeable to K+ than to Na+ or Ca²+. This makes Kᶧ the major ion contributing to the resting membrane potential. February 22, 2025 30 Sodium-Potassium Pump/ Na+-K+ ATPase It is an active type of transport with enzyme activity that catalyzes the hydrolysis of ATP to ADP which transports 3 sodium ions out of cell in exchange to 2 potassium ions that move into the cell. The energy realized from the ATP-ADP conversion is needed for the movement of the ions against their concentration gradient which maintains the RMP. Mostly used in cardiac muscle and nervous tissue. Maintains Na+-K+ gradient concentration. Huge amount of ATP goes into this Pump and it’s found in all parts of the body. Sodium-Potassium Pump can be inhibited by endogenous Ouabain Action Potential Action potentials also called “spikes” or “impulses” are self-generating wave of electrical activity that propagates from its point of initiation at the cell body (axon hillock) to the terminus of the axon where synaptic contacts are made. An AP is a regenerating depolarization of membrane potential that propagates (conducted without decrement) along an excitable (capable of generating action potential) tissue. An action potential is a very rapid change (about 1 ms) called depolarization in membrane potential that occurs when a nerve cell membrane is stimulated. February 22, 2025 33 Action Potential Con’t Current passing through opening voltage- gated Na+ channels rapidly depolarizes the membrane, causing more Na+ channels to open. Inactivation of Na+ channels and delayed opening of voltage-gated K+ channels halt membrane depolarization. February 22, 2025 34 Action Potential Con’t Outward current through open voltage-gated K+ channels repolarizes the membrane back to a - ve potential. Persistent current through slowly closing voltage-gated K+ channels hyperpolarizes membrane toward Ek; Na channels return from inactivated state to closed state (without opening). Closure of voltage-gated K+ channels returns the membrane potential to its resting value. February 22, 2025 35 February 22, 2025 36 Voltage-Gated Channels – Change shape due to changes in membrane potential Closed at resting potential – Positive feedback Influx of Na+ local depolarization more Na+ channels open more depolarization – Na+ channels open first (depolarization) – K+ channels open more slowly (repolarization) – Na+ channels close – K+ channels close slowly relative refractory period caused by open K+ channels February 22, 2025 37 Threshold Stimulus and Threshold Potential Action potentials occur only when the membrane is stimulated (depolarized) enough so that sodium channels open completely. The minimum stimulus needed to achieve an action potential is called the threshold stimulus. The threshold stimulus causes the membrane potential to become less negative (because a stimulus, no matter how small, causes a few sodium channels to open and allows some positively-charged sodium ions to diffuse in). If the membrane potential reaches the threshold potential (generally ~15 mV less negative than the resting potential), the voltage-regulated sodium channels all open. Sodium ions rapidly diffuse inward, & depolarization occurs. February 22, 2025 40 Characteristics of AP Action potentials Need to reach threshold Are all-or-none events Have constant amplitude Do not summate Are initiated by depolarization Involve changes in permeability Rely on voltage-gated ion channels February 22, 2025 41 Action Potential Con’t The generation of APs is prevented by local anesthetics such as procaine and lidocaine, because these drugs block voltage-gated Na+ channels, preventing them from opening in response to depolarization. Without AP graded signals generated in sensory neurons in response to injury, for example cannot reach the brain and give rise to the sensation of pain. February 22, 2025 42 Action Potential Con’t Some animals produce toxins (poisons) that work by interfering with nerve conduction in the same way that local anesthetics do. For example, some organs of the pufferfish produce an extremely potent toxin, tetrodotoxin, that binds to voltage- gated Na+ channels and prevents the Na+ component of the AP February 22, 2025 43 Functions of Action Potentials i. Information delivery to CNS : carriage of all sensory input to CNS. ii. Information encoding :The frequency of APs encodes information iii. In non-nervous tissue APs are the initiators of a range of cellular responses – muscle contraction – secretion (e.g. Adrenalin from chromaffin cells of medulla) February 22, 2025 44 Absolute Refractory Periods During the AP, a second stimulus, no matter how strong, will not produce a second AP. That region of the membrane is then said to be in its absolute refractory period (ARP). The ARP represents the time required for the Na+ channel gates to reset to their resting positions. The ARP ensures that a second AP will not occur before the first has finished. AP cannot overlap and cannot travel backward because of their refractory periods. February 22, 2025 45 Relative Refractory Period A relative refractory period follows the absolute refractory period. A stronger than normal depolarizing graded potential is needed to bring the cell up to threshold, and the AP will be smaller than normal February 22, 2025 46 Uses of Refractory Periods The refractory periods limit the number of AP an excitable membrane can produce in a given period of time. Most neurons respond at frequencies of up to 100 APs per second, and some may produce much higher frequencies for brief periods. RPs contribute to the separation of these APs so that individual electrical signals pass down the axon. The RPs also are the key in determining the direction of AP propagation. February 22, 2025 47 February 22, 2025 48 February 22, 2025 49 February 22, 2025 50 NERVE IMPULSE The neurons are excitable and receives signals from the sensory receptors The signals are relayed via synapses from one neuron to another to the SC & higher centres Up to 200,000 synapses can exist on a single dendritic neuron Nerve excitability is the ability to respond to stimuli. Nerve responds to effective