Enzymes (1) PDF
Document Details
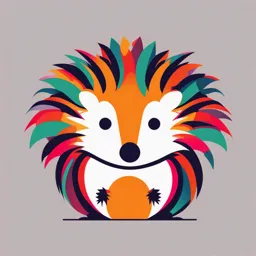
Uploaded by BrainyClarity
Lali Shanshiashvili
Tags
Summary
This document provides information about different types of enzymes and their corresponding functions. It covers various enzyme classes (oxidoreductases, transferases, hydrolases). The document also details the concept of enzyme activity and its regulation.
Full Transcript
Prof. Lali Shanshiashvili The six classes of enzimes Most of the classical metabolic enzymes are named by adding the suffix -ase to the name of their substrates or to a descriptive term for the reactions they catalyze. For example: Urease has urea as a substrate. Alcohol dehydrogen...
Prof. Lali Shanshiashvili The six classes of enzimes Most of the classical metabolic enzymes are named by adding the suffix -ase to the name of their substrates or to a descriptive term for the reactions they catalyze. For example: Urease has urea as a substrate. Alcohol dehydrogenase catalyzes the removal of hydrogen from alcohols (i.e., the oxidation of alcohols). A committee of the International Union of Biochemistry and Molecular Biology (IUBMB) maintains a classification scheme that categorizes enzymes according to the general class of organic chemical reaction that is catalyzed. The IUBMB classification scheme assigns a unique number, called the enzyme classification number, or EC number, to each enzyme. 1. Oxidoreductases Oxidoreductases catalyze oxidation–reduction reactions. Most of these enzymes are commonly referred to as dehydrogenases. Other enzymes in this class are called oxidases, peroxidases, oxygenases, or reductases. One example of an oxidoreductase is lactate dehydrogenase (EC 1.1.1.27) also called lactate:NAD oxidoreductase. This enzyme catalyzes the reversible conversion of L-lactate to pyruvate. 2. Transferases Transferases catalyze group transfer reactions and many require the presence of coenzymes. This group includes kinases, enzymes that catalyze the transfer of a phosphoryl group from ATP. Alanine transaminase, whose systematic name is L-alanine:2-oxyglutarate aminotransferase (EC 2.6.1.2), is a typical transferase. It transfers an amino group from L-alanine to a- ketoglutarate (2-oxoglutarate). 3. Hydrolases Hydrolases catalyze hydrolysis. Pyrophosphatase is a simple example of a hydrolase. The systematic name of this enzyme is diphosphate phosphohydrolase (EC 3.6.1.1). 4. Lyases Lyases catalyze lysis of a substrate generating a double bond in nonhydrolytic, nonoxidative, elimination reactions. In the reverse direction, lyases catalyze the addition of one substrate to the double bond of a second substrate. Pyruvate decarboxylase belongs to this class of enzymes since it splits pyruvate into acetaldehyde and carbon dioxide. The systematic name for pyruvate decarboxylase, 2-oxo-acid carboxy-lyase (EC 4.1.1.1), is rarely used. 5. Isomerases Isomerases catalyze structural change within a single molecule (isomerization reactions). Because these reactions have only one substrate and one product, they are among the simplest enzymatic reactions. Alanine racemase (EC 5.1.1.1) is an isomerase that catalyzes the interconversion of L-alanine and D- alanine. The common name is the same as the systematic name. 6. Ligases Ligases catalyze ligation, or joining, of two substrates. These reactions require the input of chemical potential energy in the form of a nucleoside triphosphate such as ATP. Ligases are usually referred to as synthetases. Glutamine synthetase, or L-glutamate: ammonia ligase (ADP-forming) (EC 6.3.1.2), uses the energy of ATP hydrolysis to join glutamate and ammonia to produce glutamine. Distribution of all known enzymes by EC classification number: 1. Oxidoreductases; 2. Transferases; 3. Hydrolases; 4. Lyases; 5. Isomerases; 6. Ligases. PROPERTIES OF ENZYMES Enzymes are protein catalysts that increase the velocity of a chemical reaction, and are not consumed during the reaction. Models of enzyme-substrate complex formation Lock-and-kay model Emil Fischer (1852 – 1919) Daniel Koshland (1920 –2007) Induced-Fit model D. Holoenzymes Some enzymes require molecules other than proteins for enzymic activity. The term holoenzyme refers to the active enzyme with its nonprotein component, whereas the enzyme without its nonprotein moiety is termed an apoenzyme and is inactive. If the nonprotein moiety is a metal ion such as Zn2+ or Fe2+, it is called a cofactor. If it is a small organic molecule, it is termed