Genetics Notes Exam 4 PDF
Document Details
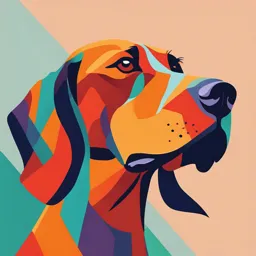
Uploaded by DurableMridangam5951
Tags
Summary
The document contains lecture notes on epigenetics and its related concepts, covering topics like the definition of epigenetics, epigenetic problems, phenomena, and mechanisms. It includes various examples and highlights the roles of different epigenetic mechanisms.
Full Transcript
GENETICS NOTES EXAM 4 Lecture 11 – Chapter 12 DEFINITION OF EPIGENTICS – - Epigenetics covers all phenomena that, without affecting the DNA sequence of interest itself, can somehow produce heritable changes – on the level of how genes are expressed and how chromosome functions wo...
GENETICS NOTES EXAM 4 Lecture 11 – Chapter 12 DEFINITION OF EPIGENTICS – - Epigenetics covers all phenomena that, without affecting the DNA sequence of interest itself, can somehow produce heritable changes – on the level of how genes are expressed and how chromosome functions work. EPIGENTIC PROBLEMS – - How come keratinocytes only form skin cells? - How do different adult stem cells know their fate? - How can identical twins have different natural hair colors? - How can a single individual have two different eye colors? - How can identical twin liter mates show different coat colors? - How can only paternal or maternal traits be expressed in offspring? - How can females express only one x chromosome per cell? - How can acquired traits be passed on to offspring? Phenomenon Mechanism/Comments Epigenetic reprogramming in the early Eggs and sperm are differentiation cells, and embryo their genomes have different epigenetic marks. They combine to give a zygotes whose genome is gradually reprogrammed to erase the great majority of the inherited epigenetic marks. By the blastocyst stage, the cells of the inner cell mass and pluripotent and will give rise ultimately to all cells of the body. Epigenetic marks and reestablished in the descendants of the cells of the inner cell mass to establish different cell lineages and permit cell differentiation. Epigenetic reprogramming on gametogenesis Readily detected as a wave of genome wide demethylation during germ cell development(erasing parental epigenetic marks) followed by comprehensive de novo DNA methylation to reset global patterns of DNA methylation and gene expression Establishment of constitutive heterochromatin Centromere establishment relies on a specific histone H3 variant known as CENP-A X-chromosome inactivation Silencing by a long noncoding RNA (XIST RNA) of almost all genes on one of the two X chromosomes in female cells Genomic Imprinting Silencing of one allele, according to parent of origin, at diverse gene loci (often organized in gene clusters) on different chromosomes Position effects causing Large-scale changes in DNA. Causing to be heterochromatinization relocated to a heterochromatin environment where they are silenced EPIGENETICS OF toti-, pluri-, and unipotency – - Totipotency – ability of a single cell to divide and produce all of the differentiated cells in an organism. Example totipotent cells are zygotes. - Pluripotency – refers to a stem cell that has the potential to differentiate into and out of the three germ layers: endoderm (interior stomach lignin, gastrointestinal tract, the lungs), mesoderm (muscle, bone, blood urogenital), or ectoderm (epidermal tissues and nervous system). - Unipotency – the concept that one stem cell has the capacity to differentiate into only one cell type. It is currently unclear if true unipotent stem cells exist. Ex. Hepatoblasts, which differentiate into hepatocytes. PLURIPOTENCY VS UNIPOTENCY – - What is the difference? The ability to become any type of cell in the body is called pluripotent. The difference between totipotent to pluripotent cells is only that totipotent cells can give rise to both placenta and embryo. As the embryo grows these pluripotent cells develop into specialized, multipotent stem cells. CAN BE REVERSED – CHROMATIN STRUCTURE AND GENE EXPRESSION – - Open to condensed = DNA methylation histone DEacetylation. - Condensed to open = DNA DEmethylation histone acetylation. DNA METHYLATION AND HISTONE MODIFICATIONS: EPIGENETIC CODE – - DNA methylation – Methyl marks added to certain DNA bases repress gene activity. - Histone modification – a combination of different molecules can attach to the tails of proteins called histones. These alter the activity of the DNA wrapped around them. DNA METHYLATION AND HISTONE MODIFICATIONS HELP TO COMPARTMENTALIZE THE GENOME INTO DOMAINS OF DIFFERENT TRANCRIPTIONAL POTENTIALS – - Euchromatin o High histone acetylation o Low DNA methylation o H3-K4 methylation - Heterochromatin o Low histone acetylation o Dense DNA methylation o H3-K9 methylation ORGANIZATION OF THE ‘EPIGENOME’ – COORDINATION OF DNA METHYLATION AND HISTONE MODIFICATION – CHROMOSOME MODIFIERS: WRITERS, ERASERS AND READERS – MODIFICATION OF HISTONES IN NUCLEOSOMES – - Acetylation – only at lysine, phosphorylation mostly as serine but both lysine and arginine can be methylated. - Acetylation of lysine – loss of positive charge, interacts less with the next nucleosome. - Methylation preserves charge, can increase or decrease nucleosome binding. DIFFERENT HISTONE VARIANTS – MEHTYLATION OF CYTOSINE IN DNA – 5-METHYL