Cardiovascular Function Study Guide
Document Details
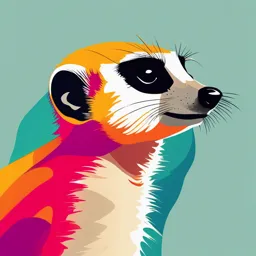
Uploaded by LovelyCarnation6385
Andrews University
Tags
Summary
This is a study guide covering cardiovascular function, including the cardiac cycle, cardiac action potential, heart structures and the physiology behind them. Helpful keywords in the summary include heart anatomy and cardiac cycle. The document has a focus on medical and biological sciences.
Full Transcript
Exam Objectives Cardiovascular Function I & II (19 MCQ) 1. Relate the structures of the heart to the phases of the cardiac cycle The heart\'s structure is intricately related to its function, particularly in the context of the cardiac cycle, which comprises several phases critical to the hear...
Exam Objectives Cardiovascular Function I & II (19 MCQ) 1. Relate the structures of the heart to the phases of the cardiac cycle The heart\'s structure is intricately related to its function, particularly in the context of the cardiac cycle, which comprises several phases critical to the heart\'s pumping action. Here\'s a detailed explanation of how the heart\'s structures relate to each phase of the cardiac cycle: **Cardiac Cycle Overview** The cardiac cycle includes all the events that occur in a single heartbeat, encompassing both diastole (when the heart relaxes) and systole (when the heart contracts). It can be divided into several phases: 1. **Atrial Systole** 2. **Isovolumetric Contraction** 3. **Ventricular Ejection** 4. **Isovolumetric Relaxation** 5. **Ventricular Filling** **Structures and Phases of the Cardiac Cycle** **1. Atrial Systole** - **Structures Involved**: Atria (left and right), atrioventricular (AV) valves (mitral and tricuspid). - **Phases Details**: - The atria contract, pushing blood into the ventricles through the open AV valves. - The right atrium pumps blood into the right ventricle, and the left atrium pumps blood into the left ventricle. **2. Isovolumetric Contraction** - **Structures Involved**: Ventricles (left and right), AV valves, semilunar valves (aortic and pulmonary). - **Phases Details**: - Ventricular contraction begins, increasing pressure inside the ventricles. - The AV valves close to prevent backflow into the atria, but the semilunar valves remain closed initially. - No volume change occurs as the ventricles contract with all valves closed. **3. Ventricular Ejection** - **Structures Involved**: Ventricles, semilunar valves, arteries (aorta and pulmonary artery). - **Phases Details**: - When ventricular pressure exceeds arterial pressure, the semilunar valves open. - Blood is ejected from the right ventricle into the pulmonary artery and from the left ventricle into the aorta. - This phase delivers oxygenated blood to the body and deoxygenated blood to the lungs. **4. Isovolumetric Relaxation** - **Structures Involved**: Ventricles, semilunar valves. - **Phases Details**: - Following ejection, the ventricles begin to relax. - The semilunar valves close to prevent backflow from the arteries into the ventricles. - All valves are closed, and the ventricular volume remains constant as pressure falls. **5. Ventricular Filling** - **Structures Involved**: Atria, ventricles, AV valves. - **Phases Details**: - As ventricular pressure drops below atrial pressure, the AV valves open. - Blood flows passively from the atria into the ventricles, leading to ventricular filling. - Most ventricular filling occurs during this phase, assisted by atrial contraction later on. **Importance of Heart Structures** - **Valves**: Ensure unidirectional blood flow and prevent backflow. - **Chambers**: Receive and pump blood effectively, with atria serving as primer pumps and ventricles as main pumps. - **Myocardium (Heart Muscle)**: The thick muscular wall of the ventricles facilitates strong contractions necessary for blood ejection. 2\. Describe the cardiac action potential and relate it to the conduction system of the heart and the surface electrocardiographic tracing. The cardiac action potential is a series of changes in electrical potential that occur across the cell membrane of heart muscle cells. It is crucial for the initiation and propagation of electrical impulses that trigger heart contractions, and it can be related to the heart\'s conduction system and the electrocardiogram (ECG). **Cardiac Action Potential Phases:** 1. **Phase 0 (Depolarization):** - Initiated when voltage-gated sodium (Na+) channels open. - There is a rapid influx of Na+ ions, leading to a sharp rise in membrane potential. - This phase corresponds to the QRS complex on an ECG, representing ventricular depolarization. 2. **Phase 1 (Initial Repolarization):** - Na+ channels close, and transient outward potassium (K+) currents occur. - This results in a slight decrease in membrane potential. - This phase contributes to the initial part of the ST segment on the ECG. 3. **Phase 2 (Plateau Phase):** - Characterized by the opening of voltage-gated calcium (Ca2+) channels. - There is a balance between Ca2+ influx and K+ efflux, maintaining a plateau. - This phase corresponds to the ST segment on the ECG, as it represents sustained depolarization of the ventricles. 4. **Phase 3 (Repolarization):** - Calcium channels close, and there is a continued efflux of K+. - This results in the return of the membrane potential to its resting state. - Corresponds to the T wave on an ECG, representing ventricular repolarization. 