a coenzyme. A. Active sites The active site is not a passive receptacle for binding the substrate, but rather is a complex molecular machine employing a diversity of chemical mechanisms to facilitate the conversion of substrate to product. The substrate-binding pockets of trypsin, chimotripsin and elastase. Composition of the amino acid sequences of trypsin, chimotripsin and elastase B. Catalytic efficiency Enzyme-catalyzed reactions are highly efficient, proceeding from 10 3 –10 8 times faster than uncatalyzed reactions. The number of molecules of substrate converted to product per enzyme molecule per second is called the turnover number, or k and typically is 10 2 –10 4 s. C. Specificity Enzymes are highly specific, interacting with one or a few substrates and catalyzing only one type of chemical reaction. E. Regulation Enzyme activity can be regulated, that is, increased or decreased, so that the rate of product formation responds to cellular need. Several enzymes are produced and stored as inactive precursors called proenzymes or zymogens. Zymogens are converted into active enzymes by the irreversible cleavage of one or more peptide bonds. Chimotripsinogen is produced in the pancreas. After chymotripsinogen is secreted into the small intestine, it is converted to its active form that is responcible for digesting dietary protein in several steps. Schematic representation of types of multienzyme systems carrying out a metabolic pathway: A. Energy changes occurring during the reaction Virtually all chemical reactions have an energy barrier separating the reactants and the products. This barrier, called the free energy of activation, is the energy difference between that of the reactants and a high-energy intermediate that occurs during the formation of product. Figure shows the changes in energy during the conversion of a molecule of reactant A to product B as it proceeds through the transition state (high-energy intermediate),T* FACTORS AFFECTING REACTION VELOCITY Enzymes can be isolated from cells, and their properties studied in a test tube (that is, in vitro). Different enzymes show different responses to changes in substrate concentration, temperature, and pH. Enzymic responses to these factors give us valuable clues as to how Enzymes function in living cells (that is, in vivo). A. Substrate concentration Maximal velocity: The rate or velocity of a reaction (v) is the number of substrate molecules converted to product per unit time; Velocity is usually expressed as μmol of product formed per minute. The rate of an enzyme-catalyzed reaction increases with substrate concentration until a maximal velocity (Vmax) is reached. The leveling off of the reaction rate at high substrate concentrations reflects the saturation with substrate of all available binding sites on the enzyme molecules present. Increase of velocity with B. Temperature temperature: The reaction velocity increases with temperature until a peak velocity is reached. This increase is the result of the increased number of molecules having sufficient energy to pass over the energy barrier and form the products of the reaction. The optimum temperature for most human enzymes is between 35°C and 40°C. Human enzymes start to denature at temperatures above 40°C. The thermophilic bacteria found in the hot springs have optimum temperatures of 70°C. C. pH 1. Effect of pH on the ionization of the active site: The concentration of H+ affects reaction velocity in several ways. First, the catalytic process usually requires that the enzyme and substrate have specific chemical groups in either an ionized or unIonized state in order to interact. For example, catalytic activity may require that an amino group of the enzyme be in the protonated form (–NH3) +. At alkaline pH, this group is deprotonated, and the rate of the reaction, therefore, declines. 2. Effect of pH on enzyme denaturation: Extremes of pH can also lead to denaturation of the enzyme, because the structure of the catalytically active protein molecule depends on the ionic Character of the amino acid side chains. 3. The pH optimum varies for different enzymes: The pH at which maximal enzyme activity is achieved is different for different enzymes and often reflects the [H]+ at which the enzyme functions in the body. For example, pepsin, a digestive enzyme in the stomach, is maximally active at pH 2, whereas other enzymes, designed to work at neutral pH, are denatured by such an acidic environment.