CYTOSINE IN DNA – CYTOSINE METHYLATION MAINTAINS INACTIVE-CONDENSED CHROMATIN STATE PRESERVATION OF DNA METHYLATION: MECHANISM – MAMMALIAN DNMTS ARE ESSENTIAL – Dnmt 1: embryonic lethal Dnmt 2: no obvious effect Dnmt 3a: perinatal death Dnmt 3b: embryonic lethal Dnmt 3l: no imprints CYTOSINE METHYLATION IN MAMMALS – - Gene expression - Chromosomal stability - Cell differentiation - Imprinting - X-inactivation - Carcinogens - Aging CRITICAL CPG SEQUENCES IN CPG ISLANDS NEAR PROMOTERS – WHY C TO T MUTATIONS ARE SO COMMON – MUTATION HOT SPOT: CG SEQUENCE – - The best known example is the two-base (dinucleotide) sequences CG. In mammals, about 80% of CG dinucleotides are methylated: A methyl group is attached to the cytosine base. A methylated cytosine, 5-methycytosine, easily loses an amino group, converting it to thymine. The end result is a mutation from cytosine to thymine. - Surveys of mutations in human genetic diseases have shown that the mutation rate at CG dinucleotides is about 12 times higher than at other dinucleotides sequences. DNA METHYLATION IN EARLY DEVELOPMENT AND GAMETOGENESIS – EX OF EPIGENTICS INHERITANCE – 1. SPREADING AND EPIGENETIC INHERITANCE OF HETEROCHROMATIN - Chromatin remodeling exposes regulatory sequences. o Chromatin remodeling is the dynamic modification of chromatin architecture to allow access of condensed genomic DNA to the regulatory transcription machinery protein, and thereby control gene expression. - Boundaries of heterochromatin must be maintained o This gene called “white: gives flies their red eye color. It is near the centromere and heterochromatin. - A chromosomal re-arrangement that moves white near heterochromatin silences the gene in patches 2. GENOMIC IMPRINTING - Genomic Imprinting o Mendel’s experimental work with garden peas established that the phenotype is the same whether a given allele is inherited from the mother or the father. Indeed, this principle has long been part of the central dogma of genetics. o Recently however, it has become increasingly apparent that some human genes, one of the alleles is transcriptionally inactive (no mRNA is produced), depending on the parent from whom the allele was received. o For example, an allele transmitted by the mother would be inactive, and the same allele transmitted by the father would be active. Ther normal individual would have only one transcriptionally active copy of the gene. This process of gene silencing is known as imprinting, and the transcriptionally silences genes are said to be imprinted. o At least several dozen human genes, and perhaps as many as 200 or so, are known to be imprinted. o Imprinted alleles tend to be heavily methylated 9in contrast to the nonimprinted copy of the allele, which typically is not methylated). The attachment of methyl groups to 5’ regions of genes, along with histone hypoacetylation and condensation of chromatin, inhibits the binding of proteins that promote transcription. It should be apparent that this process is similar in many ways to X-inactivation, discussed earlier in this chapter. o DEFINITION of Genomic Imprinting – o For some genes, although we have two copies, one on each chromosome, only one copy is active or expressed. o Expression of these genes is variable overall but specific for each gene depending on which parent the gene came from. - Established and candidate imprinted gene distribution through the human genome - Significance of genomic imprinting – proof o Uniparental diploidy – lethal! - Imprinting: How is expression controlled? o Imprinting occurs by a pattern of methylation The copy of the gene to be inactivated is coated with methyl groups. Methylation prevents that gene from being expressed. This takes place before fertilization, in the egg and sperm cells and is orchestrated by an imprinting center. o Genomic imprinting is a reversible form of gene inactivation but remains consistent/stable through mitosis. - Epigenetic imprinting o Genomic imprinting – the unequal expression of the maternal and maternal alleles of a gene. o Imprinted or marked with their gametic (parental) origin. - Methylation in early development and gametogenesis - Methylation changes during development o Each chromosome in the egg now has a maternal imprint, each in the sperm a paternal imprint. o For most genes, we inherit two working copies – one from mom and one from dad. But with imprinted genes, we inherit only one working copy. Depending on the gene, either the copy from mom or the copy from dad is epigenetically silenced. o The epigenetics tags on imprinted genes, usually stay put for the life of the organism. But they are RESET during egg and sperm formation. Regardless of whether they came from mom or dad, certain genes are always silenced in the egg, and others are always silenced in the sperm. - Establishing sex-specific imprints - Imprinting o Overall result in imprinting: Allows a gene to be expressed from one allele, the other is methylated and therefore inactive Expression is parent specific o Mechanisms of disease in imprinting disorders include: Deletion Imprinting defect Uniparental disomy Gene mutation o All mechanisms result in lack of expression of a gene that should be expressed. - Disorders of imprinting: uniparental disomy - Uniparental disomy o A unique