Exercise, Glucose Transport, and Insulin Sensitivity PDF
Document Details
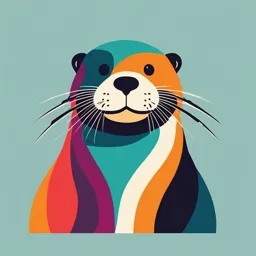
Uploaded by MiraculousDravite
Universidade de Brasília
1998
Laurie J. Goodyear, Barbara B. Kahn
Tags
Related
- EHR522 Week 1 Tutorial PDF
- Week 4 Lecture Slides - Exercise for Metabolic & Renal Conditions PDF
- Week 5 Lecture Slides (Part 1 & Part 2) PDF
- Exercise Programming and Delivery for People Living with Dementia UNSW PDF
- Week 5 - Diabetes - Type I, Type II and Gestational PDF
- Diabetes Mellitus - Exercise 6 PDF
Summary
This review article discusses the effects of exercise on glucose transport and insulin sensitivity in skeletal muscle. It details the molecular mechanisms and clinical implications for people with diabetes. The authors emphasize the importance of long-term exercise in preventing and managing diabetes.
Full Transcript
Annu. Rev. Med. 1998. 49:235–61 Copyright © 1998 by Annual Reviews Inc. All rights reserved EXERCISE, GLUCOSE TRANSPORT,...
Annu. Rev. Med. 1998. 49:235–61 Copyright © 1998 by Annual Reviews Inc. All rights reserved EXERCISE, GLUCOSE TRANSPORT, AND INSULIN SENSITIVITY Laurie J. Goodyear, PhD Annu. Rev. Med. 1998.49:235-261. Downloaded from www.annualreviews.org Research Division, Joslin Diabetes Center, Brigham and Women’s Hospital, and by Texas A&M University - Commerce on 07/19/13. For personal use only. Department of Medicine, Harvard Medical School, Boston, Massachusetts 02215; e-mail: [email protected] Barbara B. Kahn, MD Diabetes Unit, Division of Endocrinology and Metabolism, Beth Israel Deaconess Medical Center, and Department of Medicine, Harvard Medical School, Boston, Massachusetts 02215; e-mail: [email protected] KEY WORDS: skeletal muscle, GLUT4, diabetes, insulin resistance, physical training ABSTRACT Physical exercise can be an important adjunct in the treatment of both non–insulin-dependent diabetes mellitus and insulin-dependent diabetes mellitus. Over the past several years, considerable progress has been made in understanding the molecular basis for these clinically important effects of physical exercise. Similarly to insulin, a single bout of exercise increases the rate of glucose uptake into the contracting skeletal muscles, a process that is regulated by the translocation of GLUT4 glucose transporters to the plasma membrane and transverse tubules. Exercise and insulin utilize different sig- naling pathways, both of which lead to the activation of glucose transport, which perhaps explains why humans with insulin resistance can increase muscle glucose transport in response to an acute bout of exercise. Exercise training in humans results in numerous beneficial adaptations in skeletal muscles, including an increase in GLUT4 expression. The increase in muscle GLUT4 in trained individuals contributes to an increase in the responsive- ness of muscle glucose uptake to insulin, although not all studies show that exercise training in patients with diabetes improves overall glucose control. However, there is now extensive epidemiological evidence demonstrating that long-term regular physical exercise can significantly reduce the risk of developing non–insulin-dependent diabetes mellitus. 235 0066-4219/98/0201-0235$08.00 236 GOODYEAR & KAHN INTRODUCTION It has long been recognized that physical exercise has important benefits for people with diabetes (1). In the context of overall glucose homeostasis, a sin- gle bout of exercise can markedly increase rates of whole-body glucose dis- posal (2, 3) and increase the sensitivity of skeletal muscle glucose uptake to in- sulin (4). These effects can last for several hours after the exercise ends (5–7). Both the acute and persistent effects of exercise on glucose uptake and dis- posal have important implications for individuals with diabetes in terms of chronic metabolic control and acute regulation of glucose homeostasis. In ad- Annu. Rev. Med. 1998.49:235-261. Downloaded from www.annualreviews.org dition to the metabolic effects of a single exercise session, recent epidemiol- by Texas A&M University - Commerce on 07/19/13. For personal use only. ogical studies have determined that regular physical exercise can reduce the risk of developing non–insulin-dependent diabetes (NIDDM) (8–10). In the first part of this chapter we review studies that have focused on elucidating the cellular basis for the changes in glucose uptake and insulin sensitivity that oc- cur in skeletal muscle in response to a single exercise session. The second part of this chapter discusses investigations of the underlying molecular mecha- nisms for the adaptations that occur in response to chronic physical