5. **Phase 4 (Resting Membrane Potential):** - The cell returns to its baseline electrical state. - Maintained by the Na+/K+ ATPase and ion exchange mechanisms. - This phase prepares the cell for the next action potential. **Conduction System of the Heart:** The conduction system ensures that the action potential is propagated effectively through the heart, leading to coordinated contraction. - **Sinoatrial (SA) Node:** - The heart\'s natural pacemaker. - Initiates the action potential that spreads through the atria. - **Atrioventricular (AV) Node:** - Receives the impulse from the atria and delays it, allowing ventricular filling. - Transmits the impulse to the ventricles through the His-Purkinje system. - **Bundle of His and Purkinje Fibers:** - Conduct the action potential to the ventricles, ensuring rapid and coordinated contraction. **Relation to Electrocardiographic Tracing:** - **P Wave:** - Represents atrial depolarization. - Initiated by the SA node and precedes atrial contraction. - **QRS Complex:** - Represents ventricular depolarization. - The rapid upstroke (Phase 0) of the action potential. - **T Wave:** - Represents ventricular repolarization. - Corresponds to Phase 3 of the action potential. - **PR Interval:** - Reflects the time taken for the electrical impulse to travel from the atria to the ventricles. - Includes the delay at the AV node. - **QT Interval:** - Represents the total time of ventricular depolarization and repolarization. This precise coordination of action potentials, conduction, and ECG tracing is essential for efficient cardiac function and can be used diagnostically to assess heart health and detect abnormalities. 3.Compare preload and afterload **\ Preload:** - **Definition**: Preload refers to the degree of stretch of the cardiac muscle fibers at the end of diastole, just before contraction. It is primarily determined by the ventricular end-diastolic volume (EDV), which is the volume of blood present in the ventricles just before systole (contraction). - **Determinants**: - **Venous Return**: The amount of blood returning to the heart from the systemic circulation. Increased venous return elevates preload. - **Circulating Blood Volume**: More circulating blood volume can increase preload. - **Venous Tone**: The constriction state of veins can impact preload by changing the volume of blood delivered to the heart. - **Atrial Contraction**: The efficiency of atrial contraction contributes to filling the ventricles, affecting preload. - **Effects**: - **Frank-Starling Law**: An increase in preload leads to increased stroke volume due to more optimal overlap of actin and myosin filaments in cardiac muscle fibers, up to a physiological limit. **Afterload:** - **Definition**: Afterload refers to the pressure the heart must work against to eject blood during systole. It is often equated with the systemic vascular resistance and the pressure in the aorta and large arteries. - **Determinants**: - **Arterial Pressure**: Higher systemic arterial blood pressure increases afterload. - **Aortic Valve Condition**: Conditions like aortic stenosis increase the resistance (afterload) faced by the heart. - **Peripheral Vascular Resistance**: Increased resistance in the blood vessels can augment afterload. - **Effects**: - **Cardiac Output**: Higher afterload can reduce stroke volume as the heart has to exert more force to open the aortic valve and eject blood. - **Ventricular Hypertrophy**: Persistent high afterload can lead to thickening of the ventricular walls as a compensatory mechanism. **Comparison:** - **Preload** is mainly about the volume of blood filling the heart and the stretch of cardiac fibers before contraction, while **afterload** is about the resistance the heart must overcome to eject blood. - **Influence on Cardiac Performance**: An increase in preload typically enhances cardiac output, whereas an increase in afterload can lead to a decrease in stroke volume if not compensated by the heart. - **Clinical Relevance**: Conditions like heart failure often involve management of preload and afterload. Drugs like diuretics and vasodilators can be used to modify preload and afterload, respectively. 4. Compare and contrast the effect of positive and negative inotropic agents on heart contractility and stroke volume. Inotropic agents are substances that affect the strength of heart muscle contraction. They can be classified into two types: positive inotropic agents and negative inotropic agents. Here's how they compare and contrast in terms of their effects on heart contractility and stroke volume: **Positive Inotropic Agents** **Effects on Heart Contractility:** - **Increase Contractility:** Positive inotropic agents enhance the force of the heart\'s contractions. They do so by increasing the availability of calcium ions in the cardiac muscle cells, which is essential for muscle contraction. - **Mechanisms:** These agents can work through various mechanisms, such as stimulating β-adrenergic receptors (e.g., adrenaline, dobutamine) or inhibiting the enzyme that breaks down cyclic AMP (e.g., phosphodiesterase inhibitors like milrinone). Some agents, like digitalis, increase intracellular calcium by inhibiting the sodium-potassium ATPase pump. **Effects on Stroke Volume:** - **Increase Stroke Volume:** By increasing the force of contraction, positive inotropic agents lead to an increase in stroke volume, the quantity of blood ejected by the heart with each beat. This is particularly beneficial in conditions like heart failure, where the heart's ability to pump effectively is compromised. **Negative