stimulus by generating a propagated nerve impulse while muscle responds by contraction, and a gland responds by secretion. A nerve impulse is a wave of depolarization which propagates along the membrane of the nerve fiber. This impulse is the nerve signal which the nerve fiber conducts to or from the CNS when a neuron is excited by an effective stimulus. Impulse Conduction/Propagation An impulse is simply the movement of action potentials along a nerve/muscle cell membrane. Action potentials are localized (only affect a small area of nerve/muscle cell membrane). When it occurs, only a small area of membrane depolarizes (or 'reverses' potential). However, an action potential elicited at any one point on the an excitable membrane usually excites adjacent portions of the membrane, resulting in the propagation of action potential. When action potential occur at one point, areas of membrane adjacent to each other have opposite charges (the depolarized membrane is negative on the outside & positive on the inside, while the adjacent areas are still positive on the outside and negative on the inside). An electrical circuit (or 'mini-circuit') develops between these oppositely-charged areas (or, in other words, electrons flow between these areas). This 'mini-circuit' stimulates the adjacent area and, therefore, an action potential occurs. This process repeats itself and action potentials move down the nerve cell membrane. This 'movement' of action potentials is called an impulse. Action potential will travel in a direction away from point of stimulus. In a myelinated neuron, action potentials only occur along the nodes and, therefore, impulses 'jump' over the areas of myelin - going from node to node in a process called saltatory conduction (with the word saltatory meaning 'jumping'). Because the impulse 'jumps' over areas of myelin, an impulse travels much faster along a myelinated neuron than along a non-myelinated neuron. February 22, 2025 57 Effect of Myelin on Neuronal Size An unmyelinated neuron would have to be 83-times larger than a myelinated neuron to conduct at the same speed Imagine the impact this would have on brain size – you would probably be walking on your hands with your ears evolved as limbs to assist locomotion February 22, 2025 58 Uses of Myelin Conduction velocity is increased Size requirement is diminished Electrical insulation Reduced cell-energy requirement February 22, 2025 59 Demyelinating Disease In demyelinating disease, the loss of myelin from vertebrate neurons can have devastating effects on neural signaling. In central and peripheral nervous system, the loss of myelin slows the conduction of APs. In addition, when ions leak out of the now- uninsulated regions of membrane between the channel rich-rich nodes of Ranvier, the depolarization that reaches a node may not be above threshold and conduction may fail. February 22, 2025 60 Multiple Sclerosis It is the most common and best known demyelinating disease. It is characterized by a variety of neurological complaints, including fatique, muscle weakness, difficulty walking and loss of vision. Can be inherited or autoimmune disorders. One way that Multiple Sclerosis and other demyelinating illnesses are diagnosed is through the use of a nerve conduction test. This test measures the combined strength of AP and the rate at which they are conducted as they travel down neurons. February 22, 2025 61 February 22, 2025 62 SYNAPSES A synapse is a functional and structural specialized junction between two neurons, at which the electrical activity in a presynaptic neuron influences the electrical activity of the postsynaptic neuron. Anatomically, synapses include parts of the presynaptic and postsynaptic neurons and the extracellular space between these two cells. According to recent estimates, there are more than 100 trillion! synapses in the CNS. There are two types of synapses: Electrical synapses and Chemical synapses. At electrical synapses, current flows through gap junctions (specialized membrane channels that connect two cells). On the other hand, chemical synapses enable cell-to-cell communication via the secretion of neurotransmitters. SYNAPSES Contd Hundreds or thousands of synapses from many different presynaptic cells can affect a single postsynaptic cell (convergence), and a single presynaptic cell can send branches to affect many other postsynaptic cells (divergence). Convergence allows information from many sources to influence a cell’s activity; divergence allows one cell to affect multiple pathways Electrical Synapses Electrical synapse pass an electrical signal, or current, directly from the cytoplasm of one cell to another through gap junctions. Electrical synapses occur mainly in neurons of the CNS. They are also found in glia cells, in cardiac and smooth muscle, and in non excitable cells that use electrical signals, such as the pancreatic beta cell. The primary advantage of electrical synapses is rapid conduction of signals from cell to cell that synchronizes activity within a network of cells. Chemical Synapses The vast majority of synapses in the nervous system are chemical synapses, which use neurotransmitters to carry information from one cell to the next. The axon of the presynaptic neuron ends in a slight swelling, the axon terminal, which holds the synaptic vesicles that contain neurotransmitter molecules. An extracellular space, the synaptic cleft, separates the presynaptic and postsynaptic neurons and prevents direct propagation of the current from the presynaptic neuron to the postsynaptic cell. Instead, signals are transmitted across the synaptic cleft by means of a chemical messenger- a neurotransmitter- released from the presynaptic axon terminal. Chemical Synapses Con’t Neurotransmitter release is initiated when an AP reaches the presynaptic terminal membrane. A key feature of neuron terminals is that they also possess voltage-gated Ca²ᶧ channels. Depolarization during the AP opens these Ca²ᶧ channels, and because the electrochemical gradient favors Ca²ᶧ influx, Ca²ᶧ flows into the axon terminal. CHEMICAL TRANSMISSION A key feature