training, and the implications of these studies for people with diabetes. EXERCISE INCREASES MUSCLE GLUCOSE UPTAKE AND INSULIN SENSITIVITY In the exercising muscle, the increased need for metabolic fuel is met partially through an increase in the uptake and utilization of glucose. The first evidence for this phenomenon was provided over a century ago, when chewing by horse masseter muscle was shown to decrease the glucose concentration in the ve- nous outflow from the muscle (11). In the 1950s, studies of rats (12) and per- fused dog hindlimbs (13) confirmed this finding, and in the 1960s glucose up- take kinetics were first described using incubated frog sartorius muscles con- tracted in vitro (14, 15). Since these early studies, a considerable amount of work has characterized the effects of exercise on glucose uptake in skeletal muscle, and a few recent reviews have focused on this topic (16–18). In addi- tion to the acute effects of exercise to increase muscle glucose uptake, the peri- od after exercise is characterized by the muscle being more sensitive to the ac- tions of insulin. This was first demonstrated in perfused rat hindlimb muscles (19, 20) and was subsequently shown in studies of human subjects (3, 4, 6, 7). One-legged exercise models in humans have demonstrated that the exercise- induced increase in insulin sensitivity for glucose uptake is a local phenome- non restricted to the exercised muscles (4, 21). EXERCISE AND GLUCOSE TRANSPORT 237 MECHANISMS FOR THE INCREASES IN GLUCOSE UPTAKE AND INSULIN SENSITIVITY WITH ACUTE EXERCISE Numerous factors determine the rate of glucose uptake during and after exer- cise. During exercise, one of the most important regulatory responses is an in- crease in blood flow to the contracting skeletal muscles (reviewed in 16). The increase in blood flow provides ample substrate to the working muscles, and thus, glucose availability is usually not the rate-limiting factor for glucose utilization when exercise is performed under normal physiological conditions. Instead, glucose transport is thought to be the rate-limiting step in glucose Annu. Rev. Med. 1998.49:235-261. Downloaded from www.annualreviews.org by Texas A&M University - Commerce on 07/19/13. For personal use only. utilization during exercise. Over the past several years considerable progress has been made in understanding the molecular basis for the effects of exercise to increase glucose transport in skeletal muscle. The Glucose Transport System Glucose transport in skeletal muscle occurs primarily by facilitated diffusion, utilizing glucose transporter carrier proteins. In mammalian tissues, glucose transporters are a family of structurally related proteins that are expressed in a tissue-specific manner (22). GLUT4 is the major isoform present in mouse, rat, and human skeletal muscle, whereas the GLUT1 and GLUT5 isoforms are expressed at a much lower abundance (23, 24). GLUT5 is a high-affinity fruc- tose transporter with a much lower capacity to transport glucose (25). Exercise and insulin are major mediators of glucose transport activity in muscle, which occurs through an increase in the maximal velocity of transport (Vmax) without an appreciable change in the substrate concentration at which glucose trans- port is half maximal (K1/2)(14, 26–28). Glucose Transporter Translocation There is substantial evidence that a major mechanism by which exercise in- creases glucose uptake in skeletal muscle is through the translocation of glu- cose transporter proteins from an intracellular compartment to the surface of the cell (29–38). In addition, sciatic nerve stimulation resulting in contraction of hindlimb skeletal muscles in situ also increases the plasma membrane glu- cose transporter number in the rat (39–42). The exercise-induced translocation of glucose transporters is due to an increase in the plasma membrane content of the GLUT4 isoform, since a single bout of exercise does not alter the abun- dance of plasma membrane GLUT1 (33, 35, 36) or GLUT5 (EA Richter & HS Hundal, personal communication). A recent study has demonstrated that a sig- nificant percentage of the GLUT4 that is translocated to the plasma membrane fraction with exercise associates with nonjunctional transverse tubules (38). 