Inotropic Agents** **Effects on Heart Contractility:** - **Decrease Contractility:** Negative inotropic agents reduce the contractile force of the heart muscle. They usually achieve this by reducing calcium influx into the heart cells or blocking the effects of adrenaline and other stimulants. - **Mechanisms:** Common examples include beta-blockers (like propranolol, metoprolol) and calcium channel blockers (like verapamil, diltiazem). These drugs either decrease calcium entry into cells or block the effects of sympathetic stimulation. **Effects on Stroke Volume:** - **Decrease Stroke Volume:** By reducing contractility, negative inotropic agents decrease stroke volume. This can be useful in conditions where it is desirable to decrease the workload of the heart, such as hypertension or arrhythmias. **Comparison** - **Contractility Influence:** Positive inotropic agents enhance contractility, while negative inotropic agents reduce it. - **Stroke Volume Modulation:** Positive agents increase stroke volume by facilitating greater ejection of blood per heartbeat, while negative agents decrease it, making the heart work less intensely. - **Clinical Application:** Positive inotropes are often used in acute heart failure or cardiogenic shock to support heart function. Negative inotropes are used when it\'s necessary to reduce heart workload or manage abnormal heart rhythms. 5.Describe the effects of epinephrine, antidiuretic hormone, natriuretic peptides, and renin on blood pressure. To understand how various hormones affect blood pressure, it\'s important to consider the mechanisms by which they act. Here\'s a breakdown of each hormone: 1. **Epinephrine**: - **Origin**: Epinephrine, also known as adrenaline, is produced by the adrenal glands. - **Effect on Blood Pressure**: It increases blood pressure. - **Mechanism**: - **Vasoconstriction**: Epinephrine causes the blood vessels to constrict (narrow), which increases the resistance to blood flow and thus elevates blood pressure. - **Increased Heart Rate and Contractility**: It stimulates the heart to beat faster and more forcefully, which increases cardiac output and contributes to an increase in blood pressure. - **Fight-or-Flight Response**: Typically released in response to stress, epinephrine prepares the body for a rapid response. 2. **Antidiuretic Hormone (ADH)**: - **Origin**: ADH, also known as vasopressin, is produced by the hypothalamus and released by the posterior pituitary gland. - **Effect on Blood Pressure**: It tends to increase blood pressure. - **Mechanism**: - **Water Reabsorption**: ADH increases the permeability of the kidney\'s collecting ducts, leading to more water reabsorption into the bloodstream. This increases the blood volume, thereby elevating blood pressure. - **Vasoconstriction**: At higher concentrations, ADH can cause constriction of blood vessels, further contributing to increased blood pressure. 3. **Natriuretic Peptides**: - **Types**: The most notable ones are Atrial Natriuretic Peptide (ANP) and Brain Natriuretic Peptide (BNP). - **Origin**: ANP is produced by the atria of the heart, while BNP is primarily produced by the ventricles. - **Effect on Blood Pressure**: They decrease blood pressure. - **Mechanism**: - **Vasodilation**: Both ANP and BNP promote the widening of blood vessels, which reduces vascular resistance and lowers blood pressure. - **Diuresis and Natriuresis**: They increase the excretion of sodium and water by the kidneys, which decreases blood volume and subsequently lowers blood pressure. - **Inhibition of the Renin-Angiotensin-Aldosterone System (RAAS)**: Natriuretic peptides inhibit this system, further reducing blood pressure. 4. **Renin**: - **Origin**: Renin is an enzyme released by the juxtaglomerular cells of the kidneys. - **Effect on Blood Pressure**: It indirectly increases blood pressure. - **Mechanism**: - **Conversion to Angiotensin II**: Renin initiates a cascade where angiotensinogen is converted to angiotensin I, which is further converted to angiotensin II by the angiotensin-converting enzyme (ACE). - **Functions of Angiotensin II**: - **Vasoconstriction**: It constricts blood vessels, increasing blood pressure. - **Stimulates Aldosterone Release**: Leads to more sodium and water reabsorption by the kidneys, increasing blood volume and pressure. - **Stimulates ADH Release**: Enhances water retention, contributing to increased blood pressure. 5. Define aneurysm. An aneurysm is an abnormal bulge or ballooning in the wall of a blood vessel. It occurs when the wall of the vessel becomes weakened and can no longer contain the force of blood flowing through it. Aneurysms can happen in any blood vessel but are most commonly found in the aorta (the main blood vessel leaving the heart) or in the arteries of the brain. There are several types of aneurysms: 1. **Abdominal Aortic Aneurysm (AAA):** This occurs in the part of the aorta that runs through the abdomen. It is one of the most common types of aneurysms. 2. **Thoracic Aortic Aneurysm:** This occurs in the part of the aorta located in the chest. 3. **Cerebral Aneurysm:** Often referred to as a brain aneurysm, this occurs in the blood vessels of the brain. 