of all chemical synapses is the presence of small, membrane-bounded organelles called the synaptic vesicles within the presynaptic terminal Neurotransmitters There are more than 100 types of neurotransmitters and they virtually undergo a similar cycle of use: synthesis and packaging into synaptic vesicles Release from the presynaptic cell Binding to postsynaptic receptor Rapid removal and degradation The secretion of the neurotransmitters is triggered by the influx of Ca 2+ through the voltage-gated channels that give rise to transient increase in Ca2+ concentration within the presynaptic terminal. CHEMICAL TRANSMISSION – Cont’d The rise in the Ca2+ causes synaptic vesicles to fuse with presynaptic plasma membrane and release their contents into the space between the pre- and postsynaptic cells. Neurotransmitters released therefore evoke postsynaptic electrical Responses (EPSP/IPSP) by binding to members of a diverse group of neurotransmitter receptors. These receptors give rise to electrical signals by transmitter-induced opening or closing of the ion channels. NORADRENERGIC TRANSMISSION Synthesis of Norepinephrine/Epinephrine The adrenal medulla that secretes epinephrine/adrenaline and norepinephrine/noradrenaline, is an important component of the sympathetic nervous system. As a result, the sympathetic nervous system and adrenal medulla are often referred to as the sympathoadrenal system. Norepinephrine is one of the five well-established biogenic amine neurotransmitters: 3 catecholamines (dopamine, norepinephrine and epinephrine), histamine and serotonin. Noradrenaline like the other 2 catecholamines are derived from tyrosine (which is a product of phenylalanine). Synthesis of Norepinephrine/Epinephrine This reaction is catalyzed by phenylalanine hydroxylase in the liver) in a reaction catalyzed by tyrosine hydroxylase (in the neuron) requiring O2 as a co-substrate and tetrahydrobiopterin as a cofactor to form DihydrOxyPhenylAlanine (DOPA). Norepinephrine synthesis requires dopamine β-hydroxylase that catalyzes the production of noradrenaline from Dopamine. In the central adrenergic fibers (neurons of the thalamus, hypothalamus and midbrain) and in the adrenal medulla, noradrenaline is converted to adrenaline by Phenylethanolamine -N-methyl Transferase (PNMT). This enzyme is not found in the peripheral adrenergic fibers. Synthesis of Norepinephrine/Epinephrine Fate / Degradation of Norepinephrine/epinephrine Noradrenaline is the loaded into synaptic vesicles via the Vesicular MonoAmine Transporter (VMAT) same as with dopamine. Norepinephrine is cleared from the synaptic cleft by Norepinephrine Transporter (NET) mainly in the nerve terminals which is also capable of taking up dopamine to be recirculated. Small amount of Noradrenaline like dopamine is oxidized by MonoAmine Oxidase (MAO) to inactive products. MAO is found in nerve terminals and other organs like liver and kidney. Some small amount is methylated to inactive products by Catechol O-Methyl-Transferase (COMT) enzymes found in the many tissues such as kidney and brain but not in the nerve terminals. Epinephrine is mainly methylated in various organs by COMT. CHOLINERGIC TRANSMISSION Ach is synthesized in nerve terminals from the precursors acetyl coA and choline in the recation catalyzed by choline acetyl transferase (CAT) Choline is present in plasma at a high concentration (about 10 mM) and is taken up into cholinergic neurons by a high - affinity Na+/choline transporter. After synthesis in the neuroplasm a vesicular Ach transporter loads approx 10, 000 molecules of Ach into each cholinergic vesicle. In contrast to most other small-molecular neurotransmitters, the postsynaptic actions of Ach at many cholinergic synapses (the NMJ in particular) is not terminated by reuptake but by a powerful hydrolytic enzyme, Acetylcholinesterase (AchE) MUSCLE PHYSIOLOGY Characteristics of a Muscle excitability – ability to respond to stimuli contractility - ability to shorten in length extensibility – ability to stretch when pulled elasticity – ability to return to original shape and length after contraction or extension Functions of a Muscle Motion Maintenance of posture Heat production Respiration, heart beat, constriction of vessels Communication (voice) SKELETAL MUSCLE Skeletal muscles consist of about 40% of the total body muscle mass It is attached to bones and moves skeleton It is also called a striated muscle because of the appearance of some dark lines under the microscope It is a voluntary muscle i.e. under the control of will Detailed Structure of a Skeletal Muscle Skeletal muscles are usually attached to a bone by tendons that are composed of connective tissue. The connective tissue that encloses the entire muscle is called the epimysium. Within the epimysium are numerous subunits or bundles of skeletal muscles called fascicles. The fascicles are surrounded by a connective tissue called the Perimysium. Each fascicle is composed of numerous muscle fibers or muscle cells, each of which is covered by another connective tissue called the Endomysium. Structural components of a skeletal muscle To understand how a muscle contracts, you need to know a bit about the structure of muscle cells. All muscles require ATP to produce movement. Thus, muscles are Chemotransducers. Most muscles have their fibers extending the entire length of the muscle and each fiber is innervated by only one nerve ending, located near the middle of the fiber. The cell membrane of a muscle fiber is called the Sarcolemma that contains the true cell membrane and an outer coat of a thin layer of polysaccharide material that contains numerous collagen fibers. Each muscle cell consists of many fibrils (or myofibrils) that are made up of long protein molecules called the myofilaments. Each myofibril has two types of myofilaments: thick Myosin and thin actin myofilaments. This filaments partially interdigitate thereby causing the myofibrils to have alternate light and dark bands (striations) The light bands contain the actin filaments and are termed I bands because they are isotropic to polarized light. The dark bands