feature to imprinted conditions is uniparental disomy (UPD): when a child inherits both copies of a chromosome from one parent. Is clinically significant if it involves genes that are imprinting Increases the risk of autosomal recessive disorders if UPD involves the gene affected o Isodisomy Inheritance of two copies of the same homolog from one parent o Heterodisomy Inheritance of two different homologs from one parent - Prader-Willi and Angelman Syndromes o Deletion of about 4 Mb of the lang arm of chromosome 15. When this deletion is inherited from the father, the child manifests a disease known as Prader-Willi syndrome (PWS) The features of PWS include short stature, hypotonia, small hands and feet, obesity, mild to moderate mental retardation, and hypogonadism. o When the same deletion is inherited from mother, the child develops Angelman syndrome, which is characterized by severe mental retardation, seizures, and an ataxic gait. o The deletion that cause PWS and Angelman syndrome are microscopically indistinguishable and affect the same group of genes. - Genomic imprinting o Prader-Willi and Angelman Syndromes Analysis showed that the 4 Mb deletions (the critical region) contains several genes that normally are transcribed only on the chromosome inherited from father These genes are transcriptionally inactive (imprinted) on the copy of chromosome 15 inherited from the mother. Similarly, other genes in the critical region are active only on the chromosome inherited from the mother and are inactive on the chromosome inherited from the father. Thus, several genes in this region are normally active on only one chromosome. If the single active copy of one of these genes is lost through a chromosome deletion, then no gene product is produced at all, and the disease results. 3. X-CHROMOSOME INACTIVATION X inactivation - In the 1960s, Mary Lyon hypothesized that female is inactivated one X chromosome in each somatic cell of the. This would result in dosage compensation, an equalization of the amount of X-linked gene products in males and females. The Lyon hypothesis stated that X inactivation occurs early in female embryonic development and that the X chromosome contributed by the father is inactivated in some cells, whereas in other cells the X chromosome contributed by the mother is inactivated. In each cell, one of the two X chromosomes is chosen at random for inactivation, so the maternally and paternally derived X chromosomes are each inactivated in about half of the embryo's cells. Once an X chromosome is inactivated in a cell, it will remain inactive in all descendants of that cell. X inactivation is therefore a randomly determined, but fixed (or permanent), process. Because they have two populations of cells, activity. females are mosaics for X chromosome Males, having only one copy of the X chromosome, are not mosaics but are hemizygous for the X chromosome ( hemi means "half"). The inactivation process takes place within approximately 7 to 10 days after fertilization, when the embryonic inner-cell mass contains no more than a few dozen cells. Inactivation is initiated in a single 1-Mb region on the X chromosome long arm, the X inactivation center , and then spreads along the chromosome. X inactivation is permanent for all somatic cells in the female, but the inactive X chromosome must later become reactivated in the female's germline so that each of her egg cells will receive one active copy of the X chromosome. The number of Barr bodies in somatic cells is always one less than the number of X chromosomes. Normal females have one Barr body in each somatic cell, and normal males have none. Females with Turner syndrome, having only one X chromosome, have no Barr bodies. Females who have three X chromosomes per cell have two Barr bodies in each somatic cell. Is incomplete – Why aren't people with extra (or missing) X chromosomes phenotypically normal? X inactivation is all copies. incomplete. Some regions of the X chromosome remain active in For example, the tips of the short and long arms of the X chromosome do not undergo inactivation. The tip of the short arm of the X chromosome is homologous to the distal short arm of the Y chromosome. In total, about 15% of the genes on the X chromosome escape inactivation, and relatively more genes on the short arm escape inactivation than on the long arm. Some of the X-linked genes that remain active on both copies of the X chromosome have homologs on the Y chromosome, preserving equal gene dosage in males and females. Thus, having extra (or missing) copies of active portions of the X chromosome contributes to phenotypic abnormality. XIC region Characteristic of XIST How it silences the future inactive x Selection of one active x chromosome It is hypothesized that there is an autosomally-encoded 'blocking factor' which binds to the X chromosome and prevents its inactivation. The model postulates that there is a limiting blocking factor, so once the available blocking factor molecule binds to one X chromosome the remaining X chromosome(s) are not protected from inactivation. This model is supported by the existence of a single Xa in cells with many X chromosomes and by the existence of two active X chromosomes in cell lines with twice the normal number of autosomes.