4. **Peripheral Aneurysms:** These can occur in other arteries away from the heart and brain, such as those in the legs, neck, or arms. Aneurysms can be life-threatening if they rupture, leading to internal bleeding and possibly death. Symptoms may not be present until an aneurysm becomes large or ruptures, but some potential signs include pain, a palpable pulsating mass, or a sudden headache (in the case of cerebral aneurysms). Diagnosis is typically made using imaging techniques like ultrasounds, CT scans, or MRIs. Treatment may involve careful monitoring, surgical repair, or endovascular procedures, depending on the size, location, and risk of rupture of the aneurysm. Thrombus formation, commonly known as blood clot formation, is a complex process regulated by several conditions and factors. This process is a normal protective mechanism of the body to prevent excessive bleeding but can become problematic when it occurs inappropriately, such as in the blood vessels, leading to thrombosis. The main conditions contributing to thrombus formation can be explained by Virchow\'s triad, which includes three primary factors: 1. **Endothelial Injury**: - Damage to the inner lining of the blood vessels, the endothelium, can initiate clot formation. This can occur due to physical injury, inflammation, or atherosclerosis (buildup of fatty deposits in arteries). Endothelial injury exposes underlying tissues which release clotting factors and attract platelets, initiating the coagulation cascade. 2. **Abnormal Blood Flow**: - Turbulent or stagnant blood flow can contribute to thrombus formation. In cases of turbulence, such as those caused by arterial bifurcations or aneurysms, the irregular flow can damage the endothelium. Stasis, where blood flow is slow or stagnant, is common in veins and allows increased contact time between platelets and the endothelium, promoting clot formation. Conditions like atrial fibrillation, prolonged bed rest, or varicose veins are examples that can cause blood flow abnormalities. 3. **Hypercoagulability**: - This refers to an increased tendency of the blood to clot, which can result from genetic or acquired conditions. Genetic factors include inherited disorders such as Factor V Leiden mutation or prothrombin gene mutation. Acquired conditions can be due to cancer, pregnancy, hormone replacement therapy, and certain medications like oral contraceptives. Additionally, systemic inflammation and some autoimmune disorders can contribute to a state of hypercoagulability. Understanding these conditions is crucial in assessing the risk factors for thrombus formation and implementing preventive strategies such as anticoagulants or lifestyle modifications, especially in high-risk populations. 8\. Compare the manifestations of ischemia and mechanisms associated with thromboangiitis obliterans (Buerger disease), Raynaud phenomenon, and Raynaud disease Thromboangiitis obliterans (Buerger disease), Raynaud phenomenon, and Raynaud disease are vascular disorders that primarily affect the blood vessels in the extremities, but they do so through different mechanisms and with different clinical manifestations. Let\'s explore each in detail: **Thromboangiitis Obliterans (Buerger Disease)** **Mechanism:** - Thromboangiitis obliterans is an inflammatory condition affecting small and medium-sized arteries and veins, predominantly in the arms and legs. - It is strongly associated with tobacco use, and the exact pathogenesis is related to an immune response triggered by smoking, leading to vasculitis and thrombosis. - The inflammation can lead to occlusion of the vessels, impairing blood flow. **Manifestations of Ischemia:** - Ischemic pain in the limbs, particularly during physical activity (claudication). - Rest pain in severe cases, especially affecting the fingers and toes. - Ulcerations and, in some cases, gangrene due to a severe reduction in blood flow. - Cool extremities with diminished pulses. **Raynaud Phenomenon** **Mechanism:** - Raynaud phenomenon is a condition often secondary to other diseases (such as scleroderma, lupus, or rheumatoid arthritis) and characterized by excessive vasoconstriction of the small arteries and arterioles. - This vasospastic reaction typically occurs in response to cold temperatures or stress. **Manifestations of Ischemia:** - Episodes of color changes in the skin of the fingers and toes (pallor, cyanosis, then erythema) due to spasms of the blood vessels. - Numbness, tingling, or pain in the affected areas during episodes. - The skin can become brittle, leading to ulcerations or sores in severe cases. **Raynaud Disease** **Mechanism:** - Raynaud disease is similar to Raynaud phenomenon but is a primary condition not secondary to another disease. - Thought to be due to hyperactivity of the sympathetic nervous system causing vasospastic attacks. **Manifestations of Ischemia:** - Similar to Raynaud phenomenon, with episodes triggered by cold or emotional stress. - Occurs without an associated systemic illness, distinguishing it from Raynaud phenomenon. **Comparison Summary** - **Thromboangiitis Obliterans** is characterized by inflammatory thrombosis leading to ischemia, strongly linked to smoking. - **Raynaud Phenomenon** is usually secondary to other diseases and involves episodic vasospastic attacks. - **Raynaud Disease** is a primary condition with similar episodes of vasospasm but without underlying systemic causes. The distinction between Raynaud phenomenon and Raynaud disease lies in the presence of an underlying condition driving the vasospastic episodes in the former, while the latter is idiopathic. All three conditions share common themes of ischemia due to reduced blood flow but differ in their etiology and approach to treatment. **Varicose Veins** **Causes:** 1. **Valve Malfunction:** Varicose veins occur when the valves in the veins become weak or damaged. These valves are responsible for preventing the backward flow of blood. When they fail to function properly, blood can pool in the veins, leading to enlargement. 