238 GOODYEAR & KAHN Although most of the work done in this area comes from studies using subcel- lular fractionation of skeletal muscle, more recent studies using immunocyto- chemical analysis of skeletal muscle sections by electron microscopy (43), or labeling of cell surface GLUT4 protein using a membrane-impermeable bis- mannose photolabel (44, 45), have confirmed that muscle contractions in- crease plasma membrane GLUT4 protein. Similar to the effects of exercise, insulin also causes GLUT4 translocation in skeletal muscle (33, 36, 39, 46). Since blood flow is increased during exer- cise, it is conceivable that the exercise-induced recruitment of GLUT4 to the plasma membrane is due to increased delivery of insulin to the working mus- Annu. Rev. Med. 1998.49:235-261. Downloaded from www.annualreviews.org cles. However, when hindlimb skeletal muscles are contracted in situ in the ab- by Texas A&M University - Commerce on 07/19/13. For personal use only. sence of insulin, plasma membrane GLUT4 is increased to a similar degree to that which occurs with exercise in vivo (39–42). These findings demonstrate that contraction can recruit GLUT4 to the plasma membrane in rat skeletal muscle independent of insulin, and they provide a mechanism for earlier re- ports showing that insulin is not required for muscle contraction to increase glucose uptake in skeletal muscle (47–49). The combination of exercise and insulin can have additive or partially addi- tive effects on glucose transport (27, 50–52), which may be associated with an additive effect on GLUT4 recruitment to the plasma membrane (42, 45). These findings support the hypothesis that different mechanisms lead to the stimula- tion of muscle glucose transport by exercise and insulin. In fact, there is evi- dence that there are two distinct intracellular locations or pools of glucose transporters in skeletal muscle, one that responds to exercise and one that re- sponds to insulin (30, 33, 34). In these reports, insulin, but not exercise, was shown to decrease glucose transporters from an intracellular microsomal membrane fraction. Recently, modification of one of these fractionation pro- cedures resulted in the isolation of a novel intracellular membrane fraction that is sensitive to exercise (38, 53), giving further support to the hypothesis that there are separate pools of glucose transporters in skeletal muscle. While there are different sedimentation coefficients for the insulin- and exercise-sensitive fractions, there appears to be little difference in the major protein composition of these fractions (53). The exact intracellular locations of the putative exer- cise- and insulin-stimulated GLUT4 pools have not yet been elucidated. The majority of intracellular GLUT4 is located in small tubulo-vesicular organelles (43, 46), and it is still not clear if these vesicles are unique intracel- lular compartments or ubiquitously expressed organelles that are enriched in GLUT4. As has recently been reviewed in detail (54), studies of adipose cells suggest that there is a low rate of continuous recycling of GLUT4 in the basal state, and that insulin acts primarily through increasing transporter exocytosis. In skeletal muscle, it is not known if the exercise-induced recruitment of EXERCISE AND GLUCOSE TRANSPORT 239 GLUT4 occurs through the regulation of vesicular exocytosis. However, sev- eral proteins that are involved in regulated endocytosis or exocytosis in other tissues have also been identified as components of GLUT4-containing vesicles in skeletal muscle (37, 53, 55–57). Of these proteins, the aminopeptidase gp160/vp165 (53) and vesicle-associated membrane protein-2 (VAMP-2) (55) translocate to the plasma membrane in response to physical exercise in skeletal muscle, similar to the effects of insulin. In contrast, insulin, but not exercise, results in the redistribution of Rab4 (37), a small GTP-binding protein that has also been implicated in the regulation of GLUT4 translocation in adipose cells (58, 59). The studies of Rab4 suggest that there may be distinct exercise- and insulin-stimulated GLUT4-containing vesicles that utilize different molecular Annu. Rev. Med. 1998.49:235-261. Downloaded from www.annualreviews.org by Texas A&M University - Commerce on 07/19/13. For personal use only. switches for mobilization. Intracellular Signaling Mechanisms The molecular signaling pathways that lead to the stimulation of glucose uptake in skeletal muscle or other cell types have not been completely elucidated. Un- til recently, it was not known whether the analogous effects of insulin and ex- ercise on skeletal muscle glucose uptake occur via similar or different molecu- lar signals. For insulin action, the cascade of signaling events is initiated by in- sulin binding to the extracellular α-subunit of the insulin receptor, autophos- phorylation of tyrosine residues in the receptor β-subunit, tyrosine phosphory- lation of the insulin receptor substrates IRS-1 and IRS-2, and activation of phosphatidylinositol 3-kinase (PI 3-kinase) (reviewed