contains the myosin filaments as well as the ends of the actin filaments where they overlap the myosin and are called the A bands. The end of the actin filaments are attached to a Z disc. The portion of a myofibril (or of the whole muscle fiber) that lies between two successive Z discs is called a Sarcomere. The myofibrils of a muscle fiber are suspended in a matrix called the Sarcoplasm composed of usual intracellular material. Fluid of the sarcoplasm contains large amount of K+, Mg2+, Po4-, some enzymes and abundant mitochondria that lie parallel to the myofibrils. Why? Also in the sarcoplasm is an extensive endoplasmic reticulum called the sarcoplasmic reticulum. This structure is extremely important in the physiology of contraction of a muscle fiber. Motor Unit A skeletal muscle contains many muscle fibers and the contraction of each fiber is controlled by one motor neuron extending from the CNS. However, the motor neurons leading to a muscle from the CNS is less than the number o of fibers in the muscle. This means that each motor neuron must control several muscle fibers. Therefore, one motor neuron, together with the group of muscle fibers it controls is called a motor unit. All the muscle fibers in a motor neuron contract simultaneously together because of an AP thereby acting as one functional unit. The number of fibers in one motor unit varies from just a few to several hundreds. Finer control and strength of contraction depends on the number of motor units active at a time. Increasing the number of motor units that are activated at a time is known as motor unit recruitment. Motor Units and All-or-None Principle When a given muscle is stimulated by its motor neuron, it either contracts to the fullest extent possible or it does not contract at all. This is the all-or-none principle of a muscle cell. With this, how can a muscle in the upper limb allow you to pick a pencil (light) or a bucket of water (heavy)? Simple Muscle Twitch A single threshold stimulus to a muscle produces a contraction of short duration followed by a relaxation. Such a response is called a simple muscle twitch which has three phases. The time between the application of the stimulus and the beginning of contraction is the latent period. It is about 0.01sec. The period during which the muscle produces tension, measured from the beginning of the tension development to its peak, is the contraction period. The period beginning at the peak of the tension development lasting until the muscle ceases to produce force is the relaxation period. Isometric and Isotonic Contractions Contraction may cause a muscle to shorten or may cause tension in the muscle to increase while muscle length remains constant. A contraction in which the muscle length remains the same while tension in the muscle increases is called an Isometric contraction. However, when a muscle shortens while its tension remains the same, the contraction is known as an Isotonic contraction. Factors that Affect Simple Muscle Twitch A) Type of Skeletal Muscle Fibers Skeletal muscles differ from one another in: the amounts of myoglobin, number of mitochondria, levels of glycolytic enzymes they possess and in the number of capillaries carrying blood to them. These structural differences are reflected in the functional characteristics of the fibers. They are therefore grouped as: Slow-twitch Red Fibers: Have a longer contraction time than other fiber types; i.e. they contract slowly Are resistant to fatigue as they primarily depend on aerobic processes to obtain their ATP for contraction. Have abundant blood supply because of their high demand for oxygen. Have high myoglobin content Have abundance of mitochondria. Are red because they contain large amount of reddish iron- containing pigments: myoglobin, cytochromes in mitochondria and hemoglobin in the red blood cells that reaches them. Are adapted for long slow rate posture maintaining contractions. E.g. soleus muscle. Fast twitch White Fibers Have short contraction time i.e. they contract quickly. This is partly due to extensive SR that allows rapid release of Ca2+. Rely mainly on anaerobic process for their source f ATP. Have abundant glycolytic enzymes for energy production but few mitochondria and less myoglobin content Do not have extensive blood supply of other fiber types as theyneed less oxygen They fatigue quickly because of their anaerobic nature of energy production They are specialized for fine movements. E.g. external occular and finger muscles. Fast twitch Red (Intermediate) Fibers They are like slow-twitch red fibers in that they contain large amount of myoglobin. Have extensive blood supply and rely primarily on aerobic ATP production. They are resistant to fatigue, although not quite as the slow-twitch red fibers. Have short contraction time. They represent most of the red muscle fibers in humans B) Temperature The contraction of muscle is a result of many biochemical reactions, therefore an increase in temperature accelerates these chemical reactions and thereby increasing the muscle twitch. An increase in temperature also reduces viscosity of the muscle and facilitates the process of contraction. Height of contraction may also increase due to increase in temperature. Decrease in temperature has an opposite effect on the muscle. C) Muscle Fatigue Muscle fatigue is an inability of a muscle fiber to contract after an extended period of repeated stimulation or the decrease in muscular activity due to repeated stimuli The fatigue can be due to depletion of glycogen and resulting in lack of ATP Other factors that contribute to fatigue include: increase in temperature which causes heat; accumulation of lactate during anaerobic respiration that results in H+ rise thereby raising the pH; increase in pressure can cause decrease in blood flow. Continual stimulation of the NMJ can also cause fatigue. D) Staircase Effect (Treppe) If a well rested muscle is stimulated to contract several times in succession, each contraction is slightly stronger than the preceding one. This is called staircase effect or Treppe. CHANGES DURING MUSCULAR CONTRACTION