2. **Genetic Predisposition:** A family history of varicose veins can increase the likelihood of developing them. 3. **Age:** As people age, veins can lose elasticity, leading to varicose veins. 4. **Prolonged Standing or Sitting:** Occupations that require long periods of standing or sitting can contribute to pressure build-up in the veins. 5. **Obesity:** Excess body weight can put extra pressure on veins, exacerbating valve problems. 6. **Hormonal Changes:** Pregnancy, menopause, and the use of hormone replacement therapy or birth control can affect the vein walls and valves. **Manifestation:** - Twisted, bulky, and discolored veins, usually on the legs - Aching or heavy feeling in the legs - Swelling, especially after standing for long periods - Itching around the veins - Skin discoloration or ulcers in severe cases **Deep Vein Thrombosis (DVT)** **Causes:** 1. **Immobility:** Extended periods of inactivity, such as long flights or bed rest, can lead to the formation of a clot. 2. **Surgery or Injury:** These can trigger the body\'s clotting mechanism as part of the healing process. 3. **Increased Blood Clotting:** Genetic conditions, certain medications, or medical conditions like cancer can increase the risk. 4. **Obesity:** Excess weight increases pressure on veins. 5. **Pregnancy:** The increased blood volume and hormonal changes can increase clot risks. 6. **Age:** Older adults are more at risk. **Manifestation:** - Swelling, usually in one leg - Pain in the leg, often described as cramping or soreness - Red or discolored skin - Warmth in the affected leg **Superior Vena Cava Syndrome** **Causes:** 1. **Tumors:** Lung cancer or lymphomas can compress the superior vena cava (SVC), obstructing blood flow. 2. **Thrombosis:** Clots in the SVC, often due to long-term use of central venous catheters. 3. **Infections:** Infections around the area of the SVC can cause swelling and compression. 4. **Fibrosis or Scarring:** Conditions leading to scarring around the vena cava may cause blockage. **Manifestation:** - Swelling of the face, neck, upper limbs, and chest - Shortness of breath or coughing - Distended veins in the upper body - Headache or visual disturbances - Cyanosis (bluish discoloration of the skin) Each of these conditions requires appropriate medical intervention to prevent complications. Varicose veins can lead to discomfort and skin changes, while DVT can result in life-threatening complications like pulmonary embolism if not treated. Superior Vena Cava Syndrome can disrupt normal circulation and requires urgent management, especially if due to malignancies. Management strategies may involve lifestyle changes, medication, lifestyle measures, or surgical interventions depending on the severity and underlying causes. Myocardial ischemia occurs when blood flow to the heart muscle is reduced, typically due to a partial or complete blockage of the heart\'s arteries. This can lead to chest pain (angina) or even a heart attack. Several treatment regimens are utilized to address myocardial ischemia, each with distinct mechanisms and effects: 1. **Nitrates:** - **Effects:** Nitrates primarily work by dilating blood vessels. This vasodilation reduces the heart\'s workload by decreasing blood pressure and venous return (preload) to the heart. By doing so, nitrates reduce the demand for oxygen by the heart muscle, which can relieve angina symptoms. - **Mechanism:** They increase levels of cyclic guanosine monophosphate (cGMP) in vascular smooth muscle, leading to relaxation and vasodilation. 2. **Beta-Adrenergic Blocking Agents (Beta Blockers):** - **Effects:** Beta blockers reduce heart rate, decrease blood pressure, and lessen the force of heart muscle contractions. These actions collectively reduce myocardial oxygen demand. - **Mechanism:** They block the effects of adrenaline (epinephrine) on the beta-adrenergic receptors in the heart, resulting in heart rate slowing and reduced contractility. 3. **Calcium Channel Blockers:** - **Effects:** These medications relax and dilate arteries, which reduces blood pressure and myocardial oxygen demand. They can also decrease the heart rate and reduce the force of contraction. - **Mechanism:** By inhibiting calcium ions from entering cardiac and arterial smooth muscle cells, they cause vasodilation and reduce the workload on the heart. 4. **Antiplatelet Agents:** - **Effects:** Antiplatelet agents help prevent the formation of new clots and reduce the growth of existing clots. This keeps blood flowing smoothly through narrowed arteries. - **Mechanism:** Common antiplatelet drugs like aspirin inhibit cyclooxygenase enzymes and reduce thromboxane production, which decreases platelet aggregation. 