in 60, 61). As for exer- cise, several studies have clearly demonstrated that these proximal insulin- signaling steps are not components of the signaling mechanism by which exer- cise stimulates glucose uptake, since contractile activity does not stimulate autophosphorylation of isolated insulin receptors (62), receptor and IRS tyro- sine phosphorylation (63, 64), or PI 3-kinase activity (63, 64). Furthermore, wortmannin, a PI 3-kinase inhibitor, does not inhibit contraction-stimulated glucose transport in vitro (45, 65, 66). These signaling studies demonstrate that the underlying molecular mechanisms leading to the insulin- and exercise- induced stimulation of glucose uptake in skeletal muscle are distinct. In addi- tion to these studies of muscle signaling intermediaries, we recently demon- strated that the mitogen-activated protein kinase signaling cascade, which is also increased in response to exercise (67, 68), is not involved in stimulating glucose uptake with exercise (69). These findings have important implications for determining the mechanisms by which insulin and exercise regulate skele- tal muscle glucose uptake in human subjects. More importantly, in diabetic in- dividuals who are insulin resistant, it is likely that exercise can function to acti- vate alternative mechanisms to improve skeletal muscle glucose uptake. 240 GOODYEAR & KAHN There are several lines of evidence to suggest that the increase in intracel- lular calcium that leads to the interaction of actin and myosin filaments with muscle contraction is a critical mediator of contraction-stimulated glu- cose transport (70). For example, the incremental increase in contraction- stimulated transport correlates with the frequency of contraction, not the amount of work or tension developed (14). In addition, caffeine, an agent that causes contraction by increasing calcium release from the sarcoplasmic re- ticulum in the absence of membrane depolarization, increases glucose transport (15). Since cytoplasmic calcium concentrations are elevated for only a fraction of a second following each muscle contraction, these molecules probably do not directly activate the glucose transport system. Instead, the Annu. Rev. Med. 1998.49:235-261. Downloaded from www.annualreviews.org by Texas A&M University - Commerce on 07/19/13. For personal use only. rise in cytosolic calcium may initiate or facilitate the activation of intracellu- lar signaling molecules or cascades of signaling proteins that lead to both the immediate and prolonged effects of exercise on muscle glucose transport. Protein kinase C (PKC) is an example of a calcium-dependent signaling in- termediary that has been shown to be activated by muscle contraction (71, 72) and may be involved in the regulation of contraction-stimulated glucose transport. Down-regulation of PKC by long-term phorbol ester treatment (72) and inhibition of PKC using polymyxin B (73, 74) have both been associated with decreases in contraction-stimulated glucose transport. There is also evi- dence for an autocrine or paracrine component for the activation of con- traction-stimulated glucose uptake, one important example being nitric ox- ide. Nitric oxide is released from skeletal muscle contracted in vitro, and in- hibition of nitric oxide synthase has been demonstrated to decrease both ba- sal (75) and contraction-stimulated (76) glucose transport, an effect that may be regulated by a cGMP-mediated mechanism (77, 78). Another molecule that may be involved with contraction-stimulated glucose transport is kallik- rein, which catalyzes the production of bradykinin and is a potential stimu- lator of nitric oxide synthase. Adenosine has also been shown to be secreted from contracting muscle fibers (79), and it has been suggested that the adenosine receptor mediates the signaling mechanism through which con- traction results in the synergistic stimulation of glucose transport (80). In addition to the activation of specific intracellular signaling molecules, the glycogenolytic process may be an important regulator of exercise-induced GLUT4 translocation in skeletal muscle. Although there is still no direct evi- dence, it has long been hypothesized that transporter molecules are associ- ated with glycogen particles in the muscle, and that the contraction- stimulated hydrolysis of glycogen releases GLUT4, leading to translocation of these transporters to the cell surface. The effects of exercise and insulin on GLUT4 translocation in skeletal muscle are illustrated schematically in Figure 1. Annu. Rev. Med. 1998.49:235-261. Downloaded from www.annualreviews.org by Texas A&M University - Commerce on 07/19/13. For