Changes taking place during muscular contraction are as follows: Electrical changes Physical changes Histological (molecular) changes Chemical changes ELECTRICAL CHANGES Electrical events occur in the muscle during resting condition as well as active conditions Electrical potential in the muscle during resting condition is called resting membrane potential Electrical potential when the muscle is stimulated is called muscle action potential. RESTING MEMBRANE POTENTIAL It is also called transmembrane potential Is the electrical potential difference (voltage) across the cell membrane (between inside and outside of the cell) under resting condition. In human skeletal muscle, the RMP is –90 mV. It is maintained by sodium-potassium ATPase which actively pump out three sodium and actively pump in two potassium, thereby making the potential inside polarized (more negative compare to the outside). ACTION POTENTIAL Action potential in skeletal muscle is a series of electrical changes that occur in the membrane potential of the skeletal muscle when stimulated. Action potential occurs in two phases: Depolarization Repolarization. PHYSICAL CHANGES Physical change is the change in length of the muscle fibers or change in tension developed in the muscle (Saladin, 2012). Depending upon this, the muscular contraction can be isotonic contraction and isometric contraction. MOLECULAR CHANGES In relaxed state, the thin actin filaments from opposite ends of sarcomere are away from each other leaving a broad ‘H’ zone. During contraction, actin (thin) filaments glide over myosin (thick) filaments and form actomyosin complex (Tate, 2012). The molecular mechanism responsible for formation of actomyosin complex is the Excitation-contraction coupling (or Sliding mechanism) The Excitation-Contraction Coupling Excitation-contraction coupling is the process that occurs in between the excitation and contraction of the muscle. This is called Sliding mechanism, ratchet or walk-along mechanism When a muscle is excited, AP generated in the sarcolemma is rapidly spread into the muscle fiber through the T- tubules. When the action potential reaches the cisternae of ‘L’ tubules, these cisternae are excited and release calcium into the sarcoplasm. The calcium ions released bind with troponin C. This produces some change in the position of troponin molecule which in turn, pulls tropomyosin molecule away from F actin. Due to the movement of tropomyosin, the active site of F actin is uncovered and exposed. Immediately the head of myosin gets attached to the actin binding sites After binding with active site of F actin, the myosin head is tilted towards the arm so that the actin filament is dragged along with it METABOLIC/CHEMICAL CHANGES Muscle contraction requires energy. The immediate source of energy is the energy-rich organic phosphate derivatives in the muscle, namely ATP and phosphocreatine. a) Energy for contraction is provided by the hydrolysis of ATP to ADP. Myosin adenosine triphosphatase protein acts as the enzyme in the reaction. ATP + H2O ADP + H3PO4 + Energy (12, 000 cal). b) ADP which is formed during ATP breakdown, is immediately utilized for the resynthesis of ATP by the addition of a phosphate group. This reaction is endothermic that requires energy. ADP + CP ATP + Creatine The energy is supplied from phosphocreatine or the breakdown of glucose (derived from muscle glycogen or bloodstream) to CO2 and H2O. Phosphocreatine is an energy-rich phosphate compound that is hydrolysed to creatine and phosphate groups to release considerable energy. ADP + Phosphocreatine ATP + Creatine Oxygen Glucose + 2ATP 6CO2 + 6H2O + 36ATP Most of the energy for the phosphocreatine and ATP resynthesis comes from the breakdown of glucose to CO2 and H2O. The glucose is first degraded to pyruvic acid that enters the citric acid cycle. The pyruvic acid is then oxidized to CO2 and H2O with liberation of large amount of energy in the form of ATP. c) If oxygen supply to the contracting muscle is insufficient, glucose breakdown to pyruvic acid which is reduced to lactic acid. This process is anaerobic and referred to anaerobic glycolysis. It is associated with production of smaller number of ATP. anaerobic Glucose Lactic acid + 2ATP Therefore, it is clear that in the presence of sufficient oxygen, one mole of glucose provides more molecules of ATP than during anaerobic process. d) Skeletal muscles also take up free fatty acids from the blood and oxidize them to CO2 and H2O. These free fatty acids are probably the major substrates for muscle at rest and during recovery after contraction. Free fatty acids CO2 + H2O + ATP NEUROMUSCULAR JUNCTION (NMJ) NMJ or myoneural junction is a place where contraction of skeletal muscle occurs in response to action potentials that travel down somatic motor axons originating in the CNS. Neuromuscular transmission is defined as the transfer of information from motor nerve ending to the muscle fiber through NMJ Acetylcholine (Ach) is the neurotransmitter released at NMJ by the presynaptic neuron It binds to nicotinic Ach receptor on postsynaptic membrane It produce motor end plate potential which is graded to generate action potential in the muscle fiber resulting in contraction. Neuromuscular blockers Neuromuscular blockers are the drugs, which prevent transmission of impulses from nerve fiber to the muscle fiber through the neuromuscular junctions Curare prevents the neuromuscular transmission by combining with Ach receptors Bungarotoxin is a toxin from the venom of deadly Snakes that blocks Ach receptors Succinylcholine block the neuromuscular transmission by acting like acetylcholine and keeping the muscle in a depolarized state. Botulinum Toxin prevents release of acetylcholine from axon terminal into the neuromuscular junction. Neuromuscular Stimulators Neuromuscular junction can be stimulated by drugs that inactivate acetylcholinesterase. It increase the activity of acetylcholine at NMJ. These drugs are called acetylcholinesterase inhibitors. They include neostigmine, physostigmine and diisopropylfluorophosphate. Myasthenia Gravis This is a hereditary