5. **Percutaneous Coronary Intervention (PCI):** - **Effects:** PCI, commonly known as angioplasty, is a non-surgical procedure used to open narrowed coronary arteries. By placing a stent in the artery, it improves blood flow and relief of ischemic symptoms. - **Mechanism:** A balloon is inflated at the site of narrowing to widen the artery. A stent may be placed to keep the artery open. 6. **Coronary Artery Bypass Graft (CABG):** - **Effects:** CABG is a surgical procedure used to improve blood flow to the heart. It involves taking a blood vessel from another part of the body and grafting it onto the coronary artery to bypass the blocked or narrowed portion. - **Mechanism:** This provides an alternate route for blood to reach heart tissue, effectively bypassing the blocked segment and improving oxygen supply to the heart muscle. Each of these treatments targets different aspects of myocardial ischemia, either by relieving symptoms or addressing the underlying causes of reduced blood flow. The choice of treatment depends on the severity of the ischemia, the patient\'s overall health, and other clinical considerations. **Acute Pericarditis** **Pathophysiology:** - Acute pericarditis is an inflammation of the pericardium, the double-walled sac containing the heart. - It can be caused by infections (viral, bacterial, fungal), autoimmune disorders (like lupus), myocardial infarction, trauma, or effects of radiation. - The inflammatory process leads to an infiltration of white blood cells and leakage of fibrin, red blood cells, and fluid into the pericardial space. - It commonly presents with sharp chest pain, which may be worsened by inspiration or lying flat, and relieved by sitting up and leaning forward. **Constrictive Pericarditis** **Pathophysiology:** - Constrictive pericarditis occurs when the pericardium becomes thickened, fibrotic, and sometimes calcified, losing its elasticity. - This thickened state restricts diastolic filling of the heart, causing heart failure symptoms. - It can develop following acute pericarditis, due to chronic inflammatory conditions, after cardiac surgery, or from radiation therapy. - Constrictive pericarditis leads to a characteristic ventricular interdependence and a dissociation between intrathoracic and intracardiac pressures. **Pericardial Effusion** **Pathophysiology:** - Pericardial effusion involves the accumulation of fluid in the pericardial cavity. - The fluid may accumulate due to inflammation, as seen in pericarditis, or as a result of trauma, malignancy, uremia, or hypothyroidism. - The impact on heart function depends on the volume of fluid, rate of accumulation, and the compliance of the pericardial sac. - Rapidly accumulating effusions can lead to cardiac tamponade, a life-threatening condition where the heart is compressed and unable to fill properly during diastole. **Comparison:** - **Inflammation vs. Mechanical Restriction:** Acute pericarditis primarily involves inflammation, while constrictive pericarditis and pericardial effusion involve mechanical restriction of the heart\'s function due to fibrous thickening or fluid accumulation, respectively. - **Time Course:** Acute pericarditis is generally a more sudden onset condition, while constrictive pericarditis is more chronic, developing over months or years. Pericardial effusions can be acute or chronic depending on the underlying cause. - **Symptoms and Signs:** Acute pericarditis is noted for chest pain and possibly a pericardial friction rub on auscultation. Constrictive pericarditis often presents with symptoms of right-sided heart failure and Kussmaul\'s sign (a paradoxical rise in venous jugular pressure during inspiration). Pericardial effusions may present with muffled heart sounds and hypotension, especially in tamponade. **Contrast:** - **Underlying Causes:** The causes of these conditions can overlap (e.g., infections, trauma), but the clinical consequences differ significantly. For example, a viral infection might cause acute pericarditis, but chronic autoimmune activity might lead to constrictive pericarditis. - **Treatment Approaches:** The management strategies differ: acute pericarditis might be treated with NSAIDs and colchicine, constrictive pericarditis might require surgical intervention (pericardiectomy), and large or symptomatic pericardial effusions might require pericardiocentesis or surgical drainage. In summary, while all three conditions affect the pericardium, they differ primarily in their pathophysiological processes, clinical presentations, and management strategies. Understanding these differences is crucial for accurate diagnosis and treatment. Rheumatic heart disease and infective endocarditis are both conditions that affect the heart and its function, but their pathophysiologic mechanisms differ substantially. Here\'s a comparison of the two: **Rheumatic Heart Disease** **Cause**: Rheumatic heart disease is a chronic condition that results from rheumatic fever, which in turn is a complication of untreated or inadequately treated streptococcal throat infection. The responsible organisms are Group A Streptococcus (Streptococcus pyogenes). **Pathophysiologic Mechanism**: 1. **Autoimmune Reaction**: The molecular mimicry between the antigens of the bacteria and heart tissue leads to an autoimmune response. Antibodies and T-cells attack the heart tissues, mistaking them for the streptococcal antigens. 2. **Inflammation**: This autoimmune reaction primarily affects the heart valves, causing inflammation characterized by Aschoff bodies. The mitral valve is most commonly affected, followed by the aortic valve. 3. **Scarring and Fibrosis**: Chronic inflammation results in scarring and fibrosis of the heart valves, leading to stenosis or regurgitation. 