personal use only. Figure 1 GLUT4 translocation in skeletal muscle. Muscle contractions and insulin cause the translocation of the GLUT4 glucose transporter pro- teins to the plasma membrane and transverse tubules. GLUT1 and GLUT5 are present in the plasma membrane. The subcellular origin of the GLUT4- containing vesicles is not clear, but exercise and insulin appear to recruit distinct GLUT4-containing vesicles, and/or mobilize different pools of GLUT4 proteins. Insulin-stimulated GLUT4 translocation involves IRS-1 and PI 3-kinase, and the redistribution of Rab4. Contraction utilizes a PI 3- EXERCISE AND GLUCOSE TRANSPORT kinase and MAP kinase-independent mechanism and does not result in the redistribution of Rab4. The contraction signal is probably initiated by the release of calcium from the sarcoplasmic reticulum and may involve an autocrine/paracrine mechanism (e.g. nitric oxide, adenosine, bradykinin), 241 protein kinase C (not shown), or a combination of these and other currently unknown factors. NO, Nitric oxide; PI, phosphatidylinositol. 242 GOODYEAR & KAHN Mechanisms for the Post-Exercise Increases in Insulin Sensitivity The period following exercise is typically characterized by elevated rates of basal and insulin-stimulated glucose uptake. In fact, the increase in insulin- stimulated glucose uptake that occurs in response to both exercise in vivo and electrical stimulation to produce muscle contractions in situ can persist for several hours following the cessation of exercise (19, 20, 50, 81–83). The mo- lecular basis for this phenomenon has not been completely elucidated but ap- pears to be dependent on multiple factors, including muscle glycogen concen- Annu. Rev. Med. 1998.49:235-261. Downloaded from www.annualreviews.org trations, humoral factors, and autocrine/paracrine mechanisms. by Texas A&M University - Commerce on 07/19/13. For personal use only. The degree of glycogen depletion resulting from the antecedent exercise session is clearly an important factor in determining the rate and duration of the increase in muscle glucose uptake after exercise. The reversal of the in- crease in glucose uptake and insulin sensitivity can be manipulated by muscle glycogen concentrations, as carbohydrate feeding that results in increased gly- cogen concentrations accelerates the return of basal rates of glucose uptake, and carbohydrate restriction that maintains glycogen depletion slows this re- versal process (82, 84). However, the level of glycogen is clearly not the only regulator of glucose uptake post-exercise. Studies in isolated epitrochlearis muscles (51, 83, 85) and individual tissues from perfused hindquarter muscle (86) have shown that the reversal of glucose transport following contraction is not always dependent on glycogen synthesis. In these experiments, glycogen concentrations were maintained at a low level (depleted); however, in all stud- ies, 3-O-methylglucose transport returned to baseline or near baseline values. The cellular mechanism leading to the increase in insulin sensitivity after exercise has been hypothesized to involve enhanced insulin signaling. How- ever, exercise does not change insulin binding to its receptor (62, 87, 88), and prior exercise does not increase insulin-stimulated receptor tyrosine kinase ac- tivity in skeletal muscles obtained from rats (62) or humans (JFP Wojtasewski & E Richter, unpublished observation). Furthermore, prior contraction of rat hindlimb skeletal muscles has been shown to actually cause a paradoxical de- crease in insulin-stimulated tyrosine phosphorylation of IRS1 and IRS1- associated PI 3-kinase activity (67). Consistent with these finding are recent studies showing that insulin’s ability to activate IRS1-associated PI 3-kinase activity in vivo is diminished in previously exercised human muscle (JFP Wojtasewski & E Richter, unpublished observation). These studies rule out a role for enhanced insulin signaling as a mechanism for increased glucose up- take after exercise and provide additional support to the hypothesis that exer- cise and insulin act through distinct signaling mechanisms. The lack of en- hancement of these steps in the insulin signaling cascade may not be surpris- EXERCISE AND GLUCOSE TRANSPORT 243 ing, since increased sensitivity following exercise is not limited to insulin. The effect of hypoxia on glucose transport is also markedly amplified in muscles studied 3 h after exercise (89), and hypoxia has also been shown to use a sig- naling pathway that bypasses the insulin receptor, IRS-1 and IRS-2, and the activation of PI 3-kinase (90). Some studies suggest that the persistent increase in glucose