disease of skeletal muscle that is more prevalent in females than males. It is characterized by marked weakness and easy fatigability of muscles which may be due to: Presence of curate-like substance in the blood Motor end plate secretes small amount of Ach Increased activity of the enzyme cholinesterase. This condition can be treated with drugs that inhibit cholinesterase activity like neostigmine. CARDIAC MUSCLE It is the type of muscle found in the heart. It undergoes rhythmic contractions continuously throughout lifetime. It is therefore an unusually active tissue. Unlike the skeletal muscle, cardiac muscle contractions are intrinsic. Myofilaments in one myofibril Like the skeletal muscle, it may be continuous with possesses striations. myofilaments in adjacent myofibril. Myofibrils are present though not Cardiac muscle cell has a as distinct as those in the skeletal single nucleus that the muscle. myofilaments separate to bypass. There is a presence of large and numerous mitochondria with less developed sarcoplasmic reticulum that is not closely associated with the T-tubules. Cardiac muscle requires increase level of Ca2+ in the sarcoplasm for contraction, however the Ca2+ comes from outside the muscle cell in addition to that released from the sarcoplasmic reticulum. The cardiac muscles possess intercalated discs that connect the adjacent cells and having two types of membranes (desmosomes and gap junctions) connecting the cells each with distinct functions. The desmosomes are for strong attachments between the cells while the gap junctions allow electrical signals to pass from one cell to another. As a result of these electrical connections (gap junctions), the cardiac muscles act as an electrically continuous sheet which is referred to as functional syncytium that enables the excitation to spread to all the parts of the cell. Excitation-Contraction Coupling Like in the skeletal muscles when an AP passes over the cardiac muscle membrane, there is a release of Ca2+ into the sarcoplasm from the SR, leading to the sliding action of actin over the myosin. In addition to the Ca2+ released from the cisternae of the SR, a large quantity of extra Ca2+ diffuses from the ECF via the T-tubules (that have large diameters) without which the contraction of the muscle will be greatly reduced. SMOOTH MUSCLE It is an involuntary muscle It includes muscles of the viscera (e.g., in walls of blood vessels, intestine and other 'hollow' structures and organs in the body). It is innervated by the Autonomic Nervous System (ANS). It is a spindle-shaped cell and the cells are typically arranged in sheets. They lack striations. Cells do not have t-tubules and have very little sarcoplasmic reticulum. Cells do not contain sarcomeres but the myofibrils are made up of thick and thin myofilaments but not regularly arranged into sarcomeres. These muscles can be found in the blood vessels, the GIT, ducts of glands, respiratory passages, urinary bladder, and genital tract. They can also be found in erector pili muscles attached to the hair follicles in the skin. Contractions of smooth muscle fibers in the walls of the hollow organs cause the movement of food or fluids within or down the organs Types of Smooth Muscle Single Unit Smooth Muscle Found in the stomach, intestine, urinary tract, reproductive tract and all small-diameter vessels. They respond to excitation as a single functional unit rather than individual cells. The excitation is triggered by pacemaker cells via gap junction. In this way, the electrical signals in the body pass from one cell to another. Since they are electrically excited almost simultaneously, they contract as a unit. Multi vs. Single-Unit Muscle Figure 12.35 This is the reason why the smooth muscle is similar to cardiac muscle in many ways. Multi Unit Smooth Muscles They are found in the eyes, erector pili muscle, large blood vessels and in the passage ways to the lungs. They do not have pacemaker cells or gap junctions, so contraction is initiated by neural signals. In multi-unit smooth muscles, each muscle receives a branch of an axon of a nerve cell hereby being controlled individually, although simultaneous neural signals reaching them may cause them to contract together. Control of Smooth Muscle Contraction Smooth muscle contraction in some body locations is similar to that of cardiac muscle as it is initiated by a pace maker cells within the muscle tissue and controlled by involuntary neural signal. This type of smooth muscle is called single unit smooth muscle. Smooth muscles in other locations have their contractions triggered by the nervous system. This type is called the multi-unit smooth muscle. This is similar to that in the skeletal muscle but the neural signals are involuntary. The control of the two types of the muscles is by the ANS. Contraction of Smooth Muscle Like in skeletal and cardiac muscles, the smooth muscle possess contractile elements called the myofilaments, though not regularly arranged as sarcomeres. The thin filaments in smooth muscle do not contain troponin but contraction is triggered by an increase in the levels of Ca2+ in the sarcoplasm. Rather than binding to troponin, the Ca2+ binds to a protein called Calmodulin which results in the activation of myosin kinase (on one of the light chains of myosin chain) which is phosphorylating enzyme. The light chain that is phosphorylated is called the regulatory chain. After the phosphorylation, the myosin head becomes capable of binding with the actin filament and proceeding the entire cycle of contraction as in the skeletal muscle. When the Ca2+ is below a critical level, the process automatically reverses itself except for the phosphorylation of the myosin head light chain which requires an enzyme called myosin phosphatase. Smooth muscle contractions are slower and develop less force than those in the skeletal muscle. While a skeletal muscle twitch lasts a fraction of second, that in the smooth muscle may last for several seconds. Apart from the ANS, the smooth muscle can be influences other factors such as: hormones like epinephrine, estrogen, and oxytocin. How