4. **Valvular Damage**: Over time, this damage can lead to serious consequences such as heart failure, atrial fibrillation, and increased risk of stroke. **Infective Endocarditis** **Cause**: Infective endocarditis is an infection of the endocardium, usually involving the heart valves. It is typically caused by bacteria, such as Streptococcus viridans, Staphylococcus aureus, and Enterococcus species, but can also be caused by fungi and other microorganisms. **Pathophysiologic Mechanism**: 1. **Bacteremia**: The condition begins when bacteria or other pathogens enter the bloodstream through oral, gastrointestinal, urinary, or skin routes. Dental procedures, surgeries, or intravenous drug use often contribute to this entry. 2. **Adherence to Valves**: The pathogens adhere to damaged or native heart valves, creating vegetations. Conditions like rheumatic heart disease or congenital heart defects predispose individuals to such adhesion by exposing the endocardium. 3. **Vegetation Formation**: The bacteria proliferate within the vegetations, which consist of a mass of platelets, fibrin, microorganisms, and inflammatory cells. This can lead to further damage to the valve structures. 4. **Embolism and Immune Complex Deposition**: Bits of the vegetation can break off, leading to embolic events in various organs. Additionally, the continuous release of bacterial antigens into circulation results in immune complex deposition in tissues, potentially leading to conditions such as glomerulonephritis. 5. **Valvular Dysfunction**: The destruction caused by vegetations can lead to acute valvular insufficiency and heart failure if not treated promptly. **Conclusion** While both rheumatic heart disease and infective endocarditis impact heart valves and can lead to complications such as heart failure, their underlying mechanisms are quite different. Rheumatic heart disease is largely autoimmune following a bacterial infection, while infective endocarditis is a direct bacterial infection of the cardiac tissue. Each condition requires different preventive strategies and treatment approaches tailored to the root cause of the pathology. Heart failure can occur on the right side (known as right-sided heart failure or cor pulmonale) or the left side (known as left-sided heart failure or congestive heart failure). Both types have distinct pathophysiologies, manifestations, and treatment approaches. Let\'s explore these aspects for each type: **Right-Sided Heart Failure (Cor Pulmonale)** **Pathophysiology:** - Right-sided heart failure is primarily caused by pulmonary hypertension, which increases pressure in the right ventricle, leading to its eventual failure. It often results from chronic lung diseases (e.g., chronic obstructive pulmonary disease, pulmonary embolism, interstitial lung disease) that affect the pulmonary vasculature. - As the right ventricle struggles to pump blood into the high-pressure pulmonary arteries, it undergoes hypertrophy initially and eventually dilates, leading to systolic dysfunction. **Manifestations:** - Peripheral edema: Swelling in the legs and ankles due to fluid retention. - Ascites: Accumulation of fluid in the abdominal cavity. - Hepatomegaly and splenomegaly: Enlargement of the liver and spleen due to congestion. - Jugular venous distension: Visible bulging of the neck veins. - Fatigue and weakness: Due to inadequate blood flow to systemic circulation. **Treatment:** - Management of the underlying pulmonary condition to reduce pulmonary hypertension. - Diuretics to manage fluid overload and reduce edema. - Vasodilators to decrease pulmonary vascular resistance. - Oxygen therapy to improve oxygen delivery and reduce hypoxic vasoconstriction in the lungs. - In some cases, anticoagulation may be prescribed if there is a risk of pulmonary embolism or other thromboembolic events. **Left-Sided Heart Failure (Congestive Heart Failure)** **Pathophysiology:** - Left-sided heart failure occurs when the left ventricle cannot effectively pump blood into systemic circulation, leading to a backlog of blood in the lungs. - It can be categorized into systolic heart failure (due to reduced ejection fraction from weakened heart muscle) or diastolic heart failure (preserved ejection fraction but with impaired ventricular filling due to stiffened heart muscle). - Common causes include coronary artery disease, hypertension, myocardial infarction, and cardiomyopathy. **Manifestations:** - Pulmonary congestion: Leading to symptoms such as dyspnea (shortness of breath), orthopnea (difficulty breathing while lying down), and paroxysmal nocturnal dyspnea (sudden breathlessness at night). - Cough with frothy sputum: Due to pulmonary edema. - Fatigue and decreased exercise tolerance: Due to reduced cardiac output. - Crackles heard on lung auscultation: Due to fluid in the alveolar spaces. - Tachycardia: As the heart tries to compensate for reduced pumping efficiency. **Treatment:** - Diuretics to reduce fluid overload and relieve pulmonary congestion. - ACE inhibitors or ARBs to reduce afterload and prevent ventricular remodeling. - Beta-blockers to reduce heart rate, improve heart function, and decrease mortality. - Aldosterone antagonists for further diuresis and neurohormonal blockade. - In severe cases, devices like implantable cardioverter-defibrillators (ICDs) or ventricular assist devices (VADs) may be used, or heart transplantation may be considered. By understanding the differences in pathophysiology, manifestations, and treatment strategies, healthcare providers can tailor therapies to optimize outcomes for patients with heart failure. 