uptake post- exercise requires the presence of insulin (51, 83). In isolated epitrochlearis muscles in vitro, there was a persistent increase in glucose uptake only in mus- cles that were incubated with insulin following exercise (51, 83). These inves- tigators hypothesized that exercise causes a recruitment of glucose transport- Annu. Rev. Med. 1998.49:235-261. Downloaded from www.annualreviews.org ers to the plasma membrane and that the presence of insulin will slow internali- by Texas A&M University - Commerce on 07/19/13. For personal use only. zation of glucose transporters to the intracellular pool, thus keeping transport elevated. The finding that rates of glucose uptake in hindquarter skeletal mus- cle are elevated for several hours after exercise in the presence but not the ab- sence of insulin supports this hypothesis (50). More recently it was shown that when intact muscles are isolated, washed, and contracted in vitro, there is no increase in insulin sensitivity after contractions (89). This work, and a subse- quent report, suggest that a serum factor, probably a protein, is required for the effects of contraction to enhance insulin sensitivity (91). In contrast to these findings are the results from another study indicating that adenosine receptors may mediate the ability of muscle contraction to increase insulin-stimulated glucose uptake (80). Taken together, these studies suggest that there may be no single factor that regulates the enhanced muscle insulin sensitivity for glucose uptake in the post-exercise state. Instead, this physiological phenomenon may be regulated by a combination of serum factors, autocrine/paracrine mecha- nisms, and muscle glycogen concentrations. EXERCISE AND GLUCOSE TRANSPORT IN INSULIN- RESISTANT STATES The effects of exercise on glucose uptake have been studied in a number of ro- dent and human models of insulin resistance. Whereas in most insulin-resistant states, such as obesity and NIDDM, GLUT4 gene expression is reduced in adi- pose cells, in skeletal muscle GLUT4 expression is normal (92–94). Thus, the decreased glucose uptake in response to insulin (insulin resistance) in skeletal muscle results from alterations in the translocation, docking, or fusion of glucose transporters at the plasma membrane or T tubules, or potentially from changes in the specific activity of the transporters (moles of glucose transported/trans- porter/unit of time). Such defects are thought to result from impaired intracel- lular signaling, and in some states this may involve defective activation of PI 3-kinase, which results, at least in part, from decreased expression of the p85 Annu. Rev. Med. 1998.49:235-261. Downloaded from www.annualreviews.org by Texas A&M University - Commerce on 07/19/13. For personal use only. 244 GOODYEAR & KAHN EXERCISE AND GLUCOSE TRANSPORT 245 regulatory subunit of PI 3-kinase. Insulin-stimulated activation of PI 3-kinase is impaired in skeletal muscle in rodent models of genetic obesity and hyper- insulinemic diabetes (95, 96), in rats rendered insulin resistant with high-fat feeding (97) or glucocorticoid treatment (98), and in humans with obesity (99) and NIDDM (100). However, as described above, the activation of PI 3-kinase is not important for the effects of exercise to stimulate glucose transport. In many insulin-resistant states, the stimulation of glucose transport and GLUT4 translocation in response to exercise is normal. For example, in skele- tal muscle from the genetically obese Zucker rats, insulin-stimulated glucose transport is markedly decreased in spite of normal GLUT4 expression (101, 102). Studies performing subcellular fractionation of muscle followed by Annu. Rev. Med. 1998.49:235-261. Downloaded from www.annualreviews.org by Texas A&M University - Commerce on 07/19/13. For personal use only. immunoblotting for GLUT4 indicate that the defect is in the insulin-stimulated translocation/fusion of GLUT4 with the plasma membrane (103). In contrast, exercise (104, 105) and contraction (106) increase glucose uptake and GLUT4 translocation normally in muscle from these same rats (Figure 2). Other states of insulin resistance such as short periods of high-fat feeding or immobiliza- tion are also associated with insulin resistance in skeletal muscle in spite of normal GLUT4 expression (107, 108). The glucose transport response to exer- cise is normal in these states also (107, 108). With longer periods of high-fat feeding (109) or immobilization (110, 111), GLUT4 expression in muscle is reduced and once GLUT4 is down-regulated, exercise-stimulated glucose transport becomes impaired. In humans with obesity or NIDDM, defects