Do these hormones carry such an action? Calcium ions play major regulatory roles in the contraction of both smooth and skeletal muscle, but the calcium that enters the cytosol of stimulated smooth muscles binds to calmodulin, forming a complex that activates the enzyme that phosphorylates myosin, permitting its binding interactions with actin. Summary on the Comparison of the Characteristics of Muscles Table 12.2 AUTONOMIC NERVOUS SYSTEM INTRODUCTION The term “autonomic” implies independent, self-controlling function without conscious effort. Derived from ‘auto’ and ‘nomos’ (law) The ANS therefore helps to regulate our internal environment (visceral functions). The ANS is activated mainly by centers that are located in the spinal cord, brain stem and the hypothalamus. It comprises of the Sympathetic and parasympathetic nervous system but Enteric Nervous System (ENS) can also be considered as part of the ANS. INTRODUCTION CONT’D Portions of cerebral cortex, especially the limbic cortex can transmit impulses to the lower centers thereby influencing autonomic control. The ANS also operates via visceral reflexes. The autonomic nervous system (ANS) regulates physiologic processes without conscious control. The ANS consists of two sets of nerve bodies: preganglionic and postganglionic fibers. The two major divisions of the ANS are the sympathetic and parasympathetic systems. Divisions of the Autonomic Nervous System Sympathetic The preganglionic cell bodies of the sympathetic system are located in the intermediolateral horn of the spinal cord between T1 and L2 or L3. The sympathetic ganglia are adjacent to the spine and consist of the vertebral (sympathetic chain) and prevertebral ganglia Long fibers run from these ganglia to effector organs, including the smooth muscle of blood vessels, viscera, lungs, scalp (piloerector muscles), and pupils; the heart; and glands (sweat, salivary, and digestive). Parasympathetic The preganglionic cell bodies of the parasympathetic system are located in the nuclei of the brain stem and sacral portion of the spinal cord (S2-S4). These preganglionic fibers exit the brain stem with the 3rd, 7th, 9th, and 10th cranial nerves. Parasympathetic ganglia are located in the blood vessels of the head, neck, and thoracoabdominal viscera; lacrimal and salivary glands; smooth muscle of viscera and glands. Functions of the ANS The ANS fxns are categorised into 3: – Maintaining homeostatic condition within the body – Cordinating the body’s response to exercise and stress – Assisting the endocrine system to regulate reproduction Functions of the ANS Cont’d The two divisions of the ANS are dominant under different conditions. The sympathetic system is activated during emergency “fight-or-flight” reactions and during exercise. The parasympathetic system is predominant during quiet conditions (“rest and digest”). As such, the physiological effects caused by each system are quite predictable. In other words, all of the changes in organ and tissue function induced by the sympathetic system work together to support strenuous physical activity and the changes induced by the parasympathetic system are appropriate for when the body is resting. Functions of the ANS Cont’d The preganglionic axons, mostly myelinated but relatively conducting β fibres, synapses on the cell bodies of postganglionic neurons outside the CNS Each axon diverges to 8-9 postganglionic neuron leading to a diffuse autonomic output The postganglionic neurons have their cell bodies in d autonomic ganglia and synapse on effector organs Most are unmyelinated C-fibres The sympathetic division is adrenergic due to adrenaline-like action resulting from its activation The parasympathetic is cholinergic due to Ach-like action Functions of the ANS Cont’d The sympathetic preside over utilization of metabolism resources and emergency responses The parasympathetic presides over restoration and build up of body reserves & elimination of waste In reality, most of the organs in the body supplied by d ANS received both divisions In some instance, they antagonize each other e.g HR control In other instances, they are synergistic e.g GI secretions Some organs e.g skin and blood vessels have only d sympathetic innervation Functions of the ANS Cont’d The smooth muscle in the hollow viscera received both sympathetic and parasympathetic fibres Activity of one may increases activity of the smooth muscle while the other decreases the smooth muscle activity In sphincteric muscle, both are excitatory but one supplies the constrictor component while the other supplies the dilator component NE spreads farther has more prolong action than Ach NE, epinephrine and dopamine are found in the plasma unlike Ach AUTONOMIC GANGLIA These are small swellings along the course of the autonomic nerves that contain a collection of nerve cells. Efferent autonomic fibers that arise from the lateral horn cells are called the preganglionic fibers which are thick, white and myelinated fibers. The preganglionic fibers enter the autonomic ganglia where they take either of two courses: i) terminate into several nerve terminals in the ganglion that relay impulses into the ganglionic nerve cells. Postganlionic fibers emerge from these ganglionic fibers and proceed to supply the effector organs. These are thin, gray and unmyelinated fibers. AUTONOMIC GANGLIA – Cont’d ii) Pass via the ganglion uninterrupted without relay and emerge on the other side still as preganglionic fibers to proceed to the adrenal medulla or to another ganglion where they terminate and relay The postganglionic nerve fibers emerge from the later ganglion to supply the effector organs. Types of the Autonomic Ganglia a) Lateral or Paravertebral Ganglia: Form sympathetic chain on both sides of the vertebral column with each chain forming 23 ganglia (3 cervical – superior, middle and inferior ganglia; 12 thoracic; 4 lumbar and 4 sacral) connected to each other by nerve fibers. b) Collateral or Prevertebral ganglia: These are celiac, the superior and inferior mesenteric ganglia. They are found along the course of sympathetic nerves, midway