1. Discuss the source and physiologic function of surfactant. Surfactant is a complex substance primarily composed of phospholipids and proteins that plays a crucial role in the respiratory system, specifically in the alveoli of the lungs. **Source of Surfactant** Surfactant is produced by type II alveolar cells (also known as type II pneumocytes) in the lungs. These cells are located in the alveolar walls and are responsible for synthesizing and secreting the surfactant into the alveolar space. **Composition** The primary component of surfactant is a phospholipid called dipalmitoylphosphatidylcholine (DPPC). It also contains other phospholipids, neutral lipids, and surfactant proteins, which are critical for its function and regulation. There are four main surfactant proteins: SP-A, SP-B, SP-C, and SP-D, each serving distinct roles in surfactant metabolism and immune functions. **Physiologic Function of Surfactant** 1. **Reduction of Surface Tension**: The primary function of surfactant is to reduce the surface tension within the alveoli. The alveoli are tiny air sacs in the lungs where gas exchange occurs. The inner surface of these sacs is lined with a thin layer of fluid, which creates surface tension due to the cohesive forces between water molecules. This surface tension would normally make the alveoli prone to collapse, especially during expiration when the alveoli are smaller. Surfactant reduces this surface tension, helping to keep the alveoli open and stable. 2. **Enhancement of Lung Compliance**: By reducing surface tension, surfactant also increases lung compliance, which is the ability of the lungs to expand. This makes breathing easier by reducing the work required to inflate the alveoli during inspiration. 3. **Prevention of Alveolar Collapse (Atelectasis)**: During expiration, without surfactant, the surface tension would pull the alveolar walls inward, leading to collapse. Surfactant prevents this atelectasis, ensuring that alveoli remain partially open at the end of expiration, which aids in efficient gas exchange. 4. **Host Defense**: Surfactant proteins, particularly SP-A and SP-D, play roles in the lung\'s immune defense by binding to pathogens and enhancing their clearance by immune cells. 5. **Fluid Balance**: Surfactant aids in maintaining the balance between fluids in the alveolar spaces and the interstitium, preventing pulmonary edema. **Clinical Relevance** Deficiencies in surfactant production or function can lead to respiratory distress syndromes. In newborns, especially premature infants, lack of surfactant can cause neonatal respiratory distress syndrome (NRDS), a serious condition that requires medical intervention often involving surfactant replacement therapy. In adults, acute respiratory distress syndrome (ARDS) may involve surfactant dysfunction as wellIn summary, surfactant is vital for normal respiratory function, preventing alveolar collapse, enhancing lung compliance, and contributing to the innate immune defense of the lungs. 2. Describe the movement and transport forms of oxygen in the blood. Oxygen transport in the blood is a vital physiological process critical for sustaining life, and it involves two main forms: dissolved oxygen and oxygen bound to hemoglobin. **1. Oxygen Dissolved in Plasma:** - **Solubility**: Oxygen is only slightly soluble in water. Therefore, a small amount of oxygen, roughly 1.5% of the total oxygen, is transported dissolved in the plasma (the liquid component of blood). While this form of transport is not the most efficient due to poor solubility, it is important as it contributes to the oxygen tension in the blood. **2. Oxygen Bound to Hemoglobin:** - **Role of Hemoglobin**: The majority of oxygen in the blood is transported bound to hemoglobin, a protein found in red blood cells. Approximately 98.5% of oxygen is carried this way. Hemoglobin is a complex protein with four subunits, each containing an iron ion that can bind one molecule of oxygen, allowing each hemoglobin molecule to transport up to four oxygen molecules. - **Oxyhemoglobin Formation**: When oxygen binds to hemoglobin, it forms oxyhemoglobin. This reaction is reversible and is influenced by the oxygen concentration, depicted as partial pressure of oxygen ((P\_{O2})). **Steps of Oxygen Transport:** 1. **Oxygen Uptake in the Lungs**: - In the pulmonary capillaries, oxygen from inhaled air diffuses across the alveolar membrane into the blood, driven by a gradient from high partial pressure in the alveoli to lower partial pressure in the blood. - Oxygen then dissolves in the plasma and binds to hemoglobin, forming oxyhemoglobin. 2. **Transport through the Circulatory System**: - Oxygen-rich blood is pumped from the lungs to tissues via the circulatory system. - As blood travels through systemic circulation, it delivers oxygen to various tissues. 3. **Oxygen Release in Tissues**: - At the tissue level, cells consume oxygen during metabolism, lowering the local (P\_{O2}). - This lower (P\_{O2}) favours the release of oxygen from oxyhemoglobin (as hemoglobin\'s affinity for oxygen decreases), which then diffuses into cells to be used in cellular respiration. **Factors Influencing Oxygen Binding and Release:** - **Bohr Effect**: The presence of high levels of carbon dioxide and hydrogen ions (low pH) in tissues reduces hemoglobin\'s affinity for oxygen, promoting oxygen release. - **Temperature and 2,3-Bisphosphoglycerate (BPG)**: Increased temperature and the presence of BPG (a metabolite that binds to hemoglobin) also lower oxygen affinity, enhancing oxygen delivery to tissues. This complex and finely tuned system ensures that oxygen is efficiently picked up in the lungs and delivered where it is needed in the body, allowing cells to perform essential metabolic functions.