in insulin signaling are thought to be involved in the etiology of insulin resistance. Muscles from these sub- jects increase glucose transport normally in response to a variety of stimuli that act downstream of the insulin receptor, such as hypoxia, okadaic acid, vana- date, and phenylarsine oxide (90). These results suggest that the GLUT4 trans- location/ fusion machinery is operational but that defects specifically in the in- sulin signaling pathway cause resistance to insulin. The effects of contraction or exercise on glucose transporter translocation in muscles from NIDDM sub- jects have not been studied. However, in vivo studies in obese, NIDDM sub- jects show a normal increase in whole-body glucose utilization during moder- ate exercise (112). In nonobese NIDDM subjects, exercise-induced leg glu- cose uptake was actually increased compared with nondiabetic control sub- Figure 2 Effects of insulin and exercise on plasma membrane glucose transporters in lean and obese Zucker rats. (Top) Plasma membranes were partially purified from hindlimb skeletal mus- cle, and glucose transporter number (R0) was determined by Scatchard analysis derived from D- glucose inhibitable cytochalasin B binding. Values are means ± SE; n = 5–10/group. (Adapted from References 103, 104.) (Bottom) Schematic illustration showing that exercise, but not insu- lin, is effective in causing glucose transporter translocation in the obese Zucker rat. 246 GOODYEAR & KAHN jects (113). It was postulated that the latter finding resulted from a hyperglycemia-induced increase in the mass action of glucose, since the glu- cose clearance rate that takes into account the ambient glucose concentration was comparable in the two groups. The lack of impairment in the response of subjects with obesity and diabetes to exercise in spite of marked insulin resis- tance underscores the fact that exercise and insulin utilize different signaling pathways to stimulate glucose uptake. These sorts of observations underlie the utility of exercise in the prevention and treatment of NIDDM. A better under- standing of the signaling pathway(s) utilized by exercise could lead to new therapeutic approaches to prevent or treat NIDDM. Annu. Rev. Med. 1998.49:235-261. Downloaded from www.annualreviews.org by Texas A&M University - Commerce on 07/19/13. For personal use only. EPIDEMIOLOGIC STUDIES INDICATING BENEFICIAL EFFECTS OF EXERCISE IN THE PREVENTION OF NIDDM Exercise training, defined as repeated bouts of exercise, results in multiple physical and metabolic changes, including a significant increase in aerobic ca- pacity. The metabolic changes have important implications for individuals who have impaired glucose tolerance (IGT), gestational diabetes, and NIDDM. Epidemiological studies show that physical inactivity may be a risk factor for NIDDM, at least in some ethnic groups, especially among Mexican Americans (reviewed in 114). In persons without diabetes, glucose and insulin levels after a meal are significantly higher in less-active compared with more- active persons (114). Although cross-sectional studies do not find a consistent association between physical activity and NIDDM in all populations, such an association has been shown in both case-control and cohort studies (114) (Table 1). One population-based study of women aged 55–69 showed that high levels of physical activity reduced the risk of developing NIDDM by 50% compared with age-matched women with low levels of physical activity (115). Prospective cohort studies of male college alumni (10), female nurses (8), and male physicians (9) demonstrate a reduced risk of developing NIDDM with increased physical activity. This is particularly evident among high-risk persons, defined as those with a high body mass index, high blood pressure, or a parent with NIDDM (10). In one study, each 500 kcal of additional leisure- time physical activity per week was associated with a 6% decrease in the risk of developing NIDDM. Subjects participating in these prospective studies ranged from 34–84 years old (see Table 1). In addition, in feasibility studies in Sweden and China, physical activity has been instituted as part of an interven- tion to prevent the development of diabetes among persons with impaired glu- EXERCISE AND GLUCOSE TRANSPORT 247 Table 1 Epidemiological studies demonstrating the efficacy of physical activity in the prevention of NIDDMa Study population Main findings Dose response Reference Case control study Women, aged 55-69 High levels of physical activity de- Yes 115 creased risk of NIDDM by 50%. Cohort studies Male college alums, Physical activity level was inversely Yes 10 aged