between the spinal cord and the viscera. These are sympathetic ganglia i.e. sites of relay for sympathetic nerves only. c) Terminal Ganglia: These are present near or inside the effector organ e.g. the eye, heart and the stomach. They are parasympathetic ganglia i.e. sites of relay for parasympathetic nerves only. The Autonomic ganglia serve as: relay stations, expansion as well as distribution centers. REGULATORY SYSTEMS OF THE ANS Autonomic reflexes represent the simplest level of ANS control The ANS involvement with the limbic system, hypothalamus, solitary nucleus of the medulla and other brain stem nuclei has explained the ANS regulation. In fact, the limbic system has been termed the “cerebral cortex of Stimulation of the limbic the ANS”. So the limbic system system areas can evoke a broad represents one of the highest levels range of feelings and behaviors, of the hierarchy of normal control of including rage, anger, fear, and aggression. the ANS. REGULATORY SYSTEMS OF THE ANS – Cont’d In addition, stimulation of the limbic system, either directly or by input from the senses, can evoke ANS-mediated physiological changes such as increased heart rate, sexual arousal, and nausea. Autonomic Functions of the Hypothalamus Hypothalamus is known as the main ganglion of the ANS and the activation of its different parts produces a variety of coordinated autonomic responses: Activation of the dorsal hypothalamus, for e.g. increases blood pressure, intestinal motility, and intestinal blood supply but decreases supply to the skeletal muscles. These are associated with feeding behavior. However, activation of ventral hypothalamus increases blood pressure and the blood supply to the skeletal muscles but decreases intestinal motility and blood flow to the intestines. These are associated with flight or flight responses. Neurotransmitters of the ANS Two most common neurotransmitters released by neurons of the ANS are acetylcholine (cholinergic) and norepinephrine/noradrenaline (adrenergic). Acetylcholine: – All preganglionic nerve fibers – All postganglionic fibers of the parasympathetic system – Sympathetic postganglionic fibers innervating sweat glands Adrenaline: – In most sympathetic postganglionic fibers Peptides like VIP, Subst P, etc released by peptidergic neurons Co-transmitters e.g ATP & neuropeptide Y are released with NE, VIP released with Ach Non-Ach, Non-adrenergic neurons release NO Receptor types in the ANS Adrenergic/Adrenoceptors α1 receptor – Located on vascular smooth muscle of the skin & splanchnic region, GIT and Bladder sphincters & radial muscle of the iris – Produces excitation (constriction/contraction) – Equally sensitive to NE and epinephrine but only NE is present in concentration high enough to excite the receptor – MOA: formation of IP3 and ↑intracellular Ca2+ Receptor types in the ANS cont’d α2 receptor – Located in the presynaptic nerve terminals, GI wall – Often produces inhibition (relaxation/contraction) – MOA: inhibit adenylate cyclase and ↓cAMP Β1 receptor – Located in the SA node, AV node & ventricular muscle, kidneys, – Produces excitation (↑HR, ↑conduction velocity, ↑contraction) – Sensitive to both NE & epinephrine more than α1 – MOA: activation of adenylate cyclase & ↑cAMP Receptor types in the ANS cont’d Β2 receptor – Located on vascular smooth muscle in skeletal muscle, bronchial smooth muscle & wall of GIT and bladder – Prod relaxation (dilation of bronchioles, relaxation of bladder) – More sensitive to epinephrine than NE – MOA: same as β1 Cholinergic/cholinoceptors – Nicotinic – muscarinic Adrenergic Drugs/Agonists/Protagonists/Symphatomimietic Drugs These are drugs that mimic the actions of norepinephrine or epinephrine through the following mechanisms: i) Stimulating the release of the transmitter. Example of these include Amphetamine and Ephedrine ii) Inhibiting the action of MAO enzyme. Example, Ephedrine and Hydrazine iii) Direct stimulation of receptors. Example norepinephrine and epinephrine (α and β), phenylephrine (α1), clonidine (α2), Dobutamine (β1), Salbutamol (β2) and isoproterenol (β1 and β2) Agonists and antagonists of adrenergic receptors, such as the β blocker propanolol are used clinically for a variety of conditions ranging from cardiac arrhythmias to migraine headaches. However, most of the actions of these drugs are on smooth muscle receptors particularly on cardiovascular and respiratory systems. Anti-Adrenergic Drugs/Adrenergic Blockers/Antagonists/Symphatolytic Drugs These are drugs that block the actions of norepinephrine and epinephrine and they produce their actions via the following mechanisms: i) Inhibiting the synthesis of norepinephrine. Example is Aldomet (that inhibits β-hydroxylase leading to the formation of a false transmitter). ii) Preventing the release of the transmitter. Example is Guanethidine iii) Direct blocking of the receptors. Examples are: Prazosin (α1), Yohimbine (α2), Phentolamine (α1 and α2), Atenolol (β1), Butaxamine (β2) and Propranolol (β1 and β2) Effects of the ANS divisions Effects of the ANS divisions EFFECTOR ORGAN PARASYMPATHETIC SYMPATHETIC Pupil Constriction Dilation (α1) Ciliary msc Contraction Relaxation (β2) Lacrimal gland ↑secretion - Nasal gland ↑ secretion Inhibition (α1) Salivary gland ↑secretion Inhibition (β) Lungs (glands) ↑secretion ↓secretion (α1, β2) GI glands ↑secretion Inhibition Liver - Glycogenolysis, gluconeogensis (α1, β2) Pancreas (insulin) - ↓secretion (α2) Adrenal medula - ↑secretion of epi Ureter Relaxation Contraction (α1) Uterus variable Contraction (α1) Comparison between the ANS divisions Characteristic Sympathetic Parasympathetic Somatic Origin of Nuclei of T1-L3 CN nuclei - preganglionic III,VII,IX,X neuron Nuclei of S2-4 Length of Short Long - preganglionic axon Neurotransmitter Ach Ach - in ganglion Receptor type in Nicotinic Nicotinic - ganglion Length of Long Short - postganglionic axon Effector organ Smooth & cardiac Smooth & cardiac Skeletal msc msc, glands msc. glands Neurotransmitter NE (except sweat Ach Ach in effector organ glands) Receptor type in α1, α2, β1, β2 Muscarinic Nicotinic ANY QUESTION? TEST